The Central Nervous System of Invertebrates
Volker Hartenstein
8.1 Organizing Principles of Nervous System Architecture
8.1.1 Evolutionary Origin of the Nervous System
All animal taxa except porifera and placozoa have nervous systems consisting of neurons specialized for the repeated conduction of an excited state from receptor sites or other neurons to effectors or other neurons (Bullock & Horridge, 1965). Structural hallmarks of a neuron are the elongated neuronal processes (neurites) projecting from the cell body (soma) and the specialized membrane domains, called synapses, by which the electric activity of one neuron is transmitted to another. Molecularly, neurons are equipped with a multitude of specialized proteins allowing for signal conduction and transmission, including ion pumps and ion channels, transmitter receptors and transporters, and many others.
It was traditionally thought (reviewed in Moroz, 2014) that neurons are monophyletic in origin—that after the “primordial neuron” appeared on the scene, it evolved into all neurons found in present day animal taxa. However, based on current molecular as well as comparative‐morphological data, it is equally likely that neurons derived multiple times independently. It is important to note in this context that the molecular building blocks of neurons, such as gated ion channels, neurotransmitters, vesicular transporters and many others, predate the appearance of neurons, and can be found in protists, placozoa, and sponges (Achim & Arendt, 2014; Alié & Manuel, 2010; Burckhardt, 2015; Cai, 2012; Jékely, 2011; Leys & Degnan, 2001; Liebeskind, Hillis, & Zakon, 2011; Renard et al., 2009; Sakarya et al., 2007). Cells with ultrastructural building blocks of neurons, such as specialized motile or sensory cilia or vesicles releasing signaling molecules, are also common in such animals. All these cells had to do in order to evolve into what would be considered a neuron by the above definition would have been to “invent” a neurite, by rearranging certain components of their cytoskeleton. This event could easily have happened multiple times during early animal evolution (Moroz, 2009).
In the simplest case, found in many extant cnidarian and ctenophore taxa, the nervous system constitutes a nerve net (Bullock & Horridge, 1965; Eichinger & Satterlie, 2014; Grimmelikhuijzen & Westfall, 1995; Mackie, 2004; Sakaguchi, Mizusina, & Kobayakawa, 1996; Satterlie & Eichinger, 2014; Watanabe, Fujisawa, Holstein, 2009). Cnidarians are built of two epithelial tissue layers, an outer epidermis (skin) and an inner gastrodermis (gut). There is no separate muscle layer; the epidermal cells contain contractile fibrils responsible for the movement of the body. Sandwiched in between the two tissue layers is a network of neurons, called ganglion cells, that are connected to each other and to the myoepithelial epidermal cells by synapses (see Figure 8.1). Ganglion cells may have two neurites (bipolar neurons), or multiple neurites (multipolar neurons). Synapses at the junction between two ganglion cells typically conduct signals in both directions (non‐polarized synapses). However, polarized synapses, which transmit signals in only one direction and which form the prevalent type of synaptic contact in most animals, also exist already in cnidaria (Westfall, Yamataka, & Enos, 1971).

Figure 8.1 Nerve Net in Cnidarians.
(A) Line drawing of polyp, showing multipolar neurons (after). (B) Schematic cross‐section of bodywall of cnidarian, showing outer layer (epidermis), inner layer (gastrodermis), sensory neurons, and ganglion cells. (C) Hertwig’s hypothesis of evolution of nerve cells.
Hanstroem, 1968. Reproduced with permission of Springer.
(D–F) Labeling of nerve net of Hydra viridissima with antibody CC04.
Sakaguchi 1996. Reproduced with permission of John Wiley and Sons.
In addition to the ganglion cells, the epidermis and gastrodermis of cnidaria contain sensory neurons that are able to sense various mechanical and chemical stimuli. Sensory neurons are specialized epithelial cells with a modified apical cilium, which serves as the stimulus‐receiving apparatus, and a basal neurite that feeds into the nerve net. Long neurites conducting signals away from the soma are referred to as axons; neurites, such as the modified cilium of a sensory neuron, that receive a signal and transmit it to the soma (or, in many cases, directly to the axon) are called dendrites.
Ctenophora (comb jellyfish), also possess a nerve net with sensory neurons and ganglion cells (Bullock & Horridge, 1965; Jager et al., 2011), and were previously united with Cnidaria in the superphylum “Coelenterata.” However, several recent studies suggest strongly that Ctenophora split much earlier from the metazoan tree and may have evolved a nervous system independently (Moroz et al., 2014; Moroz, 2015; Ryan et al., 2013).
8.1.2 Central and Peripheral Nervous System
In all bilaterian taxa, the nervous system is formed of two major components, the central nervous system (CNS) and the peripheral nervous system (PNS) (see Figure 8.2). In the nerve net of many cnidarians and ctenophores, regions of higher neuronal density are detected in some body parts that play specialized roles in feeding or locomotion. Examples are the ring ganglion surrounding the mouth opening of polyps and medusae (Garm, Poussart, Parkefelt, Ekström, & Nilsson, 2007; Mackie, 2004), or the ganglia at the base of polyp tentacles, associated with sensory structures called rhopalia (Nakanishi, Hartenstein, & Jacobs, 2009; Skogh et al., 2006). It is likely that from such local densities or ganglia true nerve centers evolved in higher phyla (Jacobs et al., 2007; Koizumi, 2007; Watanabe et al., 2009). Ganglia are formed by two classes of cells, motor neurons and interneurons. Motor neurons are large cells that connect to muscles and glands and thereby directly control body functions. Interneurons form connections within the CNS, integrating the activity of sensory input and motor neuron activity.

Figure 8.2 Division of Central and Peripheral Nervous System.
(A) Chelicerate Limulus polyphemus; (B) Platyhelminth Bdelloura candida.
Hanstroem 1968. Reproduced with permission of Springer.
The peripheral nervous system (PNS) consists of sensory neurons that are able to sense touch, motion, and sound (mechanoreceptors), position and state of the body (proprioceptors), smell and taste (chemoreceptors), and light (photoreceptors). Axons of sensory neurons projecting towards the CNS and axons of motor neurons going in the opposite direction form peripheral nerves. Typically, sensory neuronal cell bodies of invertebrates form part of peripherally located sense organs, or sensory ganglia closely attached to sense organs (Bullock & Horridge, 1965). In some instances, cell bodies of sensory neurons are integrated into the central ganglia (for example most sensory neurons in nematodes and plathyhelminths: Ehlers, 1985; Ward, Thomson, White, & Brenner, 1975; Wright, 1992; Zhu, Li, Nolan, Schad, & Lok, 2011). In these cases, the long dendritic fibers of the sensory neuron project along the peripheral nerves towards the sensory endings (sensory cilia) located in the epidermis or specialized sensory organs.
Part of the peripheral nervous system is associated with (and often developmentally derived from) the gut, providing the intestinal glands and muscles with sensory and motor innervation. This is called the “visceral,” “autonomic,” or (in most invertebrates) “stomatogastric” nervous system (Barna et al., 2001; Csoknya, Lengvári, Benedeczky, & Hámori, 1992; Hartenstein, 1997; Reuter & Gustafsson, 1985; Selverston, Elson, Rabinovich, Huerta, & Abarbanel, 1998).
8.1.3 Subepithelial, Basiepithelial and Invaginated Nervous Systems
The basic architecture of the central nervous system falls into three main types: subepithelial, basiepithelial, and invaginated. A subepithelial (subepidermal) nervous system consists of a system of ganglia or nerve chords located within the body cavity, that is, separated from the epidermis and muscle layer of the body wall by a basement membrane (see Figure 8.3A). A ganglion has an outer layer of somata (cell bodies) called the rind or cortex, which surrounds a central core of neuropil (axons, dendrites, and synapses). Each neuron sends a single process into the central neuropil. Synapses are formed between these processes; the cell bodies do not generally receive synapses and therefore do not participate in stimulus conduction. The term “nerve chord” describes an elongated array (“bundle”) of nerve fibers surrounded by neuronal cell bodies, as opposed to “nerve” (in the peripheral nervous system) or “tract” (in the central nervous system), which simply constitute a bundle of nerve fibers without accompanying cell bodies. The layout of a subepithelial CNS is shown schematically in Figure 8.3A (for the arthropod Drosophila melanogaster); it includes the brain, formed by one or several ganglia in the head region arranged around the pharynx, and the ganglia or nerve chords of the trunk. These can be either located all around the dorso‐ventral axis (e.g., in platyhelminthes) or concentrated ventrally (e.g., in annelids, arthropods; see §8.2 below). In segmented animals, such as arthropods and annelids, the ganglia are segmentally arranged. Each trunk segment has one corresponding segmental ganglion, and the brain is formed by the fusion of several segmental ganglia.

