5 The brain–behaviour relationship
an introduction
Anatomical organisation of the brain: a brief revision
Information highways: neural connectivity within the brain
Functional organisation of the cortex
Clinical consequences of plasticity in the central nervous system
Summary and overview of plasticity
The brain and behaviour: an overview
At the end of this chapter, you should be able to:
• describe main afferent and efferent pathways and key functions of the main anatomical regions of the central nervous system in terms of behaviour, cognition and emotion.
• explain the main effects of lesions to these regions.
• compare and contrast three methods for mapping the relationship between the brain and behaviour:
• identify key principles of functional organisation of the cortex and explain the processes mediating neuroplasticity
• provide a coherent summary of the relationship between the brain and behaviour.
Introduction
As described in Chapter 1, it is now widely accepted that the brain is the centre for behaviour, thinking and emotion, but how does it govern these functions? What is the relationship between the brain and behaviour? Can knowledge about the site of a brain lesion help to explain difficulties with behaviour, thinking and emotion? Is the relationship between the brain and behaviour fixed, or does it change? Does therapeutic input, aimed at improving behavioural outcomes, have any influence on the brain?
These and other interesting questions will be explored in this chapter. We will build on Chapter 2, which introduced the main anatomical subdivisions of the brain. In particular we will expand on the cerebral cortex and explore how various regions within the cortex are involved in behaviour, cognition and emotion.
Anatomical organisation of the brain: a brief revision
You will remember the main anatomical subdivisions of the brain, which were presented in Chapter 2. Table 5.1 expands this table by adding a column with the main functional roles of each area of the brain. In this chapter, we will focus primarily on the role of the cerebral cortex in behaviour (Fig. 5.1). During the process of evolution, this part of the brain in particular has undergone a considerable expansion, which is thought to be related to skills and abilities that make humans stand out in the animal kingdom, i.e. the ability to use sophisticated language, reasoning, planning and problem solving, judgement, and…humour.
Information highways: neural connectivity within the brain
Association fibres are neurones that connect different areas within each hemisphere (Fig. 5.2a). For example, the parietal lobe receives visual and acoustic information from the occipital and temporal lobes respectively. Association fibres serve to combine the information from those areas with somatosensory information from within the parietal lobe itself. This confluence of information enables different sources of sensory information to be associated and integrated into a coherent percept about the body in space. This information is then relayed to the frontal lobe, which is responsible for preparing and executing action. In turn, any action planned by the frontal lobe is relayed back to the parietal lobe to enable it to interpret sensory feedback correctly, as the action unfolds. This reciprocal communication, evidenced by extensive white matter connections within each hemisphere, is found throughout the brain.
Commissural fibres provide connections between the two hemispheres by the corpus callosum (Fig. 5.2b). It has been estimated that there are around 200 million of such fibres, which illustrates the importance of integration of information between the left and right sides of the brain. For example, consider the ease with which you open a jar; one hand grasps the jar while the other manipulates the lid. Usually, the action is fluid and effective. Now try and undertake this action with another person; one holds the jar while the other opens the lid. Why was that so difficult? In the latter situation, the left hand did not know what the right hand was doing! The ease with which we normally undertake bimanual activities can be attributed to the extensive communication between the two hemispheres, in this case with respect to the position and forces applied to the object by each hand. In rare cases, e.g. of intractable epilepsy, the connectivity between the two hemispheres has been surgically disrupted to prevent electrical abnormality spreading from one hemisphere to the other. This results in the so-called ‘split brain’ syndrome, which gives rise to peculiar symptoms, as each hemisphere is independent and restricted to perceiving information from, and controlling action of, the contralateral side only. More about this syndrome can be read in Kolb and Whishaw (2008).
Projection fibres provide connectivity between higher and lower areas by formeing connections between end points of a neurone (Fig. 5.2c). For example, there are projection fibres from the primary motor cortex to the anterior horn in the spinal cord, or from the eye to the primary visual area in the occipital lobe.
