The Cerebellum
Anatomy of the Cerebellum
The cerebellum (Fig. 16.1 A and B), derived from the somatic afferent portion of the alar plate (rhombic lip), acts as a monitor or modulator of motor activity “originating” in other brain centers. Remarkable morphological features are its foliation, laminar structure, unique afferent and efferent connectivity of the cerebellar lobules, and molecular organization [38,114,123]. The cerebellum regulates muscle tone, posture, and equilibrium. One of the major cerebellar functions is the automatic excitation of antagonist muscles at the end of a movement, with the simultaneous inhibition of agonist muscles that initiated the movement.
The cerebellum is located in the posterior fossa of the skull, dorsal to the pons and medulla oblongata and separated from the occipital lobes by the tentorium cerebelli. On axial and coronal planes, a midline portion, the vermis, and two lateral portions, the cerebellar hemispheres, can be recognized. The falx cerebelli, a dural partition, partially separates both cerebellar hemispheres. The vermis is developmentally older and receives mainly spinocerebellar afferents, whereas the hemispheres have more complex fiber connections. Sagittal and coronal planes display best the three major components of the cerebellum, separated by fissures that lie near the axial plane: the anterior and posterior lobes (divided by the primary fissure) and the flocculonodular lobe (separated from the posterior lobes by the posterolateral or postnodular fissures). The anterior lobe comprises lobules I to V, the posterior lobe, lobules VI to IX, while the flocculonodular lobe comprises lobule X. A cerebellar tonsil forms the medioventral border of the posterior lobe of each cerebellar hemisphere [35].
Functional neuroimaging studies performed on human volunteers with noninvasive magnetic resonance imaging (MRI) have shown a somatotopic component of the cerebellar representation of foot, hand, and tongue movements [90]. For the hand, the center of activation was found in the ipsilateral anterior lobe in the intermediate hemispheric portion of the Larsell lobules H IV to V. Foot movements activated areas within the ipsilateral central lobule, in the Larsell lobules II–III medial and anterior to the corresponding hand areas. Responses for tongue movements were less consistent, but found posterior and lateral to the hand area, mostly at the posterior border of the anterior lobe extending in part to the Larsell lobules H VI—to VII [90].
Reported to have approximately 85 billion neurons [12], the cerebellum consists of a superficial cortex (made up of folia) surrounding the deep white matter, and three pairs of deep nuclei. The cortex of the cerebellum is folded into the cerebellar folia. Histologically, the cerebellar cortex has three layers: the outer molecular layer, the middle Purkinje cell layer, and the innermost granule cell layer. The granule cells are the only excitatory cells in the cerebellar cortex. Five cell types are distributed in these layers: (a) the outer basket cells and the inner stellate cells in the molecular layer, (b) Purkinje cells arranged in a single row in the Purkinje cell layer, and (c) granule cells and Golgi cells in the granule cell layer. Except for the Purkinje cells (projection neurons), whose axons project outside the cerebellum, all other cells are intrinsic neurons and establish connections within the cerebellum (Fig. 16.2).
The white matter of the cerebellum is made up of intrinsic, afferent, and efferent fibers. Incoming impulses to the cerebellum reach the dendrites and cell bodies of numerous Purkinje cells. The afferent fibers form the greater part of the cerebellar white matter, and when entering the cerebellum, segregate into one of three fiber systems: climbing, mossy, or multilayered. The climbing fibers are the terminal fibers of the olivocerebellar tracts and make multiple (1,000–2,000) synaptic contacts with one Purkinje cell. The mossy fiber system includes all other cerebellar afferent tracts except those that contribute to the climbing fibers and the multilayered system. In contrast to the climbing fiber system the mossy fiber system is diffuse, having multiple branches; so a single mossy fiber may stimulate thousands of Purkinje cells through the granule cell. The multilayered fiber system includes afferents to the cerebellum from the hypothalamus, raphe nuclei, and locus ceruleus, and also projects into the cerebellar cortex and deep cerebellar nuclei [2].
From an embryogenetic, phylogenetic, and functional standpoint, the cerebellum may also be subdivided into three units, the archicerebellum, the paleocerebellum, and the neocerebellum [22]. The archicerebellum corresponds to the flocculonodular lobe and is also called the vestibulocerebellum because it has a number of connections with the vestibular system. It also receives input from areas of the brain concerned with eye movements [111,112]. As a result of these connections, the vestibulocerebellum plays a role in the control of body equilibrium and eye movements. The paleocerebellum consists of the vermis of the anterior lobe, the pyramis, the uvula, and the paraflocculus. Also known as the spinocerebellum because it receives input mainly from the spinal cord, it plays a role in the control of muscle tone and the axial and limb movements. The neocerebellum (corticocerebellum), or cerebrocerebellum, consists of the middle portion of the vermis and most of the cerebellar hemispheres. Because it receives projections from the pons (corticopontocerebellar pathway), it is also termed the pontocerebellum. The neocerebellum projects fibers to the cerebral cortex through the thalamus, and plays a role in the planning and initiation of movements, as well as the regulation of fine limb movements.
FIG. 16.1. (A and B) The cerebellum. Midsagittal and axial sections.
FIG. 16.2. Schematic diagram of the cerebellar cortex.
Three pairs of deep nuclei on each side of the midline within the white matter core of the cerebellum receive input from the cerebellar cortex and incoming afferents. These nuclei are also the main source of cerebellar efferents. All of the efferent projections of the deep cerebellar nuclei are excitatory, except for projections to the inferior olive, which are inhibitory. From medial to lateral, these nuclei include the fastigial nucleus (nucleus fastigii), the nucleus interpositus (composed of the emboliform and globose nuclei), and the dentate nucleus (nucleus dentatus). On the basis of the connections of these nuclei, the cerebellum can be longitudinally subdivided [21,50] as follows: (a) a midline (vermal) zone, containing cerebellar neurons projecting to the fastigial nucleus; (b) an intermediate (paravermal) zone, containing neurons projecting to the nucleus interpositus; and (c) a lateral (hemisphere) zone, containing neurons projecting to the dentate nucleus.
There is separate somatotopic representation within each cerebellar nucleus, with caudal parts anterior, rostral parts posterior, trunk lateral, and limbs medial [47,126]. Each nucleus controls a different type or mode of movement [47,126], as follows:
1. The fastigial nucleus assists stance and gait and controls muscles only in the modes of sitting, standing, and walking. Therefore, fastigial lesions may cause abasia.
2. The nucleus interpositus assists segmental reflexes (e.g., those concerned with stability) and speeds the initiation of movements triggered by somatosensory cues that guide the response, stops unwanted and promotes wanted oscillations, and stabilizes holds. Therefore, nucleus-interpositus lesions may result in delayed check (rebound) responses, truncal titubation, abnormal rapid alternating movements, action tremor, oscillation of the outstretched extremities, and ataxia on finger-nose-finger and heel-knee-shin maneuvers.
3. The dentate nucleus assists in tasks requiring fine dexterity. Lesions of this nucleus or its projections cause delays in initiating and terminating movements, terminal and intention tremor, temporal incoordination in movements that require multiple joints, and abnormalities in the spatial coordination of hand and finger movements.
The cerebellum is connected to the brainstem by three large cerebellar peduncles: the caudal inferior cerebellar peduncle (restiform body), the middle cerebellar peduncle (brachium pontis), and the rostral superior cerebellar peduncle (brachium conjunctivum). The caudal and rostral peduncles contain afferent and efferent tracts; the middle peduncle is afferent. Most afferent fibers that project to the cerebellum do so through the inferior and middle cerebellar peduncle, whereas efferent fibers from the cerebellum traverse the superior and the inferior cerebellar peduncles.
The inferior cerebellar peduncle (restiform body) connects the cerebellum to the medulla oblongata and carries afferent and efferent fibers. Some afferent fibers of clinical importance include the following:
1. The dorsal spinocerebellar tract, originating in the dorsal nucleus of Clarke (T1–L2), which carries proprioceptive and exteroceptive information mostly from the trunk and ipsilateral lower extremity.
2. The cuneocerebellar tract, originating in the external arcuate nucleus, which transmits proprioceptive information from the upper extremity and neck.
3. The olivocerebellar tract, which carries somatosensory information from the contralateral inferior olivary nuclei.
4. The vestibulocerebellar tract, which transmits information from vestibular receptors on both sides of the body.
5. The reticulocerebellar tract, which arises in the lateral reticular and paramedian nuclei of the medulla.
6. The arcuatocerebellar tract, which arises from the arcuate nuclei of the medulla oblongata.
7. The trigeminocerebellar tract, which arises from the spinal and main sensory nuclei of the trigeminal nerve.
Efferent fibers in the restiform body are mainly cerebellovestibular pathways and constitute the fastigiobulbar tract, which courses in a separate pathway known as the juxtarestiform body. Other efferent fibers in the inferior cerebellar peduncle are the cerebelloreticular pathways.
The middle cerebellar peduncle (brachium pontis), the largest of the three cerebellar peduncles, connects the cerebellum to the pons and carries mainly the afferent fibers of the pontocerebellar (corticopontocerebellar) tract, which arises in the contralateral pontine gray matter and transmits impulses from the cerebral cortex to the intermediate and lateral zones of the cerebellum.