Figure 8.3 Architecture of the Central Nervous System in Bilaterian Animals.
(A) Subepidermal nervous system (arthropod Drosophila melanogaster); (B) Basiepithelial nervous system (nematode Caenorhabditis elegans); (C) Invaginated nervous system (Chordate Xenopus laevis).
In a basiepithelial nervous system (see Figure 8.3B), neurons form clusters or layers within the basal epidermis. The defining criterion is the epidermal basement membrane, which in a basiepithelial nervous system includes neuronal cell bodies and their processes. Basiepithelial nervous systems can be distributed fairly diffusely over the body, similar to the nerve net from which they presumably descended (as, for example, in hemichordates), or can form ganglia and chords in the head and trunk of the animal (as in nematodes) (see Figure 8.3B).
The invaginated nervous system, found in vertebrates and, in rudimentary form, in many lower deuterostomes, is thought to be derived from the basiepithelial configuration. Here, the epithelium overlying the neurons separates from the epidermis during early development and forms a continuous neural tube extending along the dorsal surface of the body (see Figure 8.3C). Neurons are organized in layers surrounding the central lumen of the neural tube. The subdivision into cortex and neuropil as described above for a subepidermal nervous system is absent. Instead, neuronal cell bodies and nerve cell processes are intermingled; synapses are formed on processes and cell bodies.
It is not clear whether the classification of CNS organization into the types above described has any phylogenetic significance. As outlined in more detail in §8.2, below, most basal protostome and deuterostome phyla possess a basiepithelial nervous system organization (see Figure 8.4). This finding, in conjunction with the assumption that the central nervous system descended from a basiepithelial nerve net, suggests that the basiepithelial organization may be primitive. The term “skin brain” was proposed for the hypothetical nervous system of the bilaterian ancestor (Holland, 2003). However, it is also possible that the basiepithelial and subepithelial types of nervous system organization have no profound phylogenetic implication, but are merely incidental to the overall number/density of neurons, or the embryonic development of the nervous system. In most taxa, the CNS originates from populations of neural progenitors located within the embryonic ectoderm. Frequently, for example, in insects and other arthropod taxa, these progenitors delaminate or invaginate into the body cavity, resulting in a subepithelial neural architecture from early stages onward (Hartenstein & Stollewerk, 2015). In other cases, as described for the annelid Capitella sp. (Meyer & Seaver, 2009), neural progenitors first produce a small basiepithelial brain which only secondarily adopts a subepithelial position. It is likely that a similar ontogenetic transition from basiepithelial to subepithelial will become apparent in other animal groups once relevant information on neural development becomes available.

Figure 8.4 Distribution of nervous system characters among major animal phyla.
Phylogenetic position of phyla is listed on the left. Column (A) shows presence or absence of peripheral nerve plexus (NN); shown in (B) is position of central nervous system (S subepidermal, B basiepithelial, I invaginated); (C): presence or absence of brain (Br); (D): distribution of nerve cords (Co) or ganglia (Ggl) [D dorsal; V ventral; DV dorsal (or lateral) and ventral].
8.1.4 Neuropil Compartmentalization
The multitude of neurites and synapses of the central nervous system form the neuropil. Neural circuits, the elusive objects of study of neurophysiologists and functional neuroanatomists, are embedded in the neuropil. As one of the first steps in approaching neural circuitry, it is important to define within the neuropil discrete compartments formed by neurons which share certain properties. Generally speaking, the interior of a compartment is defined by a high density of terminal neurite branches and their synapses. In many cases, a compartment is subdivided into smaller structurally and functionally distinct modules. On the outside, a compartment is surrounded by a frame of lower synaptic density, caused by the presence of bundles of axons interconnecting different compartments, as well as glial cells (Pereanu, Kumar, Jennett, Reichert, & Hartenstein, 2010; see Figure 8.5A–C).

Figure 8.5 Architecture of the Invertebrate Brain Neuropil.
(A) Schematic section of brain, showing relationship between neurons, glia, compartments, and compartment boundaries. (B, C): cross‐section of Drosophila brain hemisphere labeled with marker for synapses (B), highlighting compartments, and against glia (C), showing compartment boundaries. (D, E) Line drawings of schematic sections of insect brains, showing examples of Golgi‐stained neurons forming structured neuropils (antennal lobe, central complex, mushroom body) and unstructured neuropils.
Adapted from Hanstroem 1968. Reproduced with permission of Springer.
To introduce the concept of neuropil compartments, a sensory compartment, like the antennal lobe of the insect brain, may serve as an example (see Figure 8.5D). Insects, like most other higher animals, possess special sense organs. The insect antenna serves olfaction, and contains a large number of olfactory sensory neurons whose axons project towards a region of the brain neuropil called the antennal lobe (Stocker, 1994). As a result of the specialized, olfactory input, the neuropil of the antennal lobe acquires properties that make it different from neighboring neuropil domains lacking that input. Olfactory sensory neurons have distinctive, branched axonal endings; they form specialized synapses with the interneurons to which they are connected. Sensory neurons expressing the same olfactory receptor (and thereby sensing the same smell) converge and form a structurally visible unit called “glomerulus” within the antennal lobe (Gao, Yuan, & Chess, 200; Vosshall, Wong, & Axel, 2000: see Figure 8.5D). The interneurons receiving olfactory input project to other domains of the neuropil, which thereby also adopt characteristic structurally and functionally distinct properties. For example, the antennal lobe of the brain of many insects forms a massive projection on to a compartment called the mushroom body, which plays an important role in associative learning (see Figure 8.5D, E).
Compartments like the antennal lobe or mushroom body can be observed in the brains of most animals for which complex sensory organs have been described. An overview of these compartments and the sense organs they are directly or indirectly associated with is provided in §8.2 below. Compartments with a distinctive modular structure and well‐defined boundaries are referred to as “structured neuropil” in the literature; they contrast with other “unstructured” regions of the neuropil where compartment boundaries are less evident (see Figure 8.5E). For many invertebrate taxa that have small brains and lack distinctive sensory organs, the entire neuropil has so far remained “unstructured.” However, it is likely that, given the use of appropriate markers and applying a high enough resolution to the analysis of individual neurons, compartments will become apparent. For example, the reconstruction of the tiny brain neuropil of the microscopic flatworm Macrostomum lignano, containing only a few hundred neurons, has revealed clear compartments that differ in both intrinsic neurite “texture” (branching and orientation of neurites) and connectivity to sensory neurons and nerve cords (see §8.2.2.1 below).
8.2 Invertebrate Nervous Systems: A Brief Comparative Overview
For more than 150 years, neuroanatomists have analyzed the structure of nervous systems of invertebrates using a variety of histochemical techniques to visualize neurons and their fibers. The results of this work has been comprehensively surveyed in two books, the Vergleichende Anatomie des Nervensystems der wirbellosen Tiere [Comparative anatomy of the nerve systems of invertebrate animals] (Hanstroem, 1928; reprinted 1968), and Bullock and Horridge’s Structure and function in the nervous system of invertebrates (1965). Whereas modern techniques have added substantially to our knowledge of the neuroanatomy of many animal species used as neurobiological model systems (cnidarians, arthropods, annelids, molluscs, nematodes, and, to some extent, platyhelminths; for recent review, see Schmidt‐Rhaesa, Harzsch, & Purschke, 2016), little has been added to the classical literature dealing with the functional anatomy of the majority of phyla.