Functional organisation of the cortex
Functional maps
• By relating specific deficits to known brain lesions. A good example of this is Paul Broca’s identification of the speech production area in a patient with a localised lesion of the left frontal lobe (see Chapter 11 for more detail). The case study of H.M. has resulted in recognition of the role of the medial temporal lobe in memory (Chapter 9), while the case study of Phineas Gage placed the frontal lobe on the map in terms of its role in executive function (Chapter 12). As you can see, maps of the brain–behaviour relationship of this kind are primarily based on case studies. However, illuminating and fascinating these may be, it is important to consider the possible problems with this approach. For example, H.M.’s medial temporal lobes were removed because of severe epilepsy. How confident can we be that H.M.’s brain was otherwise normal, and that findings based on this case study can, therefore, be generalised to a normal population?
• Functional maps can also be constructed by noting behaviour(s) elicited during electrical stimulation of the cortex. A well-known contributor to this body of research was Wilder Penfield (1891–1976), a pioneer in neurosurgery in the treatment of epilepsy, who was introduced in Chapter 1. Surgical interventions for epilepsy are usually only undertaken if pharmacological interventions are ineffective, and/or where side effects are prohibitive. Surgical interventions aim to disconnect the source of epilepsy, thereby preventing the abnormal electrical activity from spreading. However, it is crucial of course that areas with normal function are preserved. The surgical procedure requires patients to be alert and interacting with the surgeon. The surgeon electrically stimulates different areas of the cortex and observes the patient’s responses. These may range from specific movements, to sensations, to the experience of emotions or memories. By noting these observations and collating the responses from numerous patients, Wilder Penfield was able to draw up what is now known as the ‘homunculus’ (Latin for ‘little man’), of which there is a motor and a sensory variation, each identifying the brain areas involved in movement and sensation, respectively (see Fig. 5.3).
• Finally, functional maps of the brain–behaviour relationship can be devised by recording activity of the cortex during a specific activity. With the advent of neuroimaging technology (Chapter 2), this area of research has seen a virtual explosion. Groundbreaking research into the neural correlates of chronic depression was published by Drevets et al. (1992), using PET scans to identify areas of abnormal activity, compared to controls. This work identified, among other areas, the left frontal cortex and the amygdala to be abnormally active. Another example is the discovery of the mirror neurone system in monkeys, using single-cell recordings, which demonstrated the involvement of the frontal lobe in observational learning – the so-called mirror neurone system (Chapter 8). Since the original discovery, the mirror neurone system has also been found in humans and theories on its potential role in empathy have been expanded.
Cytoarchitectonic maps
These maps are based on architectural properties of brain cells, such as size, shape and arrangement (Fig. 5.4). Brodmann, who pioneered this approach, used different stains to identify different types of cells. Although continually being updated and refined, the Brodmann map is still useful as the main areas correspond with functions, identified through functional or projection techniques. Brodmann’s areas and their key functions that allied health professionals (AHPs) should be familiar with are listed in Table 5.2.
Table 5.2 Brodmann’s areas relevant for allied health professionals and their main functions
Brodmann’s area | Name | Main function |
---|---|---|
1, 2, 3 | Primary sensory cortex | Somatosensory function |
4 | Primary motor cortex | Movement execution |
6 | Pre-motor cortex | Movement planning |
8 | Frontal eye field | Eye movement |
17 | Primary visual cortex (V1) | Vision |
22 | Wernicke’s area | Speech and language: comprehension |
41 | Primary auditory cortex | Audition |
44 | Broca’s area | Speech and language: expression |
Current understanding of the brain–behaviour relationship
Synthesising the information emerging from the brain–behaviour maps, what is our current understanding of the brain–behaviour relationship? A pioneer in this area was Aleksandr Romanovich Luria (1902–1977) who laid the foundation for neuropsychology, through numerous scientific as well as case study publications. Luria was a Russian neuropsychologist and much of his work is based on his experiences with war victims, affected by bullet wounds to the head. Luria’s talent was to integrate his expertise as a neuropsychologist with the experiences of his patients in his writings, crafting a unique insight into the impact of certain brain lesions on an individual’s life. A recommended read is The Man with a Shattered World (Luria 1987), which brings to life the impact of brain injury on a person’s behaviour, thinking and emotion, through years of naturalistic observation and conversation between Luria and Zasetsky, his patient.