The superior cerebellar peduncle (brachium conjunctivum) connects the cerebellum to the midbrain. It contains mainly cerebellar efferent fibers, although it also contains a few afferent fibers. Afferent fibers of the superior cerebellar peduncle include the following:
1. The ventral spinocerebellar tract, which transmits proprioceptive and exteroceptive information from levels below the midthoracic cord.
2. The tectocerebellar tract, arising in the superior and inferior colliculi, which carries auditory and visual information.
3. The trigeminocerebellar tract, which carries proprioceptive fibers from the mesencephalon and tactile information from the chief sensory nucleus of the trigeminal nerve.
4. The cerulocerebellar tract, which carries fibers from the nucleus ceruleus.
Efferent fibers of the superior peduncle include the following:
1. The dentatorubral tract, which carries output to the contralateral red nucleus. Many of the fibers ending in this nucleus are branches of the larger dentatothalamic tract.
2. The dentatothalamic tract, which transmits output to the contralateral ventrolateral nucleus of the thalamus.
3. The uncinate bundle of Russell, which carries output to the vestibular nuclei and reticular formation.
Vascular Supply of the Cerebellum
The blood supply to the cerebellum is derived from the posterior inferior cerebellar arteries, the anterior inferior cerebellar arteries, and the superior cerebellar arteries (Fig. 16.3) [4,105]. The branches of these three vessels anastomose with branches from the corresponding vessels on the opposite side to provide a rich anastomotic network.
FIG. 16.3. Course of the cerebellar arteries. SCA = superior cerebellar artery; AICA = anterior inferior cerebellar artery; PICA = posterior inferior cerebellar artery. (Adapted from Amarenco P. The spectrum of cerebellar infarctions. Neurology 1991;41:973–979, with permission.)
1. The posterior inferior cerebellar artery (PICA) arises from the intracranial vertebral artery and supplies the half of the cerebellum below the plane of the great horizontal fissure, encompassing the lateral medullary tegmentum, inferior cerebellar peduncle, the ipsilateral portion of the inferior vermis, and the inferior surface of the cerebellar hemisphere. The vermian or medial branch of the PICA supplies the medial cerebellum and the dorsolateral medulla oblongata, and the tonsillar-hemispheric or lateral branch supplies the inferoposterolateral aspect of the cerebellum, but not the medulla.
2. The anterior inferior cerebellar artery (AICA), which often arises about 1 cm above the origin of the basilar artery, most frequently as a single vessel, provides supply to the anterior petrosal surface of the cerebellar hemisphere, flocculus, lower portion of the middle cerebellar peduncle, and lateral pontomedullary tegmentum. Typically, the AICA gives origin to an internal auditory artery that enters the internal acoustic meatus. When the PICA is missing, the territory of the AICA includes the territory of the PICA.
3. The superior cerebellar artery arises near the distal segment of the basilar artery just below the terminal bifurcation into the paired posterior cerebral arteries, and supplies the upper surface of the cerebellar hemisphere, ipsilateral portion of the superior vermis, most of the dentate nucleus, upper portion of the middle cerebellar peduncle, superior cerebellar peduncle, and lateral pontine tegmentum. The oculomotor nerve (CN III) and the trochlear nerve (CN IV) run between the superior cerebellar artery and the posterior cerebral artery.
Clinical Manifestations of Cerebellar Dysfunction
The classic report of Gordon Holmes describes cerebellar dysfunction in patients with wound injuries to the cerebellum [58,59]. The cerebellum controls the rate, direction, range, and force of voluntary movements, and through its vestibular connections, corrects and adjusts the individual’s upright position in space. Thus, cardinal features of cerebellar dysfunction involve disturbances in motor control, muscle tone regulation, and coordination of skilled movements, and are briefly discussed here.
Hypotonia
Hypotonia accompanies acute hemispheric lesions and is seen less often with chronic lesions. The hypotonia is ipsilateral to the side of the cerebellar lesion and is often more noticeable in the upper limbs, particularly in the proximal musculature. Hypotonic extremities have decreased resistance to passive stretching of the muscles and often exhibit pendular muscle stretch reflexes, which may also be diminished. Occasionally, cerebellar lesions may be associated with increased tone of the extremity due in part to secondary brainstem (corticospinal tract) compression. Hypotonia occurs only with neocerebellar lesions and is probably the result of decreased fusimotor activity secondary to cerebellar injury, especially to the dentate nucleus, resulting in a decreased response to stretch in the muscle spindle afferents.
Ataxia or Dystaxia
Cerebellar disorders result principally from defective timing of sequential contractions of agonist and antagonist muscles [48]. Ataxia, regarded as the “cerebellar sign par excellence,” refers to a disturbance in the smooth performance of voluntary motor acts causing muscular incoordination or impaired balance [23]. The movements err in speed, range, force, and timing. In the absence of cerebellar inhibitory and modulating influences, skilled movements originating in the cerebral motor cortex become inaccurate and poorly controlled. Ataxia may affect the limbs, the trunk, or gait. Presentation may be acute (e.g., cerebellar hemorrhage, biotinidase deficiency, phenytoin intoxication, acute cerebellar ataxia following chickenpox); episodic (e.g., autosomal dominant channelopathies associated with mutations in the KCNA1 and CACNA1 A genes, basilar migraine, Hartnup’s disease); or chronic progressive or nonprogressive (e.g., cerebellar tumors, spinocerebellar ataxias [SCA], Friedreich’s ataxia, ataxia telangiectasia, hypothyroidism, gluten sensitivity, paraneoplastic cerebellar degeneration) (Tables 16.1, 16.2, and 16.3) [26,41,46,52,54,55,56,60,61,67,84,85,86,87,102,104,120,141]. Inherited ataxias comprise a heterogeneous group of disorders characterized by variable combinations of progressive degeneration of the cerebellum and spinocerebellar tracts associated with clinical manifestations of pyramidal, extrapyramidal and peripheral nervous system dysfunction, and cerebellar or olivopontocerebellar atrophy on neuroimaging studies. Hereditary ataxias are further classified into autosomal dominant, autosomal recessive, and X-linked forms. Autosomal dominant cerebellar ataxias typically present in adulthood, and are currently classified as SCA 1 to 30 according to the specific gene or chromosomal locus associated with these disorders [86,109]. Two of these SCAs are briefly described here. Clinical features suggestive of SCA 3 (Machado-Joseph disease) include progressive cerebellar ataxia, nystagmus, ophthalmoplegia, facial fasciculations, facial dystonias, eyelid retraction with bulging eyes, and parkinsonism [110]. Features suggestive of dentatorubropallidoluysian atrophy encompass cerebellar ataxia, myoclonus, choreoathetosis, dystonia, parkinsonism, psychiatric disturbances, epilepsy and dementia [88].
TABLE 16.1 Causes of Acute Ataxia
Autosomal recessive ataxias typically result in early onset cerebellar ataxia associated with various neurologic, ophthalmologic, and systemic manifestations [46]. Pathologically characterized by the degeneration of the spinocerebellar tracts, dorsal columns, and to a lesser extent, the corticospinal tracts, Friedreich’s ataxia is the most common of the autosomal recessive ataxias. Age at onset is typically 5 to 25 years. Friedreich’s ataxia is caused by a deficiency of the mitochondrial protein frataxin. It is characterized by slowly progressive limb and gait ataxia, dysarthria, rhythmic head tremor, abnormal eye movements (fixation instability, saccadic dysmetria, disruptive pursuit, and vestibular abnormalities), optic atrophy, areflexia of knee and ankle jerks, loss of vibration and position sense, axonal polyneuropathy, extensor plantar responses, hypertrophic cardiomyopathy, diabetes, pes cavus, and kyphoscoliosis [33,39,40,45].
TABLE 16.2 Causes of Episodic/ Recurrent Ataxia
Ataxia secondary to cerebellar injury characteristically persists in spite of visual cues (unlike sensory ataxia which is more pronounced with eyes closed). The term ataxia includes other abnormalities of voluntary movement control, such as asynergia (lack of synergy of the various muscle components in performing more complex movements so that movements are disjointed and clumsy and broken up into isolated successive parts), dysmetria (abnormal range, force and excursions in movement), dysdiadochokinesia (impaired performance of rapidly alternating movements), and past-pointing. Dysmetria is best appreciated with the finger-nose test, or in the case of the lower extremities, heel-knee-shin or great toe-to-examiner’s finger test. The finger-nose test may show a tendency to fall short or overshoot the examiner’s finger (past-pointing). The heel-knee-shin test may reveal a wavering of the heel away from the line of the shin. When an opposed motion is suddenly released, an impaired checking response and an excessive rebound phenomenon are also involved.
Gait disturbance is one of the most disabling manifestations of cerebellar disease. Typically, patients with cerebellar disease have a wide-based stance and a gait [49] with increased trunk sway, irregular stepping with a tendency to stagger as if intoxicated, and impaired tandem walking and tandem gait paradigm with inappropriate timing of foot placement [118]. Truncal instability may be manifested by falls in any direction. Truncal ataxia and titubations suggest a midline cerebellar lesion.