As an ordering principle for the following survey of invertebrate neuroanatomy I will use the phylogenetic classification proposed in a number of recent studies (Erwin et al., 2011; see Figure 8.4). Two taxa that branched off the phylogenetic tree at an early stage are placozoa and porifera. Extant members of these groups do not possess a nervous system. All elements of a nervous system are present in two other early branches, Ctenophora and Cnidaria (“diploblastic” animals because of their formation of only two germ layers, ectoderm and endoderm). Here, as described at the beginning of this chapter, sensory neurons and ganglion cells form a peripheral nerve net, with the first appearance of nerve centers associated with the mouth and tentacles.
A significant step towards the potential for higher structural complexity among the multicellular animals took place approximately 700–600 million years ago (MYA) with the appearance of the bilaterian, triploblastic animals. Bilateria are animals with an elongated, bilaterally symmetric body and a third tissue layer, the mesoderm, interposed between the outer ectoderm and inner endoderm. Specialized tissues and organs derived from the mesoderm, including muscle, vascular, excretory, and connective tissue, are thought to have allowed the bilaterian body to move more efficiently, grow to bigger sizes, and occupy new ecological niches. At the same time, neurons became organized into central ganglia and peripheral sensory organs. During the early Cambrian period, 540–515 MYA, bilateria split into different phyla, each with a characteristic body plan. Fossil collections such as the Burgess shale in Canada show that by 515 MYA most currently extant animal phyla had made their appearance (“Cambrian explosion”), implying that the common ancestor of animals as diverse as vertebrates, flies, nematodes and molluscs lived during the early Cambrian period. The early, almost simultaneous appearance of bilaterian taxa is one of the reasons why it is difficult to establish clear phylogenetic relationships between extant phyla. Most of the recently proposed trees assume that bilaterians comprise deuterostomes and protostomes, the latter further subdivided into the “supertaxa” ecdysozoa (molting animals; including arthropods, nematodes and others) and lophotrochozoa (molluscs, annelids, platyhelminths, many others; Aguinaldo et al., 1997; Dunn et al., 2008; Erwin et al., 2011; see Figure 8.4). However, the relationship of phyla within these supertaxa is, in large part, unsettled.
8.2.1 Invertebrate Deuterostomes
The nervous system of invertebrate deuterostomes—echinoderms, hemichordates, cephalochordates, and urochordates—consists of a basiepithelial nerve plexus with local condensations that, in most cases, derive from invaginations of the neuroepithelium (see Figures 8.4 and 8.6). Sensory neurons are distributed over much of the body surface; no large, complex sensory organs, such as image‐forming eyes, exist. A neuronal net spreading over the intestine (enteric nervous system) has been described for echinoderms (Garcia‐Arraras, Rojas‐Soto, Jiménez, Díaz‐Miranda, 2001), and is also likely to exist in other invertebrate deuterostomes.

Figure 8.6 The central nervous system of deuterostomes.
(A) Essential characteristics of nervous system architecture in hemichordates, urochordates, and vertebrates, represented as schematic sagittal sections. (B) Line drawing of nervous system of hemichordate Saccoglossus cambrensis. Top: nerve net, dorsal cord and ventral cord in collar region. Bottom: cross‐section of body wall, showing cytology of basiepithelial nerve plexus.
Knight Jones, 1952. Reproduced with permission of the Royal Society.
(C, D) Cross‐sections of dorsal cord of hemichordate Ptychodera flava. Neuronal marker Elav is expressed in ventral wall of epithelial dorsal cord (blue; black arrowhead in D). Scale bar: 100 mm. Neuropil of dorsal cord and peripheral nerve plexus is labeled by antibody against Acetylated tubulin (red; red arrowheads). Black arrowhead points at continuous strand of neuropil connecting dorsal cord with peripheral nerve plexus.
Nomaksteinsky 2009. Reproduced with permission of Elsevier.
(E) Schematic representation of brain of urochordate Botryllus schlosseri (left) and cephalochordate Branchiostoma floridae (right; both in dorsal view). Expression domains of Otx, Pax2/5/8 and Hox complex demarcatates region considered to be homologous to the vertebrate midbrain‐hindbrain boundary (red bar).
Lacalli 2001. Reproduced with permission of The Royal Society.
Hemichordates include enteropneusts (acorn worms) and sessile colony‐forming pterobranchs (sea angels). Both are characterized by a tripartite body structure: a prosome (proboscis in enteropneusts, tentacles in pterobranchs), mesosome (collar), and metasome (tail) (see Figure 8.6A). Condensations of the basiepithelial nerve layer occur in the collar. In some hemichordates, this region sinks in dorsally (sometimes also ventrally) and forms a tube‐like structure (dorsal cord), surrounded by densely packed neurons, similar to the neural tube in vertebrates (Kaul & Stach, 2010; Nomaksteinsky et al., 2009; see Figure 8.6A–C). Distinct neuropil compartments are absent in the hemichordate CNS. However, the dorsal cord of the collar may foreshadow the formation of the vertebrate brain. Thus, in the enteropneust Saccoglossus, the anteriormost Hox genes (Hox1, 2), characteristic for hindbrain in vertebrates, are expressed at the boundary between collar and tail (Lowe et al., 2003; Pani et al., 2012). Otx, demarcating forebrain and midbrain in vertebrates, occupies the entire collar region; Engrailed (en), at the vertebrate midbrain‐hindbrain boundary, is expressed in the posterior collar.
Like hemichordates, echinoderms include both free‐living groups, such as asteroids (star fish) and holothuroids (sea cucumbers), and sessile forms with tentacles (crinoids or sea lillies). Echinoderms are predominantly indirect developers which form from small, bilaterally symmetric larvae. These larvae have a similar three‐part body plan to hemichordates, and produce a simple basiepithelial nerve plexus with only a small number of neurons (Burke, 1978; Byrne & Cisternas, 2002; Nakano, Murabe, Amemiya, & Nakajima, 2006). During metamorphosis, the bilateral bodyplan is lost. Larval structures perish, and a five‐fold symmetric adult body evolves. Adult echinoderms possess a basiepithelial nerve plexus with condensations around the mouth (circum‐oral ring) and along the arms (radial nerve cords) (Cobb & Stubbs, 1981; Mashanov, Zueva, Heinzeller, Aschauer, & Dolmatov, 2010). The cords appear to develop from invaginated epithelial tubes (Märkel & Röser, 1991; Mashanov, Zueva, & Garcia‐Arraras, 2007), similar to the collar tube in hemichordates.
Urochordates (tunicates or sea squirts) are predominantly sessile, colonial filter feeders. As larvae they are motile tadpoles that resemble vertebrate larvae in many fundamental aspects. Some tunicates, including the appendicularians (larvaceans), retain a tadpole‐like body plan as adults. Tunicate larvae possess gills that function as a straining apparatus for filter feeding, a notochord, and a tripartite neural tube, forming an anterior sensory vesicle, an intermediate visceral ganglion, and a posterior spinal cord (Nicol & Meinertzhagen, 1991; Lacalli, 2001; Sorrentino, Manni, Lane, & Burighel, 2000; see Figure 8.6A, D). The neural tube is formed by an epithelial (ependymal) wall, surrounded by a small number (on the order of 100) of neurons. Given the paucity of neurons, the neuropil forms a loose plexus of nerve bundles surrounding the neuronal somata; specialized neuropil compartments are absent. Neurons associated with the sensory vesicle form the cerebral ganglion. Inserted in the epithelial wall of the sensory vesicle are groups of photoreceptive and ciliated mechano‐receptive sensory neurons, which are considered forerunners of vertebrate eyes and inner ear (Caicci, Burighel, & Manni, 2007; Lacalli, Varona, Arshavsky, Rabinovich, Selverston, 2004; Manni, Caicci, Gasparini, Zaniolo, & Burighel, 2004). Based on such morphological arguments, as well as the expression pattern of patterning genes like the Hox genes (Cañestro, Bassham, & Postlethwait, 2005; Lacalli, 2006; Wada, Saiga, Satoh, & Holland, 1998), the sensory vesicle is homologized with the forebrain/midbrain of vertebrates (see Figure 8.6D). The visceral ganglion, roughly corresponding to the vertebrate hindbrain in regard to gene expression pattern, contains a cluster of neurons with axons that project down the spinal cord—which itself has no neuronal cell bodies attached to it (see Figure 8.6A, D)—and innervate the musculature. The nervous system of cephalochordates (lancelets) is similar in its simplicity and tripartite layout to the tunicate tadpole brain (Lacalli, 1996, 2004, 2006; Lacalli, Holland, & West, 1994; see Figure 8.6A, D).