Although the details of Luria’s model have been updated with emerging experimental findings, his global interpretation is still of value. Luria proposed that the brain was broadly divided into two units, i.e. the motor unit (all brain area ventral of the central sulcus) and the sensory unit (all brain areas rostral to the central sulcus) (Fig. 5.5). Let’s use the example of stopping before a red traffic light. The information process would commence with visual information being relayed from the eyes to the primary sensory area (i.e. the first port of call for raw visual sensory information such as light, colour or shape), where this information is integrated into the percept of ‘traffic light’. The secondary area would elaborate on this information, by taking in the traffic scene as a whole, while in the tertiary area, this information is further integrated with memories and emotions of past experiences, prompting with a sense of urgency the recollection that this signal implies a ‘stop’. Next, this information is relayed from the sensory unit to the action unit, where the appropriate action is prepared. In the tertiary area of this unit, different plans may be considered (e.g. to speed up/stop) from which one is selected, based on the visual information, memory and emotion. The plan is further prepared in the secondary area, which is responsible for planning the action. The actual initiation of the action is the responsibility of the primary area, which issues motor commands through the dedicated motor pathways – resulting in the final action of operating the brake. Luria’s model – the first comprehensive model explaining the relationship between the brain and behaviour – is now considered to be simplistic, however the legacy of this pioneering work is evident in that some of his original terminology (e.g. primary and secondary (or association) areas) are still in use today:
• Primary sensory areas are brain areas that receive raw sensory information, which is processed in secondary – or association areas into meaningful percepts (see Chapter 10).
• Primary motor areas are cortical areas involved in issuing motor commands, which are planned in secondary – or association areas (Chapter 7).
• The organisation of the brain is hierarchical.
• Information is processed in a parallel-distributed manner:
To explain the above in more detail: the brain operates in a hierarchical manner, which means that some areas are essential for particular functions. An example is Brodmann’s area 17 (known as V1 or primary visual area), where all visual sensory information converges. If a person has a lesion that has affected this entire area, then the person will experience what is known as ‘cortical blindness’. This means that, although the visual system proper (i.e. eyes and optic nerves) is intact, the person acts as if they are blind as the processing of visual information is impaired. Cortical blindness is an interesting phenomenon, for which the interested reader is referred to Kolb and Whishaw (2008).
A key phenomenon of brain function is plasticity, also known as neuroplasticity.
Neuroplasticity
Neuroplasticity1 is the capacity of the nervous system to adapt itself to a change in input. For example, learning a new language, a new musical instrument, or learning to walk with crutches. There are several aspects to neuroplasticity: anatomical, physiological and pharmacological. This section will discuss neuroplastic responses to injury and compare processes in the peripheral nervous system with those in the central nervous system.
How modifiable are the connections made during development?
Anatomical change
Redundancy refers to the ability of the brain to recruit intact, surplus substrate in order to recover function. Work on primates by Nudo et al. (2000) has shown that specific portions of the motor map (e.g. of the hand) contain connections with a considerable amount of overlap. This implies that if some connections have been damaged, others can be recruited to support the original function (Kleim and Schwerin 2010).