Cerebellar Dysarthria
Dysarthria occurring with cerebellar disease is generally characterized by abnormalities in articulation and prosody [30,50]. These two abnormalities may occur together or independently. Speech production is often labored with occasional excessive facial grimacing. Comprehension remains intact. Cerebellar dysarthria has been described as scanning, slurred, staccato, explosive, hesitant, slow altered accent, and garbled speech [100]. The dysarthria may be a result of a generalized hypotonia, and may affect intonation rather than articulation [64]. The development of agrammatic speech after right cerebellar infarction supports the hypothesis that the cerebellum provides temporal interplay among the neural structures underlying the processes responsible for the production of sentences [115].
TABLE 16.3 Causes of Chronic Ataxia
Functional MRI studies among healthy volunteers have shown that the cerebellar representation of the tongue and oral facial muscles corresponds to the areas involved in patients with cerebellar dysarthria. In a series of 162 cases of focal cerebellar disease, 31 patients had dysarthria, of whom 22 had predominantly or exclusively left hemispheric lesions [71]. Cerebellar hemispheric lesions were associated with speech disorders more often than vermal lesions. Dysarthria was especially evident after damage extending into the superior paravermal segment of the left cerebellar hemisphere. Because cerebrocerebellar connections are predominantly contralateral, and the nondominant cerebral hemisphere is concerned with prosody of speech [71,100], the authors concluded that the dominance of the left cerebellar hemisphere in the regulation of speech (melody and continuity) may be due to this hemisphere accessing the nondominant cerebral hemisphere. Confirming the importance of the superior cerebellum in voice control, other authors have found dysarthria, characterized by irregularly distributed articulatory deficits and slowed speech tempo, to be as common with right-sided as with left-sided lesions [2]. Silveri et al. reported a patient with agrammatical speech after a PICA territory infarction, suggesting a right cerebellar hemispheric dominance for language function [115]. Isolated cerebellar dysarthria, without any other deficit, has also been reported with a small infarct in the left paravermal zone of the rostral cerebellum (lobulus simplex and semilunaris superior) in the territory of the medial branch of the superior cerebellar artery [5], with right paravermal segment infarcts, with lesions in the upper paravermal area of the right cerebellar hemisphere [132], as well as with an intact dentate nucleus [1].
Clinical and neuroimaging studies suggest that the cerebellum is involved in language tasks. Transient mutism after excision of cerebellar tumors (vermian neoplasms) has been increasingly recognized after posterior fossa surgery in children. In children, its incidence is estimated between 8% and 31% [37]. Whether this syndrome is tumor specific or not remains debatable. Symptoms usually develop one to three days after surgery. In many instances, these children also have oropharyngeal apraxia, with difficulty initiating the chewing and swallowing process, global impairment in the initiation of voluntary activity, impaired eye opening, and urinary retention [95]. It has been speculated that transient bilateral involvement of the dentato-rubro-thalamic tract may lead to this state of mutism and subsequent dysarthria [24,44,96,98,133,134]. Other neurologic lesions known to cause mutism include damage to Broca’s area, damage to the supplementary motor area, bilateral damage to the reticular formation of the mesencephalon (akinetic mutism), the locked-in syndrome and chronic vegetative state, Marchiafava-Bignami disease, severe pseudobulbar palsy due to diffuse bilateral cerebral hemispheric dysfunction, bilateral thalamic damage following thalamotomy for Parkinson’s disease, corpus callosotomy, surgical resection of the supplementary motor cortex of the dominant hemisphere, and bilateral pharyngeal or vocal cord paralysis (e.g., due to bulbar poliomyelitis or Guillain-Barré syndrome) [20,32].
Tremor
Lesions of the cerebellum, especially those affecting the dentate nucleus, induce a kinetic (intention) tremor occurring during activity. A static (postural) tremor may also occur. Lesions of the dentate nucleus may result in tremor because they interrupt a rubro-olivo-cerebellar circuit [69]. Patients with Wilson disease may have tremor or ataxic dysmetria. Cerebellar disease may also be associated with cerebellar fits; these are episodes of decerebrate rigidity usually seen with large midline cerebellar mass lesions [139].
Ocular Motor Dysfunction
Nystagmus is frequently observed in association with cerebellar disorders. Gaze-evoked, upbeat, rebound, and abnormal optokinetic nystagmus may be seen with midline cerebellar lesions [43,50]. Periodic alternating nystagmus is seen with lesions of the uvula and nodulus or with lesions involving their connections with the brainstem vestibular nuclei. Downbeat nystagmus may also occur with posterior midline cerebellar lesions involving the vestibulocerebellum [75]. Positional nystagmus, mimicking positional nystagmus of the benign paroxysmal type, may occur in patients with posterior fossa tumors. Ocular dysmetria [29]—a conjugate overshoot and undershoot of a target with voluntary saccades—may be seen with midline [111] or lateral [97] cerebellar lesions. Other ocular signs seen with cerebellar disorders include irregular tracking, saccadic breakdown of pursuit, ocular flutter, opsoclonus, ocular bobbing, paresis of conjugate gaze, square wave jerks at rest, skew deviation, ocular tilt reaction, and failure to suppress the vestibulo-ocular reflex [13,51,127]. Because most of the disorders that give rise to these abnormalities also affect other brainstem structures, the cerebellar role in their genesis has not been fully defined. In general, most “cerebellar” eye signs cannot be precisely localized to specific areas of the cerebellum. (See Chapter 8 for a discussion of cerebellar control of eye movements.)
Nonmotor Manifestations
The role of the cerebellum in the modulation of complex non-motor behaviors, including various aspects of cognition and linguistic functioning are becoming unraveled [34,36,53,68,76,77,80,81,103,113,131,137,138]. Accumulating evidence also supports the idea that the cerebellum may be altered in a wide range of psychiatric disorders including schizophrenia and bipolar disease [14] as well as addictive behaviors [130]. Moreover, a growing body of data also gives credence to the contribution of the cerebellum to the modulation of a variety of behavioral processes in humans such as dyslexia [89], schizophrenia [11] and autism [3]. Subjects with dyslexia have been found to have magnetic resonance imaging (MRI) voxel differences on the right cerebellar declive and right lentiform nucleus [93]. In autism, postmortem histopathologic studies have shown marked hypoplasia within the cerebellar vermis and variable loss of Purkinje cells throughout the cerebellar hemispheres and archicerebellum. Abnormalities have also been found in the fastigial and interposed nuclei, whereas the anterior lobe of the cerebellum and vermis have not been involved [18,31,80]. Bipolar disorders were also noted in 3 of 15 subjects after focal cerebellar lesions; lesions included right cerebellar hypoplasia, bilateral cerebellar atrophy, and left midbrain pathology [70]. A cerebellar cognitive affective syndrome, characterized by impaired executive functioning, personality changes associated with blunted effect or disinhibited and inappropriate behavior, visuospatial disorganization, impaired visual-spatial memory, mild anomia, agrammatism, and dysprosody, has been reported [108]. The neurobehavioral presentation was more evident in those patients with pancerebellar disease and in those with acute onset cerebellar disease. Lesions of the posterior lobe were particularly responsible for the disturbed cognitive behaviors in the generation, whereas the cerebellar vermis was more consistently involved in patients with a more pronounced affective symptomatology. The anterior lobe of the cerebellum has not been implicated to the same extent in the generation of these cognitive/behavioral manifestations [107,108]. A disruption of the cerebellar modulation of neural circuits linking prefrontal, posterior parietal, superior temporal, and limbic cortices with the cerebellum has been proposed as the mechanism for this syndrome. On the basis of the several reciprocal anatomic connections between the cerebral cortex and cerebellum, this appears to be a plausible explanation. More recently, lesions in the cerebro-ponto-cerebellar pathways have been proposed as a plausible anatomical site for pathological laughter and crying [92]. Contralateral cerebellar hypometabolism has been noted among aphasic patients with left cerebral infarcts or hemorrhages [57]. Cerebellar disease may be associated with macrographia. In addition, typical features of spatial dysgraphia, with omission and repetition of strokes and letters, have been described in a patient with marked cerebellar atrophy (cerebellar hemispheres more involved than the vermis) associated with diffuse corticosubcortical atrophy [116]. A discoordination between planning of the movement and performance, due to a lack of the cerebellar modulation between premotor cortex and proprioceptive afference during the ongoing handwriting, has been postulated as an explanation for this observation [116].