In urochordates (but not in cephalochordates), the larval neural tube degenerates during metamorphosis and is replaced (through proliferation of progenitors most likely contained within the sensory vesicle) by the cerebral ganglion of the adult sea squirt. Unlike its larval predecessor, the adult ganglion shows attributes of typical non‐deuterostome nervous systems, with neuronal somata (measuring in the hundreds) sourrounding a central neuropil (Koyama & Kusunoki, 1993; Manni et al., 1999). No overt similarity to the vertebrate brain is left; however, motor neurons of the cerebral ganglion, projecting fibers towards the muscles of the branchial basket via peripheral nerves, have been likened to hindbrain motor neurons based on gene expression patterns (Dufour et al., 2006).
8.2.2 Lophotrochozoans
Lophotrochozoans combine two major, previously known supra‐phyla, the lophophorates and spiralians, as well as several other phyla with unclear phylogenetic position, including chaetognaths, rotifers, and gastrotrichs (see Figure 8.4). Lophophorates are sessile, worm‐shaped animals with a conspicuous U‐shaped fold, the lophophore, located at their anterior end (see Figure 8.7B). The lophophore bears the multitude of fine tentacles used for filter feeding. Spiralians, including annelids, molluscs, nemertines, and platyhelminths are defined by the spiral mode of cleavage; many species belonging to these phyla also undergo a larval stage known as trochophore (hence the name “lophotrochozoans”). Finally, chaethognaths, rotifers and gastrotrichs are counted as members of the lophotrochozoa based on molecular data.

Figure 8.7 The central nervous system of lophotrochozoans.
(A) Essential characteristics of nervous system architecture in chaetognaths, platyhelminths, and gastrotrichs, represented as schematic sagittal sections. (B) Ventral ganglion of chaetognath Sagitta setosa (ventral view). Left photograph shows cortex (nuclei of neuronal cell bodies labeled blue) and neuropil (anti‐Synapsin, red); right photograph represents higher magnification of neuropil with subset of neurons (anti‐RFamide, red) forming distinct longitudinal tracts (scale bars 25 mm).
(A, B) Harzsch 2007. Reproduced with permission of BioMed Central Ltd.
(C) Brain of platyhelminth Macrostomum lignano (dorsal view; scale bar 25 mm). Nerve fibers and cilia of epidermis/pharynx are labeled by anti‐Tyrosinated tubulin (red); muscle fibers labeled by phalloidin (green); nuclei of all cells in blue. (D) Line drawing of nervous system of platyhelminth Bothrioplana semperi (dorsal view). (E) Line drawing of nervous system of ectoproct (=bryozoan) Cristatella mucedo (lateral view).
(D, E) Hanstroem, 1968. Reproduced with permission of Springer.
8.2.2.1 Chaetognaths, rotifers, gastrotrichs, flatworms.
Chaetognaths (arrow worms) are predatory worm‐like animals living among the plankton; rotifers (“wheeled animals”) and gastrotrichs (“hairy backs”) are microscopic benthic filter feeders. All three groups have a basiepithelial nervous system (see Figure 8.7A). Chaetognaths possess a supraesophageal brain and vestibular ganglion, connected to a large ventral ganglion. These ganglia are considered local condensations of a diffuse basiepithelial nerve net that extends throughout the body (Goto, Katayama‐Kumoi, Tohyama, & Yoshida, 1992; Harzsch & Müller, 2007; Rehkämper & Welsch, 1997; Shinn, 1997). Paired eyes and presumed olfactory organs project nerves into the brain neuropil; however, no specialized neuropil compartments have been described.
Platyhelminths (flatworms) represent a large and diverse phylum of animals and include free‐living and parasitic groups. All flatworms possess a relatively compact anterior brain organized in a way that resembles a typical invertebrate ganglion (see Figure 8.7A, C; for review, see Bullock & Horridge, 1965; Ehlers, 1985; Hartenstein, 2015; Reuter & Gustavsson, 1985). Nerve cell bodies are arranged in an external layer (cortex), and processes of the predominantly unipolar neurons form a neuropil in the center (see Figure 8.7A, C). Given the relatively small number of neurons (hundreds to a few thousands) and the absence of large sensory organs (see below), the neuropil does not show any overt compartmentalization. However, reproducible patterns of nerve fiber bundles (fascicles) produced by sensory axons entering the brain, or interneuronal connections, can be distinguished (e.g., for Macrostomum lignano; Morris, Cardona, De Miguel‐Bonet, & Hartenstein, 2007). Non‐neuronal cell types, including muscles and glands, penetrate the brain cortex and neuropil.
Issuing posteriorly from the flatworm brain are multiple, paired nerve cords. In most cases, three pairs (dorsal, lateral, and ventral) are distinguished. These longitudinal cords are transversally connected by more or less regularly spaced commissures; longitudinal cords and commissures form the so called “orthogon” (Hanstroem, 1968; see Figure 8.7A, D). Brain and orthogon are subepidermal structures; however, in many instances, a clearly visible basement membrane separating CNS from the overlying body wall is absent. Likewise, glial sheaths forming a cohesive sheath around the brain surface or nerve fiber tracts have not been observed, even though individual cells with glia‐like properties may occur (e.g., Biserova, 2008; Koopowitz & Chien, 1974; Sukhdeo & Sukhdeo, 1994).
Aside from the CNS, flatworms possess a peripheral nerve plexus, consisting of nerve cells and sensory cell processes. Mechanosensory and chemosensory neurons are located either in the epidermis or the central nervous system (see Figure 8.7; Ehlers, 1985; Rieger, Tyler, Smith, & Rieger, 1991). A high concentration of these ciliated sensory cells occurs at the anterior tip of the head, where they accompany a diverse array of glandular cells (apical complex). Flatworms have simple eyes and/or statocysts that are located in the brain.
Flatworms have a single gut opening, which was one argument by which previous structure‐based cladistic analyses placed them at the very bottom of the bilaterian animals (i.e., closest to cnidarians). A muscular pharynx, sometimes located near the head and sometimes more posteriorly, controls food uptake and egestion and is innervated by neurons forming a dense nerve ring around the pharynx wall (pharyngeal or stomatogastric nervous system).
One group of flatworms, the acoels, lack a gut and pharynx. Their interior is filled with a large syncytium (multinucleated cell), and digestion takes place in the syncytial cytoplasm. Recent molecular phylogenomic studies place the acoels (along with another flatworm taxon, called nemertodermatids) outside the flatworm phylum, and outside the lophotrochozoans altogether; these studies suggest that “acoelomorpha” (acoels and nemertodermatids) represent the sister taxon of all other bilateria (Dunn et al., 2008; Ruiz‐Trillo, Riutort, Littlewood, Herniou, & Baguña, 1999). Despite this deep phylogenetic separation, the structure of the central nervous system is similar to that described above for platyhelminths. Notably, and in contrast to some previous claims (e.g., Kotikova & Raikova, 2008; Reuter, Raikova, & Gustafsson, 1998), both types of flatworms have relatively compact anterior brains that consist of a cortex of neural cell bodies and a central neuropil with numerous commissural and longitudinal fiber bundles (Bailly, Reichert, & Hartenstein, 2013; Bery, Cardona, Martinez, & Hartenstein, 2010; Ramachandra, Gates, Ladurner, Jacobs, & Hartenstein, 2002; Semmler, Chiodin, Bailly, Martinez, & Wanninger, 2010).