Initially it was thought that cortical representations (e.g. the motor and sensory homunculus) were static once adulthood had been reached. However, more recent studies have shown that these representations are dynamic; their size changes depending on how much the area is being used. Studies on healthy primates (Nudo et al. 2000
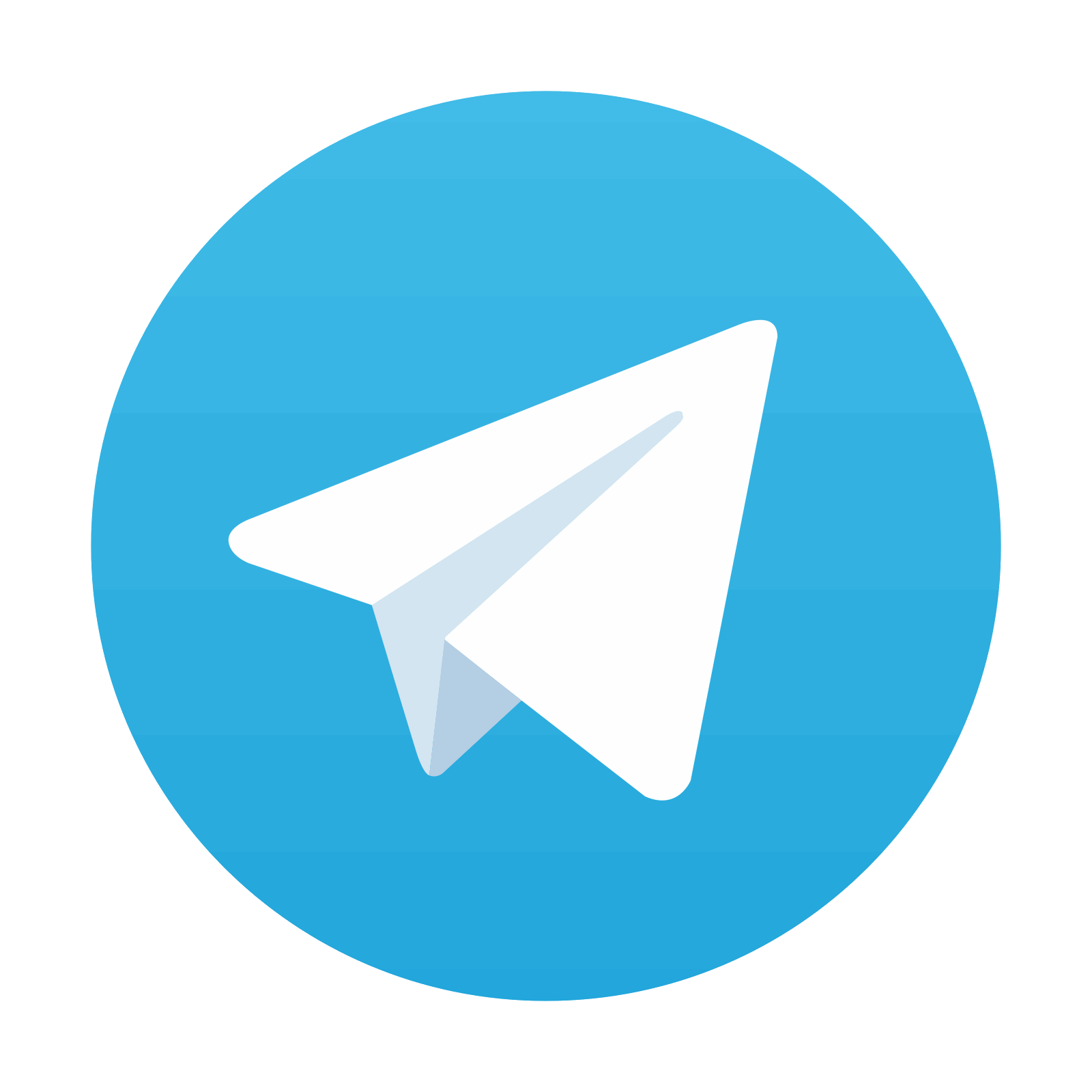
Stay updated, free articles. Join our Telegram channel

Full access? Get Clinical Tree
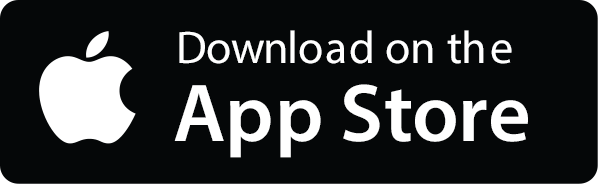
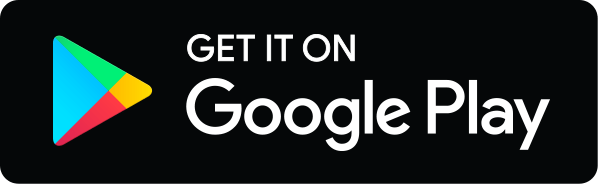