Cerebellar Syndromes
The exquisiteness of high resolution structural MRI has fueled renewed interest in human cerebellar lesion studies [128]. In general, disorders predominantly involving the midline cerebellum affect primarily the truncal musculature and body equilibrium. In contrast, disorders affecting primarily the cerebellar hemispheres have an ipsilateral impairment of the voluntary movements of the fingers and legs as their most salient deficit. The cerebellar syndromes may be divided as follows:
1. The rostral vermis syndrome (anterior lobe)
2. The caudal vermis syndrome (flocculonodular and posterior lobe)
3. The hemispheric syndrome (posterior lobe, variably anterior lobe)
4. The pancerebellar syndrome (all lobes)
Rostral Vermis Syndrome
The clinical characteristics of this syndrome include the following:
1. A wide-based stance and titubating gait
2. Ataxia of gait, with proportionally little ataxia on the heel-to-shin maneuver with the patient lying down
3. Normal or only slightly impaired arm coordination
4. Infrequent presence of hypotonia, nystagmus, and dysarthria
This syndrome is best exemplified by the restricted form of cerebellar cortical degeneration that occurs with unknown prevalence in the alcoholic population [135]. There is selective atrophy of the anterior and superior vermis, particularly the superficial folia, with lesser involvement of the caudal vermis, anterior parts of lateral lobes, flocculus, and paraflocculus. Morphometric methods have further demonstrated a remarkable Purkinje cell loss and densities especially in lobules I to IV, IX, and X of the vermis [25]. A Japanese autopsy study found the following sequence of involvement: anterior superior vermis; anterior superior hemisphere; anterior inferior hemisphere; and finally anterior inferior vermis [140]. Clinically, these patients present with incoordination of gait of the legs with little involvement of the arms, speech, or ocular motility.
Caudal Vermis Syndrome
The clinical characteristics of this syndrome include the following:
1. Axial disequilibrium and staggering gait
2. Little or no limb ataxia
3. Sometimes spontaneous nystagmus and rotated postures of the head
This syndrome is typically seen with disease processes that damage the flocculonodular lobe, especially medulloblastoma in children. Medulloblastomas account for approximately 20% of pediatric brain tumors. As these tumors grow, a hemispheric cerebellar syndrome may be superimposed due to neocerebellar involvement. Metastasis may occur throughout the craniospinal axis. Patients with tumors in either the vermis or the cerebellar hemispheres may present with symptoms and signs of increased intracranial pressure.
Surgical transection of the posterior inferior cerebellar vermis may result in marked impairment of tandem gait with minimal impairment of regular gait, standing, and hopping on one foot. Visually guided limb movements and speech remain unaffected. Surgical disruption of the parallel fibers crossing the midline cerebellar cortex may be a critical variable accounting for these clinical features known as the posterior vermal split syndrome [17].
Cerebellar Hemispheric Syndrome
Patients with this syndrome typically show incoordination of ipsilateral appendicular movements, particularly when they require fine motor coordination. Thus, it affects mainly muscles closely controlled by the precentral cortex, such as those involved in speech and finger movements. The most likely etiologies of a cerebellar hemispheric syndrome include infarcts, neoplasms, and abscesses [50].
Pancerebellar Syndrome
This syndrome, a combination of all other cerebellar syndromes, is characterized by bilateral signs of cerebellar dysfunction affecting the trunk, limbs, and cranial musculature. It is seen with infectious and parainfectious processes, hypoglycemia, hyperthermia, paraneoplastic cerebellar degeneration associated with small cell lung cancer (anti-Hu antibodies, ZIC antibodies) breast and ovarian carcinomas (anti-Yo antibodies), or Hodgkin’s lymphoma (Tr antibodies) and other toxic-metabolic disorders [136].
Gilman has suggested that, for localization purposes, the cerebellum should be viewed as a sagittally oriented structure containing three zones: midline, intermediate, and lateral [49,50]. The midline zone encompasses the anterior and posterior parts of the vermal cortex, the fastigial nucleus, and the associated input and output projections. Many of these projections are concerned with posture, locomotion, the position of the head in relation to the trunk, and the control of extraocular movements. Thus, cerebellar signs resulting from midline cerebellar disease are characterized by disorders of stance and gait, truncal titubation, rotated postures of the head, and disturbances of eye movements. The intermediate zone consists of the paravermal region of the cerebellar cortex and the interposed nuclei on each side. Neuronal activity in this region appears to be involved in the control of movement velocity, force, and the pattern of muscle activity, but clinical disorders related to disease of this zone have not been clearly delineated. The lateral zone consists of the cerebellar hemisphere and the dentate nucleus of each side. Neural units in this zone are involved in the planning of movements in connection with neurons in the precentral region of the cerebral cortex; lesions result in abnormalities related to voluntary movements and include hypotonia, dysarthria, dysmetria, dysdiadochokinesia, excessive rebound, impaired check response, kinetic and static tremors, decompensation of movements, past-pointing, and eye movement abnormalities [49,50].
Syndromes of Cerebellar Infarction
Cerebellar infarcts result from thrombotic or embolic occlusion of a cerebellar vessel. The clinical manifestations and resulting deficits are related to the specific vessels involved and the extent of collateral circulation. Main symptoms include headache, vertigo, dizziness, nausea, vomiting, unsteady gait, limb clumsiness, dysarthria, diplopia, and decreased level of alertness. Diagnosis relies on detailed attention to eye movements, coordination, and gait [42]. Most prominent signs include limb and gait ataxia, dysarthria, nystagmus, ocular tilt reaction [13] and altered mental status. Motor symptoms may be minimal or lacking, particularly among patients with infarcts involving lobule VI of the cerebellum [106]. According to their topographical distribution, four types of cerebellar infarction are recognized corresponding to the arterial territories of (a) the PICA (40%), (b) the AICA (5%), (c) the superior cerebellar artery (35%), and (d) the cortical watershed and deep cerebellar white matter borderzone infarcts (20%) [16,65,129]. Two distinct clinical syndromes are recognized: space-occupying cerebellar infarcts with fourth ventricular and brainstem compression and cerebellar infarcts without fourth ventricular or brainstem compression.
Space-occupying cerebellar infarcts likely to cause brainstem compression may present with sudden onset of occipital headache, vertigo, nausea, vomiting, gait unsteadiness, and dysarthria [4]. Patients often display gait and truncal ataxia, ipsilateral axial lateropulsion, or both, which usually prevent them from standing upright [4]. They may also exhibit nystagmus, ipsilateral limb dysmetria, dysarthria, and impaired consciousness [125]. The edematous cerebellum may compress the aqueduct of Sylvius or the fourth ventricle causing acute obstructive hydrocephalus, or may compress the brainstem resulting in increasing headaches, decreased level of alertness, and occasionally a head tilt [4,74].
Two distinct syndromes of cerebellar tissue herniation are recognized: descending or tonsillar herniation syndrome, and upward or transtentorial herniation syndrome. With a cerebellar pressure cone, there is downward displacement of the cerebellar tonsils through the foramen magnum causing compression of the medulla oblongata. Clinical manifestations of tonsillar herniation include neck stiffness, paresthesias in shoulders, opisthotonus, cardiac and respiratory rhythm disturbances, leading to apnea and possible death. With upward transtentorial herniation, there is upward displacement of the superior aspect of the cerebellar hemisphere through the free edge of the tentorial incisura, resulting in midbrain compression. Clinical manifestations of upward cerebellar herniation include lethargy, coma, paralysis or upward gaze, mid-position and unreactive pupils, and abnormal extensor posturing. Large cerebellar infarcts tend to involve the territory of the PICA, the territory of the superior cerebellar artery, or both [4,7]. Delayed alteration in the level of consciousness may occur hours to days after the onset of ischemic symptoms either in isolation or in association with the worsening of other neurologic signs [4,7]. Emergency surgery (e.g., ventriculostomy or posterior fossa decompression or both) is often required.
The other end of the clinical spectrum includes very small (border zone) cerebellar infarcts not easily localizable within well-defined arterial boundaries [8,65]. In a study of 47 patients, Amarenco et al. attributed the mechanisms of these small cerebellar infarctions to: global hypoperfusion secondary to cardiac arrest (4%), small or end (pial) artery disease due to intracranial atheroma or hypercoagulable states (20%), focal cerebellar hypoperfusion due to large artery vertebrobasilar occlusive disease (34%), or brain embolism (23%). In nine patients (19%), the mechanism of infarction was unknown. Physical findings were either absent or included a wide-based gait, lateropulsion, mild ipsilateral dysmetria, dysarthria, or dysdiadochokinesia [8]. Small (border zone) cerebellar infarcts can also mimic acute vestibular neuritis, including canal paresis [65]. Acute isolated hemiataxia is, in most cases, due to infratentorial (cerebellar) stroke. However, supratentorial stroke (e.g., thalamic infarction extending into the adjacent posterior limb of the internal capsule and infarction or hemorrhage restricted to the posterior limb of the internal capsule) may also cause isolated hemiataxia due to interruption of the cerebellar pathways at the level of the internal capsule [79].
Inferior Cerebellar Infarct (Posterior Inferior Cerebellar Artery)
Most symptomatic cerebellar infarctions occur in the territory supplied by the PICA. Infarcts stemming from occlusion in the territorial supply of the PICA are described in Chapter 22. Most often, infarcts in this arterial territory result from occlusion of the distal vertebral artery before the origin of the PICA. PICA or medial PICA territory infarcts cause acute vertigo and truncal ataxia, whereas lateral PICA territory infarcts cause unsteadiness, limb ataxia, and dysmetria without dysarthria. Unilateral limb ataxia without dysarthria or vestibular signs or symptoms suggests an infarct isolated to the territory of the lateral branch of the PICA [15]. Bilateral cerebellar infarctions in the territory of the medial branches of PICA may cause vertigo, dysarthria, disequilibrium, bilateral gaze evoked nystagmus, and gait ataxia without brainstem signs [122]. Vertigo and upside-down vision may also result from cerebellar flocculus and nodulus infarction due to involvement of the medial branch of PICA [27]. Rarely, patients may develop failure of automatic breathing when asleep (Ondine’s curse) [78]. Patients may also present with isolated vertigo resembling acute vestibular neuritis [72]. Cardiac embolism, atherothrombosis, and vertebral artery dissection are the main causes of large PICA territory infarcts [16].