Gastrotrichs and rotifers contain very small numbers of neurons (100 to a few hundreds). Clusters of neurons in the head form the cerebral ganglion (brain); neurons of the trunk form four paired nerve chords in gastrotrichs, whereas they are clustered in a paired pedal ganglion in rotifers (Clement & Wurdak, 1991; Hochberg, 2009; Hochberg & Litvaitis, 2003; Joffe & Wikgren, 1995; Ruppert, 1991; Teuchert, 1977). Complex sensory organs or neuropil compartments are absent. It is worth noting that the innervation of muscles by motorneurons in these small lophotrochozoans, as well as many other invertebrate taxa (e.g., platyhelminths discussed above) is different from the usual configuration that we know from vertebrates or “higher” invertebrates. There, motor neurons located in the central ganglia send axons towards the muscles, where they form neuro‐muscular synapses. Here, in rotifers, gastrotrichs, platyhelminths, nematodes, and other groups, muscle fibers or specialized processes of muscle cells invade the neuropil; neuro‐muscular synapses are an integral part of the central nervous system.
8.2.2.2 Lophophorates.
Four lophophorate phyla are distinguished: Ectoprocta (Bryozoa: mossy animals); Entoprocta (Kamptozoa: goblet worms); Phoronida (horseshoe worms); Brachiopoda (lamp shells). According to recent molecular analyses (e.g., Dunn et al., 2008; Erwin et al., 2011; Hejnol et al., 2009), these taxa are no longer considered members of a monophyletic group, but have branched off at various positions along the tree (see Figure 8.4). Detailed studies of the adult organization of the nervous system of lophophorates date back to the early 1900s (Hanstroem, 1968); several more recent papers look at the CNS structure of lophophorate larvae (Santagata, 2008, 2011; Wanninger, 2008).
Phoronids have a diffuse nerve net which, at the base of the lophophore, is condensed into a basiepithelial nerve ring. Larvae of phoronids, which share many characteristics with the typical trochophore larvae of other protostomes (annelids, molluscs), have an anterior basiepithelial apical ganglion connected by nerve cords to the ciliated rings and sensory cells of the body (Hay‐Schmidt, 1989; Lacalli, 1990; Santagata, 2002; Temereva, 2012; Temereva & Tsitrin, 2014).
The CNS of bryozoa (sessile, colonial filter feeders) consists of a paired ganglion (cerebral ganglion or brain) located close to the mouth; bilateral nerve tracts projecting out of the brain surround the pharynx, thus completing the circum‐oral nerve ring typical for most protostomes. Anteriorly, the brain is drawn out into two long processes (“ganglionic horns”) that form a U‐shaped structure underlying the lophophore (Hanstroem, 1968; Schwaha & Wanninger, 2012; Shunkina, Zaytseva, Starunov, & Ostrovsky, 2014; Weber, Wanninger, & Schwaha, 2014; Figure 8.7E). From these cords emanate many regularly spaced radial nerves that innervate the tentacles of the lophophore. The brain is reported to develop from an invagination of the ectoderm and therefore has an interior lumen, surrounded by inner and outer layers of neuronal cell bodies that enclose a central layer of neuropil (Gruhl & Bartolomaeus, 2008; Weber et al., 2014). Bryozoans possess a peripheral nerve plexus that contacts sensory receptors distributed in the tentacles and all over the body. Complex sensory organs are absent.
The CNS of brachiopods (“lamp shells”) and entoprocts (“goblet worms”), containing mostly colonial or solitary, marine filter feeders, consists of subepidermal ganglia. Entoprocts possess only one, paired ganglion located close to the pharynx (Schwaha, Wood, & Wanninger, 2010). In brachiopods, a paired cerebral ganglion and subesophageal ganglion are arranged around the pharynx. Nerves emanating from these ganglia project towards the digestive tract, the tentacles, and stalk (Hanstroem, 1968; Santagata, 2011).
8.2.2.3 Nemertines, annelids, molluscs.
Members of these groups of animals can grow to large sizes and inhabit marine, freshwater, and terrestrial environments. They possess complex sensory organs and a central nervous system formed by subepidermal ganglia, containing large numbers of neurons (tens of thousands to millions). Along with the appearance of complex sensory organs and increased neuron number, the brain neuropil exhibits distinct structural/functional compartments.
Nemertines (“ribbon worms”) are mostly marine worms. Many are predators; all are characterized by a retractible proboscis involved in prey capture and probing the environment. Nemertines have a paired dorsal (supraesophageal) and ventral (subesophageal ganglion; Beckers, Faller, & Loesel, 2011; Beckers, Loesel, & Bartolomaeus, 2013; Hanstroem, 1968; Turbeville, 1991; Turbeville & Ruppert, 1985; see Figures 8.7, 8.8). These give rise to ventral nerve cords, as well as smaller lateral and dorsal cords. Nerve cords and the commissural tracts interconnecting them, resemble the orthogon defined for flatworms (see above). However, nemertine neural structure is further developed than that of flatworms. Glial layers surround the brain/nerve cords at its outer surface, and separate cortex from neuropil. Aside from ciliary receptors located all over the body, nemertines possess complex, paired sensory organs in their head, including the frontal organ (chemoreception and/or mechanoreception), cerebral organ (probably olfactory in function), and (in some species) eyes. Sensory nerves project into the neuropil of the supraesophageal ganglion. In some species, the dorsolateral part of this ganglion exhibits glomeruli‐like neuropil condensations, formed by large masses of densely packed interneurons. We observe here for the first time an example of the structured neuropil compartment, typically seen in conjunction with the appearance of complex sensory organs. Structured neuropil compartments are even more pronounced in the brains of molluscs and annelids.
The diverse phylum of molluscs includes groups, considered phylogenetically basal, which share many characteristics with flatworms, as well as highly derived groups with complex nervous systems. Among the former are the aplacophorans (small, worm‐like deep‐sea animals) and polyplacophora (chitons). The nervous system of these animals consists of a subepidermal brain (“cerebro‐buccal ring”) located anterior to the mouth and two paired nerve cords, the lateral pallio‐visceral cord, and the ventral pedal cord (Eernisse & Reynolds, 1994; Moroz et al., 1994; Scheltema et al., 1996; Shigeno, Sasaki, & Haszprunar, 2007; see Figure 8.7). Regularly spaced commissures interconnect the cords, bestowing an orthogon‐like organization upon the polyplacophoran CNS. Two paired nerves connect the brain to ganglia innervating the foregut (esophageal ganglion) and muscles of the radula (the mollusc‐specific toothed tongue used to shred ingested food). Aplacophorans and polyplacophorans have a diffuse peripheral nerve net. Complex sensory organs are absent, and highly structured neuropil compartments have so far not been described.
More highly derived molluscs, including gastropods (snails), bivalves (clams) and cephalopods (squid and octopus), possess subepidermal ganglia and a peripheral diffuse nerve net. The arrangement of ganglia in these animals can be derived from the polyplacophoran condition described above (see Figure 8.7). Gastropods and bivalves have a cerebral ganglion (brain) that gives rise to two pairs of nerve cords. The ventrally located pedal cord innervates the muscular “foot” of gastropods and bivalves (arms in cephalopods); in most species, the cord has condensed into a large ganglion (pedal ganglion) connected to the brain by a fiber bundle, the pedal connective. The lateral, pallio‐visceral cord has also condensed into three paired ganglia, the pleural ganglia (innervating the mantle cavity), branchial ganglia (gills) and visceral ganglia (intestinal tract). Nerve tracts connecting the brain to ganglia innervating the pharynx and foregut (buccal ganglia) form the stomatogastric nervous system. In cephalopods, all ganglia are fused into one large “brain” that, in regard to cell number and complexity, surpasses many vertebrate brains. In addition, prominent optic lobes have evolved in conjunction with the large image‐forming eyes; intrabrachial ganglia, connected to the pedal ganglion, control movement of the arms.
Gastropods and cephalopods have complex sensory organs; accordingly, the brain neuropil exhibits structured compartments. The gastropod head has paired tentacles, two pairs in most terrestrial gastropods, one pair in many marine opisthobranchs (sea slugs). Tentacles carry eyes, often with lenses and many thousands of photoreceptors. In addition, tentacles act as olfactory organs. Specialized sensory nerves, including the optic nerve and tentacle nerve, carry the axons of these receptors to the cerebral ganglion. The cerebral ganglion of gastropods has three major compartments, called pro‐, meso‐, and metacerebrum (Chase, 2000; Elekes, 2000; Hanstroem, 1968; see Figure 8.8 schematic). The procerebrum, which receives the optic nerve and tentacle nerve, is formed by large numbers (upwards of 20,000) densely packed neurons (“globuli cells”) which form a structured neuropil whose function is the processing and retention of olfactory information. The mesocerebrum, an overtly left‐right asymmetric structure, is involved in mating behavior (Chase, 2000). The posteriorly located metacerebrum is connected to the skin of the head, lips, and mouth cavity. It emits the stomatogastric nerves to the buccal ganglia that control feeding behavior.

Figure 8.8 The Central Nervous System of Lophotrochozoans.
(A) Essential characteristics of nervous system architecture in nemertines, molluscs, and annelids, represented as schematic sagittal sections. (B–D) Schematic representation of head sensory organs and brain of nemertines (B), gastropod molluscs (C), and polychaete annelids (D; all in dorsal view). Sensory nerves in blue, structured neuropil compartments in purple. (E–G) Line drawings of neurons forming structured neuropil compartments. (E) Globuli cells of gastropod Helix pomata. (F, G) Sensory nerves, brain and mushroom body of polychaete Nereis diversicolor.
(F–G) Hanstroem, 1968. Reproduced with permission of Springer.
Inset in (G): photograph of mushroom body of Nereis diversicolor (scale bar 50 mm).
Heuer and Loesel, 2008. Reproduced with permission of Springer.
Annelids (segmented worms) include a wide variety of motile and sessile marine worms (polychaetes, echiurids, sipunculids), as well as leeches and terrestrial earthworms. The central nervous system of annelids consists of subepidermal ganglia (Hanstroem, 1968). The brain (cerebral ganglion, supraesophageal ganglion) is located in the head, anterior to the mouth, a region called prostomium; it receives input from sensory organs of the prostomium. The brain connects to the stomatogastric nervous system and a ventral nerve cord, which consists of a series of segmental ganglia. Segmental ganglia have the tendency to fuse; for example in many species of leeches, the four anterior ganglia have fused into one subesophageal ganglion, the four posterior ganglia into one caudal ganglion. Lateral and dorsal nerve cords, and the peripheral nerve net as seen in molluscs, are absent (see Figures 8.7, 8.8). Segmental ganglia of the ventral nerve cord contain motor neurons and interneurons which control body movement; examples of specific neuronal circuits, which have been elucidated in great detail for leeches, will be discussed in §8.4 of this chapter.
The prostomium of polychaetes possesses several complex sensory organs, including the palps, one or several paired antennae (tentacles), one or more paired eyes, and the nuchal organs. Palps, antennae and nuchal organs bear large arrays of sensory receptors, which are involved in olfaction and mechanoreception (Bullock & Horridge, 1965; Forest & Lindsay, 2008; Golding, 1992; Mill, 1978; see Figure 8.8). The brain, which receives the afferent nerves of these sensory organs exhibits three domains, termed forebrain (procerebrum), midbrain (mesocerebrum), and hindbrain (metacerebrum). The nerve from the palps terminates in the forebrain; eyes and antennae project to the inner part of the midbrain, and the nuchal organ to the hindbrain. The outer part of the midbrain contains large numbers of interneurons which form a structured neuropil, called the mushroom body (Hanstroem, 1968; Heuer & Loesel, 2008; Heuer, Müller, Todt, & Loesel, 2010; see Figure 8.8). It is thought that incoming afferents of all modalities, directly or via interneurons, gain access to the mushroom body. Afferents from the eyes and the nuchal organs also form structured neuropils in the inner midbrain (central optic neuropil; Heuer & Loesel, 2008) and hindbrain (nuchal commissure), respectively. The optic center and nuchal commissure are are “midline neuropils” which cross the midline between left and right brain hemisphere (see Figure 8.8). These midline neuropils, as well as the mushroom body, have been compared to (and may be homologous to) similar structured neuropils observed in arthropods (Heuer et al., 2010; see below). It appears that complex sensory organs and accompanying structured brain neuropils only occur in polychaetes, and have been lost in other annelid taxa, including earthworms or leeches.
8.2.3 Ecdysozoa
The ecdysozoa (animals with a cuticular exoskeleton that is renewed during molts) include two super‐phyla, pan‐arthropods (likely to be monophyletic) and cycloneuralians (probably para‐phyletic; Borner, Rehm, Schill, Ebersberger, & Burmester, 2014; Rota‐Stabelli et al., 2010; Telford, Bourlat, Economou, Papillon, & Rota‐Stabelli, 2008). Among the pan‐arthropods are insects and crustaceans (“tetraconata”), chelicerates (horseshoe crabs, scorpions, spiders, mites), myriapods (centipedes, millipedes), and onychophorans (velvet worms; see Figure 8.4); all of these groups are segmented animals with a subepidermal nervous system forming a complex brain and a ventral chain of segmental ganglia. Tardigrades (“water bears”) show many structural similarities to the segmented pan‐arthropods, but their inclusion in this clade is unclear. Cycloneuralians are unsegmented, worm‐like animals with simple, basiepithelial nervous systems; they include nematodes (round worms) and nematomorphs (hair worms), kinorhynchs, loriciferans, and priapulids.
8.2.3.1 Cycloneuralians and tardigrades.
The small, basiepithelial nervous system of cycloneuralians has been studied in great detail for a number of nematodes, most importantly Ascaris suum (intestinal roundworm; large parasitic nematode) and Caenorhabditis elegans (microscopic nematode living in the soil). Neurons of the nematode CNS form a ring‐shaped ganglion that surrounds the pharynx (pharyngeal nerve ring or brain) that emits a ventral, lateral and dorsal nerve cord (Hanstroem, 1968; White, Southgate, Thomson, & Brenner, 1980; Wright, 1991; see Figure 8.9A). The only neuronal cell bodies found in the ventral cord are those of motoneurons innervating the body musculature; posteriorly, neurons controlling mating and defecation form a tail ganglion. As described for platyhelminths and other lower lophotrochozoans, motoneurons have no peripheral axons innervating the muscles; instead muscle cells form processes that travel towards the ventral nerve cord, where they form synapses with motor neurons (see Figure 8.9B).

Figure 8.9 The Central Nervous System of Ecdysozoans.
(A) Essential characteristics of nervous system architecture in nematodes, priapulids, and arthropods, represented as schematic sagittal sections. (B) Schematic cross‐section of nematode Caenorhabditis elegans, showing basiepithelial dorsal and ventral cord and muscle process forming connection to ventral nerve fiber. (C) Line drawing of representative neuron of nematode C. elegans based on electron microscopic reconstruction. Neuron forms single nerve process with interspersed input and output synapses. (D–F) Schematic representation of head sensory organs and brain of arthropods (D: onychophoran; E: chelicerate; F: hexapod; all in dorsal view). Sensory nerves in blue, structured neuropil compartments in purple. Hatched lines indicate boundaries between segmental ganglia. Segmental ganglia of arthropods can be homologized based on anatomical and molecular criteria (for details, see text); green bar registers brains, indicating tritocerebrum (hexapod), pedipalpal ganglion (chelicerate), and ganglion innervating oral papilla (onychophoran) as homologous neuromeres. (G, H) Line drawings of neurons forming structured neuropil compartments. (G) Optic neuropils, mushroom body, and arcuate body of chelicerate. (H) Mushroom body, central complex, and antennal lobe of hexapod Periplaneta americana.