Ventral Cerebellar Infarct (Anterior Inferior Cerebellar Artery)
Infarcts in the distribution of the AICA territory involve the lateral mid- and low-pontine region and the anterolateral part of the cerebellum, particularly the middle cerebellar peduncle, flocculus, and the anterior part of the cerebellar lobules, with the exception of the lobulus anterior [4]. Because of the variability of the AICA, not all syndromes caused by its occlusion are expected to be identical. The characteristic clinical picture of an AICA infarct consists of the following constellation of symptoms and signs [4–6,9,91,121,129]:
1. Prominent vertigo, nausea, vomiting, and nystagmus were noted (due to involvement of the vestibular nuclei).
2. Ipsilateral facial hypalgesia and thermoanesthesia, and corneal hypesthesia (due to involvement of the trigeminal spinal nucleus and tract). Facial sensation is spared at times because of the occasional occurrence of an independent vessel arising from the basilar artery supplying the spinal tract and nucleus of the trigeminal nerve.
3. Ipsilateral Horner syndrome (due to interruption of the descending pupillodilator oculosympathetic fibers in the lateral portion of the pons and medulla). Occasionally, there may also be a skew deviation [5].
4. Contralateral trunk and extremity hypalgesia and thermoanesthesia were noted (due to involvement of the lateral spinothalamic tract).
5. Ipsilateral ataxia and asynergia were noted (due to involvement of the middle cerebellar peduncle and cerebellum).
6. Ipsilateral deafness and facial paralysis were noted (due to involvement of the lateral pontomedullary tegmentum).
The clinical manifestations of an AICA syndrome may at times be confused with those of the lateral medullary (Wallenberg) syndrome because of shared signs, such as dysmetria, vestibular signs, Horner syndrome, and facial sensory impairment with contralateral trunk and extremity pain, and temperature sensory loss [5]. However, facial palsy and deafness due to involvement of cranial nerves VII and VIII and their nuclei, lateral gaze palsy, and multimodal sensory impairment of the face should suggest an AICA syndrome [5].
Typically, infarctions in the AICA territory give rise to combined loss of both auditory and vestibular functions [73]. Moreover, labyrinthine infarction may be a precursor of impending pontocerebellar infarction in the AICA territory [66]. AICA territory infarcts may also present with isolated vestibular manifestations or isolated cerebellar signs [94,101]. Two patients have been described with clinical features of infarction in the distribution of the AICA who had isolated vertigo for several months before infarction [91]. Both patients had cerebrovascular risk factors and had experienced other episodes of transient neurologic symptoms not associated with vertigo. At the time of infarction, they developed vertigo, unilateral hearing loss, tinnitus, facial numbness, and hemiataxia. MRI revealed ischemic lesions in the lateral aspect of the pons and middle cerebellar peduncle. Because the blood supply to the inner ear and vestibulocochlear nerve arises from the AICA, the vertigo preceding the infarction may have resulted from transient ischemia to the inner ear structures or the vestibular nerve [91].
Dorsal Cerebellar Infarct (Superior Cerebellar Artery)
When the superior cerebellar artery (SupCA) is occluded at its origin, patients may have ipsilateral cerebellar signs, brainstem disturbances, and a contralateral dissociated sensory syndrome. In contrast, when there is an occlusion of peripheral branches of the SupCA, patients often present only with dysarthria and unsteady gait but without vertigo, brainstem signs, or contralateral dissociated sensory disturbances [124]. The “classic” presentation of SupCA territory infarction, as described by Mills, remains a rare occurrence [4,7,28,63,82,83,117,119,129]. When the classic syndrome occurs, the following may be found on examination:
1. Vertigo and vomiting (due to involvement of the vestibular nuclei and connections)
2. Nystagmus (due to involvement of the medial longitudinal fasciculus and cerebellar pathways)
3. Ipsilateral Horner syndrome (due to compromise of the descending oculosympathetic fibers)
4. Ipsilateral ataxia and asynergia (due to involvement of the superior cerebellar peduncle and cerebellum)
5. Ipsilateral intention tremor (due to involvement of the dentate nucleus and superior cerebellar peduncle)
6. Contralateral trunk and extremity hypalgesia and thermoanesthesia (due to involvement of the lateral spinothalamic and quintothalamic tracts)
7. Contralateral hearing impairment (due to involvement of the crossed fibers of the lateral lemniscus)
8. Contralateral fourth nerve palsy (due to involvement of the pontine tectum)
A SupCA distribution infarction may also cause contrapulsion of saccadic eye movements associated with ipsilateral limb ataxia [97]. This saccadic disorder has three elements: (a) horizontal saccades away from the lesion during attempted vertical saccades, resulting in oblique trajectories, (b) hypermetria of contralateral saccades, and (c) hypometria of ipsilateral saccades. This contrapulsion of saccades (lateropulsion contralateral to a cerebellar lesion) differs from the lateropulsion of saccades seen in the lateral medullary syndrome, which is directed to the side of the lesion and associated with hypermetria of saccades toward the brainstem lesion and hypometria of saccades away from the lesion (see Chapter 15). Contrapulsion of saccades has also been described in association with primary-position upbeat nystagmus in cases of hemispheric cerebellar lesions [19].
A study of 33 patients with SupCA territory infarction [7] showed frequent association with infarcts in the distribution of the distal basilar artery (73%), including the rostral brainstem, thalamosubthalamic region, and occipitotemporal lobes. Patients presented with altered level of consciousness, ocular motor abnormalities, signs of thalamic dysfunction, visual field defects, cortical blindness, and/or memory loss [4]. One-third also had infarcts in the distribution of the PICA, sometimes associated with infarcts in the distribution of the AICA. Clinical presentations included a rostral basilar artery syndrome; coma at onset, often with tetraplegia; or cerebellar-vestibular signs, often with delayed coma due to cerebellar swelling. However, a “classic” SupCA syndrome was unusual [5,7].
Infarction in the distribution of the lateral branch of the SupCA (anterior rostral cerebellar infarct) may present with ipsilateral dysmetria and axial lateropulsion, contrapulsion of saccades, dysarthria, unsteadiness, and vomiting [10,97]. A dysarthria–clumsy hand syndrome may also be the presenting feature [10]. Patients with anterior rostral cerebellar infarction usually improve without suffering major sequelae. Dysarthria is frequently seen with the involvement of the territory of the medial branch of the SupCA [5,71]. Selective involvement of the anterior cerebellar lobe in cases of medial SupCA territory infarction may cause mild appendicular dysmetria and spontaneous extensor posturing of the neck, trunk, and all four extremities [99].
Patients with infarcts in the territories of the PICA or the SupCA were studied to compare their clinical presentations, course, and prognosis [62]. In 36 patients with PICA territory infarcts, a triad of vertigo, headache, and gait imbalance predominated at stroke onset, and computed tomography showed signs of severe cerebellar mass effect (30%) with associated hydrocephalus and brainstem compression, resulting in four deaths. In 30 patients with SupCA infarcts, gait disturbances predominated at onset; vertigo and headache were significantly less common. Isolated vertigo resembling vestibular neuritis is seldom seen with infarcts in the SupCA territory [72]. The clinical course was usually benign, and computed tomography showed signs of cerebellar mass effect, hydrocephalus, and brainstem compression in only 7% of the cases. Presumed cardiac embolism or artery-to-artery embolism was the predominant stroke mechanism in patients with SupCA distribution infarcts, whereas in those with PICA distribution infarcts, the stroke mechanism was equally divided between those with cardiac sources of embolism and those with posterior circulation atherothrombotic occlusive disease. Thus, cerebellar infarcts in the PICA and SupCA distribution have distinct differences in clinical presentation, course, and prognosis [62].
References
1. Ackermann H, Vogel M, Petersen D, et al. Speech deficits in ischaemic cerebellar lesions. J Neurol 1992; 239:223–227.
2. Afifi AK, Bergman RA. Functional neuroanatomy. Text and atlas. Cerebellum. New York, NY: McGraw-Hill, 2000:303–329.
3. Amaral DG, Schumann CM, Nordahl CW. Neuroanatomy of autism. Trends Neurosci 2008;31: 137–145.
4. Amarenco P. The spectrum of cerebellar infarctions. Neurology 1991;41:973–979.
5. Amarenco P, Chevrie-Muller C, Roullet E, et al. Paravermal infarct and isolated cerebellar dysarthria. Ann Neurol 1991;30:211–213.
6. Amarenco P, Hauw JJ. Cerebellar infarction in the territory of the anterior and inferior cerebellar artery. A clinicopathological study of 20 cases. Brain 1990a; 113:139–155.
7. Amarenco P, Hauw JJ. Cerebellar infarction in the territory of the superior cerebellar artery: a clinicopathologic study of 33 cases. Neurology 1990b;40: 1383–1390.