Hanstroem, 1968. Reproduced with permission of Springer.
The pharyngeal nerve ring, or brain, is composed of the anterior ganglion (somata of sensory neurons innervating the simple chemosensory and mechanosensory organs of the head), lateral ganglion, ventral ganglion, and retro‐vesicular ganglion. The latter ganglia contain a small number of motoneurons innervating the head and pharynx muscles, and interneurons integrating sensory inputs and synapsing on the motoneurons. The neuropil of the brain and nerve cords is exceedingly small and unstructured, because neurons are low in number (a few hundreds) and are mostly unbranched (White et al., 1980; see Figure 8.9C).
Kinorhynchs are microscopic benthic worms with a basiepithelial nervous system. The brain is formed by clusters of neurons associated with three circular nerve tracts surrounding the pharynx; multiple nerve cords innervate the trunk, but the unpaired ventral cord contains most neurons (Herranz, Pardos, & Boyle, 2013; Kristensen & Higgins, 1991; Neuhaus & Higgins, 2002). Priapulids (“penis worms”) are somewhat larger cylindrical worms living in the mud of shallow water; like nematodes and kinorhynchs they possess a basiepithelial nerve ring around the pharynx. A single ventral nerve cord projects into the trunk (Rehkämper, Storch, Alberti, & Welsch, 1989; Rothe & Schmidt‐Rhaesa, 2010; Storch, 1991; see Figure 8.9A).
8.2.3.2 Arthropods.
Arthropods, representing the largest phylum (by far), are segmented animals with jointed limbs. One distinguishes onychophorans, myriapods, chelicerates, crustaceans, and insects (see Figure 8.4). All of these taxa have subepidermal, ganglionic nervous systems, typically with hundreds of thousands to millions of neurons, and the neuroanatomy of many species has been described in considerable detail (Bullock & Horridge, 1965; Hanstroem, 1968; Strausfeld, 1976). Ganglia form an anterior brain and a ventral nerve cord. A peripheral nerve net is absent. The body of crustaceans and insects, which are more closely related to each other than to the remainder of the arthropods, has three domains (tagmata): head, thorax, and abdomen. Each of these domains comprises multiple more or less fused segments. The head is formed by a large anterior, unsegmented component (acron) and five segments flanking the mouth opening; the thorax has eight (most crustaceans) or three (insects) segments; segment number in the abdomen is highly variable. The acron (sometimes considered an additional, “ocular” segment; Schmidt‐Ott & Technau, 1992) carries the compound eyes and median ocelli (see Figure 8.9F). The first two segments have chemosensory appendages (two pairs of antennae in crustaceans; one pair of antennae and a gustatory organ in insects); the three segments posterior to the mouth opening (gnathal segments) are equipped with paired appendages (mandible, maxilla, labium) specialized for feeding (see Figure 8.9F). Thoracic segments carry large walking legs in insects and many crustaceans; abdominal segments have no appendages (insects) or small appendages involved in swimming (crustaceans) and other functions.
The brain of insects and crustaceans, according to the segmental organization of the head, is formed by six fused ganglia or “neuromeres” (where separate ganglia are not visible the term “neuromere,” which refers to a segmental unit within the nervous system, is preferable). Neuromeres of the acron and two first segments form the supraesophageal ganglion; neuromeres of the three gnathal segments are fused into the subesophageal ganglion (see Figure 8.9F). The anterior neuromere (protocerebrum) consists of large, multi‐layered optic lobes which process information from the compound eyes, and a central brain with a number of structured neuropil compartments (Hanstroem, 1968; Krieger et al., 2012; Loesel, Nässel, & Strausfeld, 2002; Strausfeld, 1976; Strausfeld, Sinakevitch, Brown, & Farris, 2009). The most prominent of these are the paired mushroom bodies, crucial for learning and memory (prominent in insects; reduced or absent in crustaceans), and the central complex, which consists of several layers of structured neuropil crossing the midline, and which plays important roles in motor control, spatial orientation/memory, and many other functions (see Figure 8.9F, H). The second neuromere (deutocerebrum) receives olfactory and mechanosensory input from the first antenna and possesses a highly structured neuropil compartment, the antennal lobe. The third neuromere (tritocerebrum) is also predominantly chemosensory, being innervated by the second antenna (crustaceans) and sensory organs located in the mouth cavity and the gut (see Figure 8.9F). The tritocerebrum also connects to the stomatogastric nervous system, formed by a series of peripheral ganglia associated with the intestinal tract.
The structure of the head and brain of Myriapods (centipedes, millipedes) closely resembles that of insects; some neuropil compartments, notably the optic lobe and central complex, are reduced in size (Hanstroem, 1986; Sombke et al., 2012).
The body of chelicerates (horseshoe crabs, spiders, scorpions, mites) has only two tagmata, the cephalo‐thorax (fused head and thorax), and abdomen. The cephalothorax includes an anterior acron, carrying the eyes, and six segments with appendages involved in chemoreception, feeding, and locomotion. Appendages of the first two segments are the cheliceres (“pincers” for grasping food) and pedipalps (leg‐like tactile organs in spiders; claws with pincers in scorpions; see Figure 8.9E); the posterior four segments carry walking legs. Ganglia of the cephalo‐thorax form a fused mass, comprising a supraesophageal ganglion and a ventral ganglion. Primitively (e.g., in horseshoe crabs), the supraesophageal ganglion includes only the protocerebrum; ganglia innervating the the cheliceres and pedipalps, as well as the walking legs, form part of the ventral cord (Babu, 1985; Hanstroem, 1968). In spiders and scorpions, the ganglion of the cheliceral segment is incorporated into the supraesophageal ganglion (see Figure 8.9E). Homologizing segments in chelicerates and insects/crustaceans is problematic. Based on neuroanatomical data, the first segment with its cheliceral neuromere corresponds to the insect/crustacean tritocerebrum because its commissural axons cross ventral to the foregut and it is connected to the stomatogastric nervous system (reviewed by Scholtz & Edgecombe, 2006; Weygoldt, 1985). This would mean that a chelicerate counterpart of the first antennal segment/deutocerebrum is missing. However, based on the pattern of Hox gene expression, the cheliceral segment is homologous to the first antennal segment of insects/crustaceans; its neuromere would then correspond to the deuterocerebrum (Damen et al., 1998), and the pedipalpal neuromere would be the tritocerebrum (this interpretation is represented in Figure 8.9E).
In accordance with the presence of large, complex sensory organs, chelicerates also have structured neuropil compartments. They comprise layered optic neuropils, bilateral mushroom bodies, and a central complex (Strausfeld, Weltzien, & Barth, 1993; Strausfeld & Barth, 1993). It is not yet clear in how far, functionally or ontogenetically, these compartments correspond to the structures of the same name in insects. In insects the mushroom bodies receive predominantly input from the antennal lobe and are involved in olfactory learning and memory. The mushroom bodies in spiders are targeted mostly by 2nd order visual interneurons (Strausfeld & Barth, 1993; Strausfeld, Hansen, Li, Gomez, & Ito, 1998; see Figure 8.9E, G). Also, the correspondence between the central complex in insects/crustaceans and spiders (where it is now called arcuate body; Strausfeld, Strausfeld, Loesel, Rowell, & Stowe, 2006) is not clear.
Onychophorans (velvet worms) are terrestrial, segmented, worm‐like animals with short limbs on each segment. The head has relatively small cup eyes, a pair of large chemosensory antennae, paired mandibles for mincing food, and oral papillae that produce mucus employed to snare prey. Eyes and antennae, as well as mandibles, are innervated by the supraesophageal ganglion; based on the pattern of innervation, as well as the expression pattern of Hox and other patterning genes, one can subdivide the onychophoran brain into an anterior brain (“protocerebrum”) associated with eyes and antennae, and a posterior brain (“deutocerebrum”) innervating the mandibles (Mayer et al., 2010; see Figure 8.9D). The oral papillae receive innervation from the first ganglion of the ventral cord. Structured compartments are found in the protocerebrum, and include antennal glomeruli, as well as mushroom bodies and a central complex (Homberg, 2008; Strausfeld et al., 2006).
Tardigrades have a subepidermal CNS that includes a brain (supraesophageal ganglion) and a ventral nerve cord, composed of a pharyngeal (subesophageal) ganglion and four segmental ganglia innervating the segmented body with its stubby appendages (Hanstroem, 1968; Mayer et al., 2013; Persson et al., 2012). Unlike arthropods and onychophorans, tardigrades possess only small numbers (20–30 per ganglion) of neurons in their brain and ventral chord, and complex sensory organs or structured neuropil compartments are absent.
8.3 Morphological Building Blocks of the Invertebrate CNS
8.3.1 Synapses
Synapses are the points of contact between neurons, or between neurons and other target cells, such as muscle, gland, or sensory receptor. Two types of synapses, chemical and electrical, can be distinguished. The ultrastructural criteria of a chemical synapse are vesicles, containing neurotransmitter, and membrane thickenings, corresponding to pre‐ and postsynaptic protein complexes involved in signal transmission, such as vesicle‐docking protein complexes (presynaptic) and receptors/ion channels (postsynaptic). Another presynaptic specialization found in many synapses is the synaptic ribbon, or T‐bar (because, in cross section, it adopts the shape of the letter “T”); this organelle is formed by protein complexes involved in the docking of vesicles to the presynaptic membrane (Wichmann & Sigrist, 2010; see Figure 8.10A). Based on their ultrastructural features, various classifications of synapses have been proposed. In his classical electron microscopic investigation, Gray (1959) defined type 1 (asymmetric) and type 2 (symmetric) synapses in the mammalian visual cortex. Type 1 synapses are characterized by a larger diameter of the postsynaptic (compared to presynaptic) membrane thickening. Type 2 synapses have presynaptic and postsynaptic membrane thickenings of equal diameter (symmetric). It was originally proposed that asymmetric synapses are associated with round vesicles and are excitatory, whereas symmetric synapses possessed flattened or irregularly shaped (pleomorphic) synaptic vesicles, and are inhibitory. However it became soon clear that synaptic membrane specializations (e.g., symmetric vs. asymmetric) are unrelated to the type of vesicles present (Ebner & Colonnier, 1975; 1978; see Figure 8.10B), and that functional characteristics of synapses cannot be deduced from Morphology ogical observations.

Figure 8.10 Ultrastructure of Synapses.
(A) Type 2 (asymmetric) synapse from rat cortex (cv clear synaptic vesicles; po postsynaptic element; pre presynaptic element; T T‐bar (synaptic ribbon).
Gray 1959. Reproduced with permission of John Wiley and Sons.
(B) Type 1 (asymmetric) and type 2 (symmetric) synapse from ccerebral cortex of turtle Pseudemys scripta.
Ebner and Colonnier 1975. Reproduced with permission of John Wiley and Sons.
(C, D) Synapses in brain of mollusc Aplysia californica: (C) Peptidergic synapse with dense core vesicles (dcv). (D) Mixed synapse with clear vesicles (cv) and ultralarge vesicles (ulv).
(C, D) Tremblay 1979. Reproduced with permission of John Wiley and Sons.
(E) Non‐polarized synapse from pharyngeal nerve net of ctenophore Beroe ovata.
Hernandez‐Nicaise ML 1973. Reproduced with permission of Springer.
(F) Polarized synapse from cnidarian Hydra pseudoligactis.
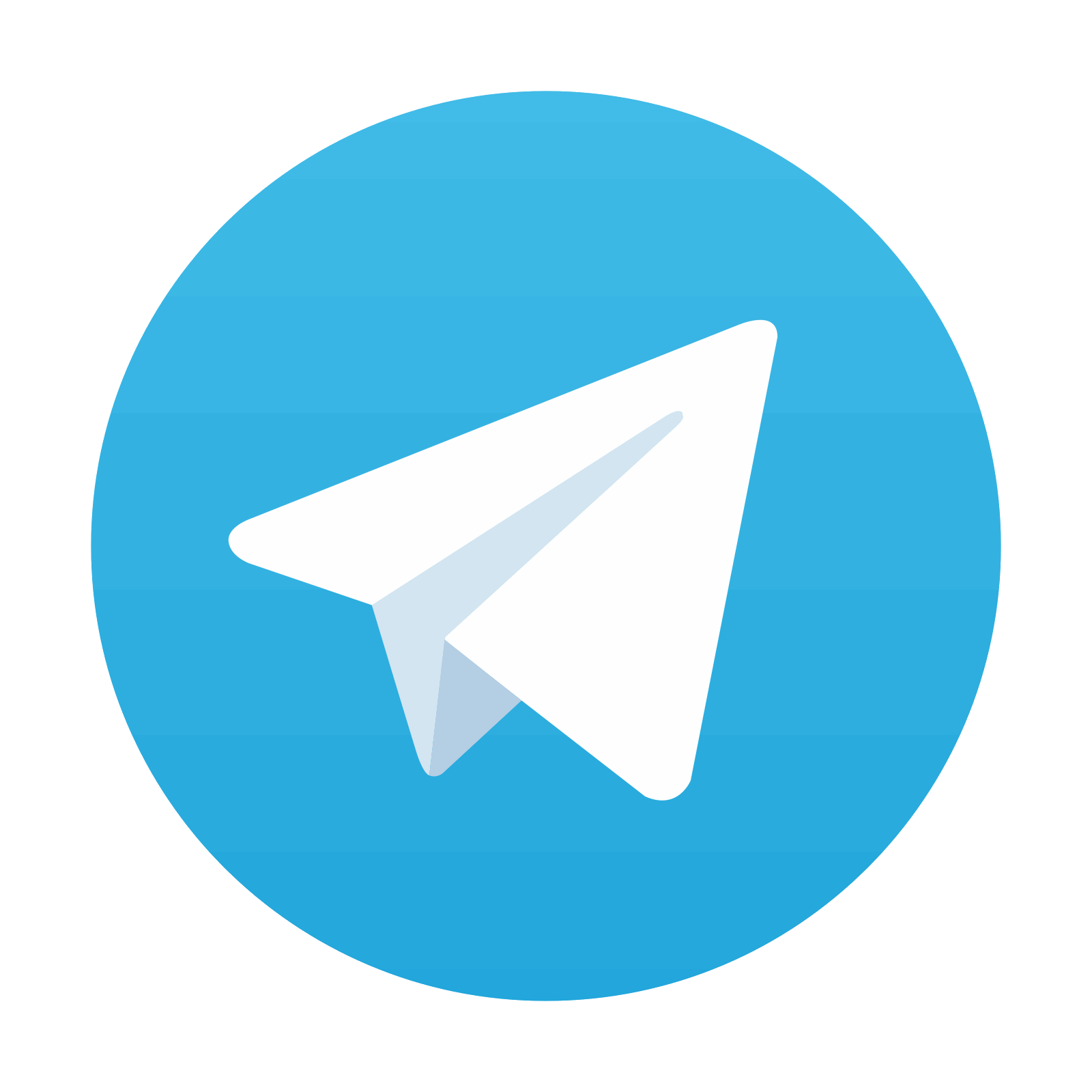
Stay updated, free articles. Join our Telegram channel

Full access? Get Clinical Tree
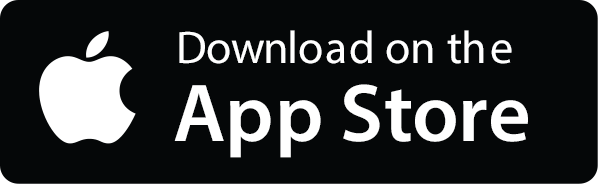
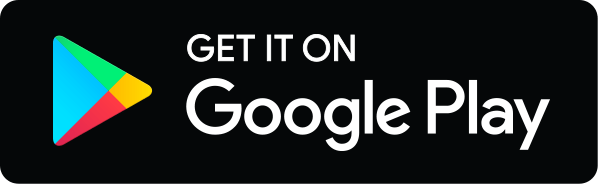