8. Amarenco P, Kase CS, Rosengart A, et al. Very small (border zone) cerebellar infarcts. Distribution, causes, mechanisms and clinical features. Brain 1993;116: 161–186.
9. Amarenco P, Rosengart A, DeWitt LD, et al. Anterior inferior cerebellar artery territory infarcts. Mechanism and clinical features. Arch Neurol 1993;50:154–161.
10. Amarenco P, Roullet E, Goujon C, et al. Infarction in the anterior rostral cerebellum (the territory of the lateral branch of the superior cerebellar artery). Neurology 1991;41:253–258.
11. Andreasen NC, Pierson R. The role of the cerebellum in schizophrenia. Biol Psychiatry 2008;64:81–88.
12. Azevedo FA, Carvalho LR, Grinberg LT, et al. Equal numbers of neuronal and non-neuronal cells make the human brain an isometrically scaled-up primate brain. J Comp Neurol 2009;513:532–541.
13. Baier B, Dieterich M. Ocular tilt reaction: a clinical sign of cerebellar infarctions? Neurology 2009;72: 572–573.
14. Baldacara L, Borgio JGF, de Lacerda ALT, et al. Cerebellum and psychiatric disorders. Rev Bras Psyquiatr 2008;30(3):281–289.
15. Barth A, Bogousslavsky J, Regli F. Infarcts in the territory of the lateral branch of the posterior inferior cerebellar artery. J Neurol Neurosurg Psychiatry 1994;57: 1073–1076.
16. Barth A, Bogousslavsky J, Regli F. The clinical and topographic spectrum of cerebellar infarcts: a clinical-magnetic resonance imaging correlation study. Ann Neurol 1993;33:451–456.
17. Bastian AJ, Mink JW, Kaufman BA, et al. Posterior vermal split syndrome. Ann Neurol 1998;44:601–610.
18. Bauman ML. Neuropathology of autism. In: Joseph AB, Young RR, eds. Movement disorders in neurology and neuropsychiatry. Boston, MA: Blackwell Scientific, 1992:662–666.
19. Benjamin EE, Zimmerman CF, Troost T. Lateropulsion and upbeat nystagmus are manifestations of central vestibular dysfunction. Arch Neurol 1986;43:962– 964.
20. Benson DF. Aphasia, alexia, and agraphia. New York, NY: Churchill-Livingstone, 1979:163–164.
21. Brodal A. Cerebrocerebellar pathways. Anatomical data and some functional implications. Acta Neurol 1972;51(Suppl 51):153–195.
22. Brodal A. Neurologic anatomy in relation to clinical medicine, 3rd ed. New York, NY: Oxford University Press, 1981:294–298.
23. Brown JR. Diseases of the cerebellum. In: Baker AB, Baker LH, eds. Clinical neurology. Philadelphia, PA: Harper & Row, 1962;3:1406–1455.
24. Catsman-Berrevoets CE, Van Dongen HR, Mulder PGH, et al. Tumour type and size are high risk factors for the syndrome of “cerebellar” mutism and subsequent dysarthria. J Neurol Neurosurg Psychiatry 1999; 67:755–757.
25. Cavanagh JG, Holton JL, Nolan CC. Selective damage to the cerebellar vermis in chronic alcoholism: a contribution from neurotoxicology to an old problem of selective vulnerability. Neuropathol Appl Neurobiol 1997;23:355–363.
26. Cerqueira AC, Reis MC, Novis FD, et al. Cerebellar degeneration secondary to acute lithium carbonate intoxication. Arq Neuropsiquiatr 2008;66(3A): 578–580.
27. Charles N, Froment C, Rode G, et al. Vertigo and upside down vision due to an infarct in the territory of the medial branch of the posterior inferior cerebellar artery caused by a dissection of the vertebral artery. J Neurol Neurosurg Psychiatry 1992;55:188–192.
28. Chaves CJ, Pessin MS, Caplan LR, et al. Cerebellar hemorrhagic infarction. Neurology 1996;46:346–349.
29. Cogan DG. Ocular dysmetria: flutter-like oscillations of the eyes and opsoclonus. Arch Ophthalmol 1954; 51:318.
30. Cole M. Dysprosody due to posterior fossa lesions. Trans Am Neurol Assoc 1971;95:151.
31. Courchesne E, Yeung-Courchesne R, Press GA, et al. Hypoplasia of cerebellar vermal lobules VI and VII in autism. N Engl J Med 1988;318:1349–1354.
32. Crutchfield JS, Sawaga RS, Meyers CA, et al. Postoperative mutism in neurosurgery. J Neurosurg 1994;81: 115–121.
33. Cruz-Mariño T, González-Zaldivar Y, Laffita-Mesa JM, et al. Uncommon features in Cuban families affected with Friedreich ataxia. Neurosci Lett 2010; 472(2):85–89.
34. Daum I, Ackermann H. Cerebellar contributions to cognition. Behav Brain Res 1995;67:201–210.
35. DeMyer W. Neuroanatomy. 2nd ed. Baltimore, Maryland: Williams and Wilkins, 1998.
36. De Smet HJ, Baillieux H, Castman-Berrevoets C, et al. Postoperative motor speech production in children with the syndrome ‘cerebellar’ mutism and subsequent dysarthria: a critical review of the literature. Eur J Paediatr Neurol 2007;11(4):193–207.
37. De Smet HJ, Baillieux H, De Deyn PP, et al. The cerebellum and language: the story so far. Folio Phoniatr Logop 2007;59(4):165–170.
38. Diedricksen J, Balsters JH, Flavell J, et al. A probabilistic MR atlas of the human cerebellum. Neuroimage 2009;46(1):39–46.
39. Diehl B, Lee MS, Reid JR, et al. Atypical, perhaps under-recognized? An unusual phenotype of Friedreich ataxia. Neurogenetics 2010;11(2):261–265.
40. Durr A. Friedreich’s ataxia: treatment within reach. Lancet Neurol 2002;1:370–374.
41. Durr A, Cossee M, Agid Y, et al. Clinical and genetic abnormalities in patients with Friedreich’s ataxia. N Engl J Med 1996;335:1169–1175.
42. Edlow JA, Newman-Toker DE, Savitz SI. Diagnosis and initial management of cerebellar infarction. Lancet Neurol 2008;7(10):951–964.
43. Ellenberger C, Keltner JL, Stroud MH. Ocular dyskinesia in cerebellar disease. Brain 1972;95:685– 692.
44. Ersahin Y, Mutluer S, Cagli S, et al. Cerebellar mutism: report of seven cases and review of the literature. Neurosurgery 1996;38:60–66.
45. Fielding J, Corben L, Cremer P. Disruption to higher order processes in Friedreich ataxia. Neuropsychologia 2010;48(1):235–242.
46. Fogel BL, Perlman S. Clinical features and molecular genetics of autosomal recessive cerebellar ataxias. Lancet Neurol 2007;6:245–257.
47. Ghez C. The cerebellum. In: Kandel ER, Schwartz JH, Jessell TM, eds. Principles of neural science, 3rd ed. New York, NY: Elsevier, 1991:626–646.
48. Gilman S. Cerebellar control of movement. Ann Neurol 1994;35:3–4.
49. Gilman S. Cerebellum and motor dysfunction. In: Asbury AK, McKhann GM, McDonald WI, eds. Diseases of the nervous system. Clinical neurobiology, 2nd ed. Philadelphia, PA: Saunders, 1992:319–341.
50. Gilman S, Bloedel JR, Lechtenberg R. Disorders of the cerebellum. Philadelphia, PA: FA Davis Co, 1981.
51. Glaser JS. Neuro-ophthalmology. Hagerstown, MD: Harper & Row, 1978:212–213, 221–240.
52. Goldstein BH, Birk CL, Van Houten M, et al. Ovarian cancer and late onset paraneoplastic cerebellar degeneration. Arch Gynecol Obstet 2009;280(1): 99–101.
53. Gordon N. The cerebellum and cognition. Eur J Paediatr Neurol 2007;11(4):232–234.
54. Hadjivassilou M, Boscolo S, Tongiorgi E, et al. Cerebellar ataxia as a possible organ-specific autoimmune disease. Mov Disord 2008;23(10):1370–1377.
55. Harding AE. Hereditary ataxias and related disorders. In: Asbury AK, McKhann GM, McDonald WI, et al., eds. Diseases of the nervous system. Philadelphia, PA: WB Saunders, 1986:1229–1238.
56. Henry RJ, Harries-Jones, Balakrishnan VK. Gynaecology meets neurology: paraneoplastic cerebellar degeneration. Aust NZJ Obstet Gynaecol 2005;45(4): 342.
57. Hetter EJ, Kempler D, Jackson CA, et al. Cerebellar glucose metabolism in chronic aphasia. Neurology 1987;37:1599–1606.
58. Holmes G. The cerebellum of man. Brain 1939;62:1.
59. Holmes G. The clinical symptoms of cerebellar disease and their interpretation. Lancet 1922;1:1177.
60. Jen JC, Graves TD, Hess EJ, et al. CINCH investigators. Primary episodic ataxias: diagnosis, pathogenesis, and treatment. Brain 2007;130:2484–2493.
61. Karmon Y, Inbar E, Cordoba M, et al. Paraneoplastic cerebellar degeneration mimicking acute post-infectious cerebellitis. Cerebellum 2009;8(4):411– 444.
62. Kase CS, Norrving B, Levine SR, et al. Cerebellar infarction. Clinical and anatomic observations in 66 cases. Stroke 1993;24:76–83.
63. Kase CS, White JL, Joslyn JN, et al. Cerebellar infarction in the superior cerebellar artery distribution. Neurology 1981;35:705–711.
64. Kent R, Netrell R. A case study of an ataxic dysarthria: cineradiographic and spectrographic observations. J Speech Hear Disord 1975;40:115–134.
65. Kikuchi S, Yamasoba T. Neuro-otological findings in patients with very small (border zone) cerebellar infarcts. Acta Otolaryngol Suppl 2007;559:56–60.
66. Kim JS, Cho KH, Lee H. Isolated labyrinthine infarction is a harbinger of anterior inferior cerebellar artery territory infarction with normal diffusion-weighted brain MRI. J Neurol Sci 2009;278(1–2):82–84.
67. Klockgether T, Ludtke R, Kramer B, et al. The natural history of degenerative ataxia. A retrospective study in 466 patients. Brain 1998;121:589–600.
68. Konczak J, Timmann D. The effect of damage to the cerebellum on sensorimotor and cognitive function in children and adolescents. Neurosci Biobehav Rev 2007;31(8):1101–1113.
69. Larochelle L, Bedard P, Boucher R, et al. The rubro-olivo-cerebello-rubral loop and postural tremor in the monkey. J Neurol Sci 1970;11:53–1164.
70. Lauterbauch EC. Bipolar disorders, dystonia, and compulsion after dysfunction of the cerebellum, dentatorubrothalamic tract, and substantia nigra. Biol Psychiatry 1996;40:726–730.
71. Lechtenberg R, Gilman S. Speech disorders in cerebellar disease. Ann Neurol 1978;3:285–290.
72. Lee H, Kim JS, Chung EJ, et al. Infarction in the territory of anterior inferior cerebellar artery: spectrum of audiovestibular loss. Stroke 2009;40(12):3745–3751.
73. Lee H, Sohn S-I, Cho Y-W, et al. Cerebellar infarction presenting isolated vertigo: frequency and vascular topographical patterns. Neurology 2006;67:1178– 1183.
74. Lehrich JR, Winkler GF, Ojemann RG. Cerebellar infarction with brainstem compression. Arch Neurol 1970;22:490–498.
75. Leigh RJ, Zee DS. The neurology of eye movements, 3rd ed. New York, NY: Oxford University Press, 1999.
76. Leiner HC, Leiner AL, Dow RS. Cognitive and language functions of the human cerebellum. Trends Neurosci 1993;16:444–448.
77. Leiner HC, Leiner AL, Dow RS. The human cerebro-cerebellar system: its computing, cognitive and language skills. Behav Brain Res 1991;44(2):113–128.
78. Levin B, Margolis CT. Acute failure of automatic respirations secondary to a unilateral brain stem infarct. Ann Neurol 1977;1:583–586.
79. Luijckx GJ, Boiten J, Lodder J, et al. Isolated hemiataxia after supratentorial brain infarction. J Neurol Neurosurg Psychiatry 1994;57:742–746.
80. Macklis RM, Macklis JD. Historical and phrenologic reflections of the non-motor functions of the cerebellum: love under the tent? Neurology 1992;42:928– 932.
81. Middleton FA, Strick PL. Anatomical evidence for cerebellar and basal ganglia involvement in higher cognitive function. Science 1994;266:458–461.
82. Mills CK. Hemianesthesia to pain and temperature and loss of emotional expression on the right side, with ataxia of the upper limb on the left: the symptoms probably due to a lesion of the thalamus or superior peduncles. J Nerv Ment Dis 1908;35:331–332.
83. Mills CK. Preliminary note on a new symptom complex due to a lesion of the cerebellum and cerebello-rubro-thalamic system, the main symptoms being ataxia of the upper and lower extremities of one side, and on the other side deafness, paralysis of emotional expression in the face, and loss of the senses of pain, heat and cold over the entire half of the body. J Nerv Ment Dis 1912;39:73–76.
84. Mittelbronn N, Schittenhelm J, Bakos G, et al. CD8(+)/perforin/granzyme B(+) effector cells in filtrating cerebellum and inferior olives in gluten ataxia. Neuropathology 2010;30(1):92–96.
85. Moon JS, Kim G, Koo YH, et al. Kinetic tremor and cerebellar ataxia as initial manifestations of Kikuchi-Fujimoto’s disease. J Neurol Sci 2009;277(1–2):181– 183.
86. Morrison PJ. Paediatric and adult autosomal dominant ataxias (update 6). Eur J Paediatr Neurol 2010; 14:261–263.
87. Mosca Al, Laurent N, Guibaud L, et al. Polymicrogyria, cerebellar-vermis hypoplasia, severe facial dysmorphism and cleft palate: a new syndrome. Eur J Med Genet 2007;50(1):48–53.
88. Naito H, Oyanagi K. Familial myoclonus epilepsy and choreoathetosis: hereditary dentatorubral pallidoluysian atrophy. Neurology 1982;32:789–817.
89. Nicholson RI, Fawcett AJ, Dean P. Developmental dyslexia: the cerebellar deficit hypothesis. Trends Neurosci 2001;24:508–511.
90. Nitschke MF, Kleinschmidt A, Wessel K, et al. Somatotopic motor representation in the human anterior cerebellum. A high resolution functional MRI study. Brain 1996;119:1023–1029.
91. Oas JG, Baloh RW. Vertigo and the anterior inferior cerebellar artery syndrome. Neurology 1992;42:2274– 2279.
92. Parvizi J, Anderson SW, Martin CO, et al. Pathological laughter and crying. A link to the cerebellum. Brain 2001;124(9):1708–1719.
93. Pernet CR, Poline JB, Demonet JF, et al. Brain classification reveals the right cerebellum as the best biomarker of dyslexia. BMC Neurosci 2009;25:10–67.
94. Philips PC, Lorentsen KJ, Shropshire LC, et al. Congenital odontoid aplasia and posterior circulation stroke in childhood. Ann Neurol 1988;23:410–413.
95. Pollack IF. Posterior fossa syndrome. Int Rev Neurobiol 1997;41:411–432.
96. Pollack IF, Polinko P, Albright AL, et al. Mutism and pseudobulbar symptoms after resection of posterior fossa tumors in children: incidence and pathophysiology. Neurosurgery 1995;37:885–893.
97. Ranalli PJ, Sharpe JA. Contrapulsion of saccades and ipsilateral ataxia: a unilateral disorder of the rostral cerebellum. Ann Neurol 1986;20:311–316.
98. Rekate HL, Grubb RL, Aram DM, et al. Muteness of cerebellar origin. Arch Neurol 1985;42:697–698.
99. Ringel RA, Culberson JL. Extensor tone disinhibition from an infarction within the midline anterior cerebellar lobe. J Neurol Neurosurg Psychiatry 1988;51: 1597–1599.
100. Ross ED, Mesulam M. Dominant language functions of the right hemisphere? Prosody and emotional gesturing. Arch Neurol 1979;36:144–148.
101. Rubenstein RL, Norman DM, Schindler RA, et al. Cerebellar infarction: a presentation of vertigo. Laryngoscope 1980;90:505–514.
102. Sabater L, Bataller L, Suávez-Calvet M, et al. ZIC antibodies in paraneoplastic cerebellar degeneration and small cell lung cancer. J Neuroimmunol 2008; 201–202:163–165.
103. Sacchetti B, Scelfo B, Strata P. Cerebellum and emotional behavior. Neuroscience 2009;162:756–762.
104. Salam M. Metabolic ataxias. In: Vinken PJ, Bruyn GW, et al., eds. Handbook of clinical neurology. New York, NY: American Elsevier, 1975:573–585.
105. Savoiardo M, Bracchi M, Passerini A, et al. The vascular territories in the cerebellum and brainstem: CT and MR study. Am J Neuroradiol 1987;8:199–209.
106. Schmahmann JD, Macmore J, Vangel M. Cerebellar stroke without motor deficit: clinical evidence for motor and non-motor domains within the human cerebellum. Neuroscience 2009;162(3):852–861.
107. Schmahmann JD, Sherman JC. Cerebellar cognitive affective syndrome. Int Rev Neurobiol 1997;41:433–440.
108. Schmahmann JD, Sherman JC. The cerebellar cognitive affective syndrome. Brain 1998;121:561–579.
109. Schols L, Bauer P, Schmidt T, et al. Autosomal dominant cerebellar ataxias: clinical features, genetics, and pathogenesis. Lancet Neurol 2004;3:291–304.
110. Schols L, Peters S, Szymanski S, et al. Extrapyramidal motor signs in degenerative ataxias. Arch Neurol 2000;57:1495–1500.
111. Selhorst JB, Stark L, Ochs AL, et al. Disorders in cerebellar ocular motor control. I. Saccadic overshoot dysmetria. An oculographic, control system, and clinicoanatomical analysis. Brain 1976a;99:497–508.
112. Selhorst JB, Stark L, Ochs AL, et al. Disorders in cerebellar ocular motor control. II. Macrosaccadic oscillation: an oculographic control system, and clinico-anatomical analysis. Brain 1976b;99:509–522.
113. Sens PM, de Almeida CI. Participation of the cerebellum in auditory processing. Braz J Otorhinolaryngol 2007;73(2):266–270.
114. Sillitoe RV, Joyner AL. Morphology, molecular codes, and circuitry produce the three-dimensional complexity of the cerebellum. Annu Rev Cell Dev Biol 2007;23:549–577.
115. Silveri MC, Leggio MG, Molinari M. The cerebellum contributes to linguistic production: a case of agrammatic speech following a right cerebellar lesion. Neurology 1994;44:2047–2050.
116. Silveri MC, Misciagna S, Leggio MG, et al. Spatial dysgraphia and cerebellar lesion: a case report. Neurology 1997;48:1529–1532.
117. Simmons Z, Biller J, Adams HP Jr, et al. Cerebellar infarction: comparison of computed tomography and magnetic resonance imaging. Ann Neurol 1986;19: 291–293.
118. Stolze H, Klebe S, Petersen G, et al. Typical features of cerebellar ataxic gait. J Neurol Neurosurg Psychiatry 2002;73:310–312.
119. Struck LK, Biller J, Bruno A, et al. Superior cerebellar artery territory infarction. Cerebrovasc Dis 1991;1:71–75.
120. Subramoni SH. Degenerative ataxias. Curr Opin Neurol Neurosurg 1995;7:316–322.
121. Sypert GW, Alvord EC Jr. Cerebellar infarction: a clinicopathological study. Arch Neurol 1975;32:357–363.
122. Tada Y, Mizutani T, Nishimura T, et al. Acute bilateral cerebellar infarction in the territory of the medial branches of posterior inferior cerebellar arteries. Stroke 1994;25:686.
123. Ten Donkelaar HJ, Lammens M. Development of the human cerebellum and its disorders. Clin Perinatol 2009:36(3)513–520.
124. Terao S, Sobue G, Izumi M, et al. Infarction of superior cerebellar artery presenting as cerebellar symptoms. Stroke 1996;27:1679–1681.
125. Tettenborn B, Caplan LR, Sloan MA, et al. Postoperative brainstem and cerebellar infarcts. Neurology 1993;43:471–477.
126. Thach WT, Goodkin HP, Keating JG. The Cerebellum and the Adaptive Coordination of Movements. Ann Rev Neurosci. 1992;15:403–442.
127. Tilikete C, Pélisson D. Ocular motor syndromes of the brainstem and cerebellum. Curr Opin Neurol 2008;21(1):22–28.
128. Timmann D, Brandauler B, Hermsdörfer J, et al. Lesion-symptom mapping of the human cerebellum. Cerebellum 2008;7(4):602–606.
129. Tohgi H, Takahashi S, Chiba K, et al. Cerebellar infarction. Clinical and neuroimaging analysis in 293 patients. Stroke 1993;24:1697–1701.
130. Toledo MM, Garcia LI, Coria-Avila GA, et al. Why should we keep the cerebellum in mind when thinking about addiction? Curr Drug Abuse Rev 2009;2(1): 24–40.
131. Turner BM, Paradiso S, Marvel CL, et al. The cerebellum and emotional experience. Neuropsychologia 2007;25:45(6):1331–1341.
132. Urban PP, Marx J, Hunsche SD, et al. Cerebellar speech representation: lesion topography in dysarthria as derived from cerebellar ischemia and functional magnetic resonance imaging. Arch Neurol 2003;60: 965–972.
133. Van Calenbergh F, Van De Laar A, Plets C, et al. Transient cerebellar mutism after posterior fossa surgery in children. Neurosurgery 1995;37:894–898.
134. Van Dongen HR, Catsman-Berrevoets CE, van Mourik M. The syndrome of ‘cerebellar’ mutism and subsequent dysarthria. Neurology 1994;44:2040– 2046.
135. Victor M, Adams RD, Mancall EL. A restricted form of cerebellar cortical degeneration occurring in alcoholic patients. Arch Neurol 1959;1:579.
136. Victor M, Ferrendelli JA. The nutritional and metabolic diseases of the cerebellum: clinical and pathological aspects. In: Fields WS, Willis WD, eds. The cerebellum in health and disease. St. Louis, MO: Green, 1970:412–449.
137. Vlachos F, Papthanasiou I, Andreou G. Cerebellum and reading. Folio Phoniatr Logop 2007;59(4):177– 183.
138. Walter N, Joanette Y. The unnoticed contributions of the cerebellum to language. Folio Phoniatr Logop 2007;59(4):171–176.
139. Weisenburg TH. Cerebellar localization and its symptomatology. Brain 1927;50:357.
140. Yokota O, Tsuchiya K, Terada S, et al. Alcoholic cerebellar degeneration: a clinicopathological study of six Japanese autopsy cases and proposed potential progression pattern in the cerebellar lesion. Neuropathology 2007;27(2):99–113.
141. Zinni G, Bertiani E, Bellcross C, et al. X-linked congenital ataxia: a new locus maps to Xq25-q27.1. Am J Med Genet A 2008;146A(5):593–600.
< div class='tao-gold-member'>
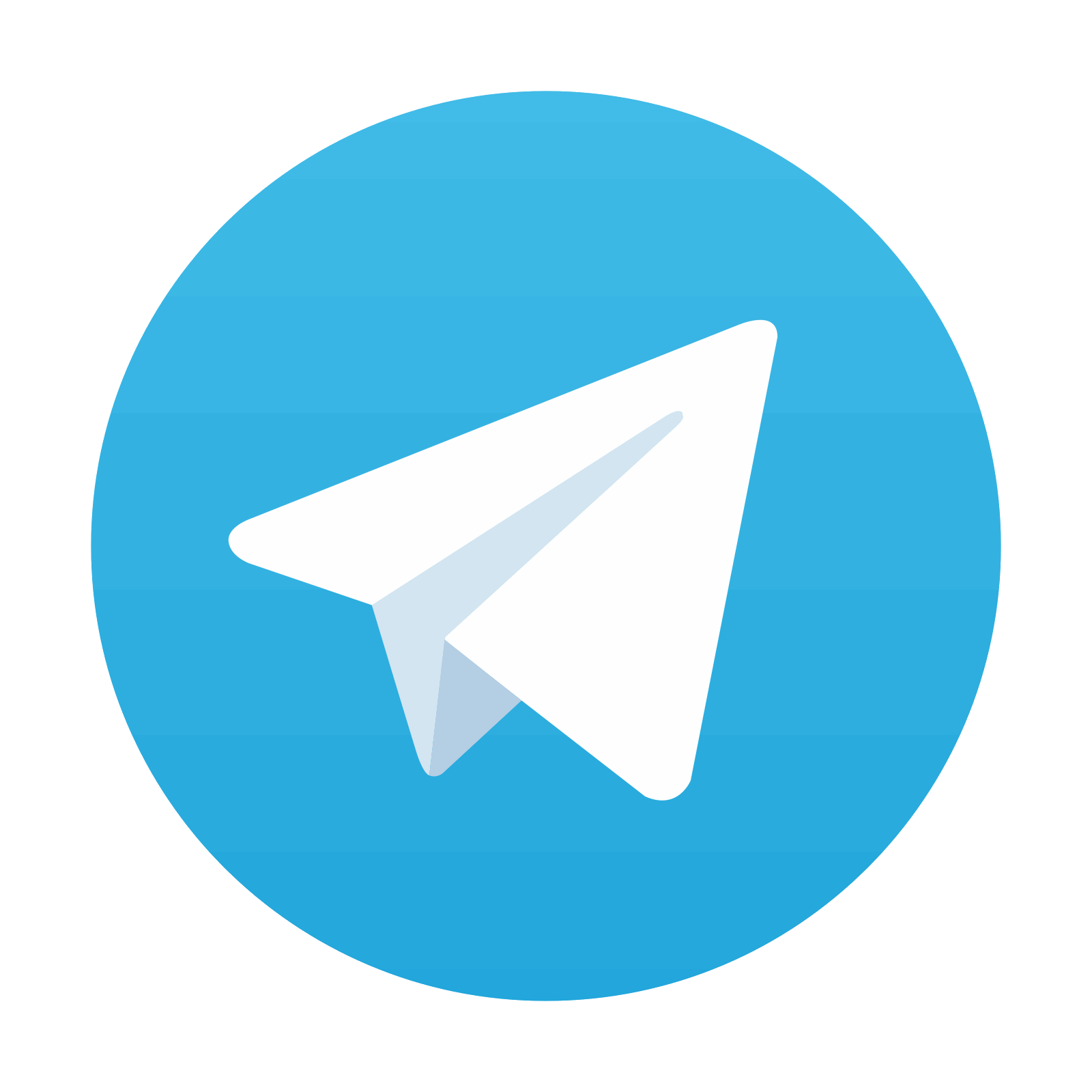