(1)
Facultad de Ciencias Médicas, Pontificia Universidad Católica Argentina, Buenos Aires, Capital Federal, Argentina
Abstract
The traditional view of the autonomic nervous system (ANS) considers only its peripheral part, the sympathetic and parasympathetic systems, to which sometimes the enteric ANS is added as an independent entity. However, this view is insufficient to understand the most important function of the ANS: the maintenance of homeostasis. This Chapter describes the hierarchical organization of the autonomic motor pathways and their similarities with the somatic motor organization. It analyzes the types of neurons, neuronal circuits, and electric potentials identified in autonomic neurons.
Keywords
AcetylcholineAutonomic postureBasic neuronal circuitsCircadian clockFight or flight reactionGolgi I and Golgi II neuronsHierarchical organization of the ANSHomeostasisNorepinephrineSomatic and visceral motor neuronsObjectives
After studying this chapter, you should be able to:
Describe the control of reactive and predictive homeostasis as the most important function of the ANS
Describe the hierarchical organization of the autonomic and somatic motor pathways and their similarities
Name the components of the autonomic posture in comparison with the mechanisms of body posture
Describe the two types of neurons and the three neuronal circuits that give rise to the basic neuronal organization of ANS
Name the different types of electrical potentials identified in autonomic neurons
The Control of Homeostasis Is the Most Important Function of the ANS
The traditional view of the autonomic nervous system (ANS) considers only its peripheral part, the sympathetic and parasympathetic systems, to which sometimes the enteric ANS is added as an independent entity. However, this view is insufficient to understand the most important function of the ANS: the maintenance of homeostasis.
The term “homeostasis” was coined by the distinguished American physiologist Walter B. Cannon as a comprehensive concept to describe the physiological factors that maintain the equilibrium state of the body, and therefore, life [1]. Following Claude Bernard, Cannon perfected the idea of the constancy of the internal environment, giving it its present meaning. As explained by Cannon, he chose as a prefix homeo- (“like”) instead of homo- (“the same”) to take into account the normal variations that any physiological variable exhibits, thus avoiding the misleading consideration of a “constant (fixed) internal milieu.”
The development of chronobiology as an independent discipline added another aspect to homeostasis. This term is used today to define not only the strategies that allow the organism’s proper response to changes in the environment (“reactive homeostasis”), but also the time-related mechanisms, remarkably developed, that allow the body to predict the occurrence of a given environmental stimulus to initiate the appropriate corrective responses beforehand (“predictive homeostasis”) [2]. Predictive homeostasis comprises the anticipatory mechanisms that precede a regularly occurring environmental phenomenon, thus facilitating a better physiological adaptation to it. For example, the increase in plasma cortisol that precedes waking anticipates the energy demands of a changing posture; the increased gastrointestinal secretion preceding the usual lunch time anticipates the changes in the content of the digestive tract.
Let us suppose that a diurnally active mammal in search of food finds the food at a place about 2 h from the shelter it uses to escape its predator at night. This situation makes it essential for the animal to predict nightfall about 2 h in advance. It do this using the position of the sun or other environmental variables, such as temperature or humidity. However, the existence of clouds or a large daily variation in ambient temperature make the value of such reference parameters unreliable. It is then extremely useful for survival to possess a system of time control integrated into its own organism that allows a temporal prediction without having to depend on the reading of external signals. This was probably the strategy selected as an advantage by evolution.
The circadian clock is ideal to fulfill such a function: we could have a sufficiently accurate idea of the time of day by simply analyzing our biological structure periodically and without consulting our wristwatch. That is, a “day” and “night” have been created within the organism to allow it to optimize adaptation [3].
Generally, the nervous system can produce a small number of actions: (a) the contraction of a muscle or of a group of striated or smooth muscles; (b) the exocrine, endocrine, or paracrine secretion of a cell or cell group; (c) changes in cellular permeability. This is achieved via motor neurons , which can be classified per their targets into three categories:
Somatic motor neurons , which originate in the central nervous system (CNS), project their axons to the skeletal muscles (such as the muscles of the limbs, abdominal, and intercostal muscles) that are involved in locomotion.
Special visceral motor neurons , also called branchial motor neurons , which directly innervate the branchial muscles (that motorize the gills in fish and the face and neck in land vertebrates).
Visceral preganglionic neurons , which indirectly innervate the heart, the smooth muscle in all organs, the abdominal viscera, the exocrine and endocrine glands, and the immune system. They synapse onto neurons located in the autonomic ganglia of the ANS (sympathetic and parasympathetic postganglionic neurons).
As a consequence: (a) the motor command of skeletal and branchial muscles is monosynaptic (involving only one motor neuron, somatic and branchial respectively, which synapses onto the muscle); (b) the command of the viscera is disynaptic, involving two neurons, the visceral preganglionic neuron located in the CNS, which synapses onto a postganglionic neuron, which synapses onto the organ.
The ANS, which is in charge of the innervation of smooth muscles of all organs and innervation of the viscera, immune tissue, and exocrine and endocrine glands, can produce the three actions mentioned above (smooth muscle contraction; exocrine, endocrine, or paracrine secretion; permeability changes). It must be noted that for the command of visceral muscles, the ganglionic neuron, parasympathetic or sympathetic, is the real motor neuron, as it is the one that directly innervates the muscle, whereas the general visceral motor neuron is, strictly speaking, the preganglionic one [4].
All vertebrate motor neurons are cholinergic, that is, they release the neurotransmitter acetylcholine (ACh). Parasympathetic ganglionic neurons are also cholinergic, whereas most sympathetic ganglionic neurons are noradrenergic, that is, they release the neurotransmitter norepinephrine (NE) .
It is not unusual to hear or read or to remain implicit that actions of the sympathetic and parasympathetic divisions promote opposite effects in the organs they innervate. However, for the precise aim of a homeostatic response requiring effectiveness, energy economy, and sometimes a fast response, the sympathetic and parasympathetic neurons must collaborate for the final net effect.
In general, the sympathetic activity is designed to place the individual in a situation of defense in the face of danger, real or potential. Sympathetic stimulation leads to variations in visceral functions designed to protect the integrity of the organism and to ensure survival. In fact, a sympathectomized animal hardly survives if left free and exposed in its natural environment. However, in addition to calm and resting states, our daily life involves many perturbations that induce active conditions such as locomotion, eating, and communication. Thus, the mobilization of physiological variables does not occur in an all-or-nothing manner and is not exclusive of extreme “fight or flight” conditions; rather, they can occur with graded intensities in ordinary daily situations. During such active periods, cardiovascular, respiratory, and body temperature regulation needs to be adjusted to situational demands, which differ from those during resting states, by modulating or resetting homeostatic points. For instance, if an increase in blood pressure (BP) is needed, a greater gain in the response can be obtained by the concomitant enhancement of sympathetic activity and inhibition of parasympathetic activity. This is obtained not only in the CNS areas, but also via reciprocal inhibitory connections at the level of the heart wall [5].
Autonomic nervous system activity adapts to body changes and supports somatic reactions. The autonomic response may be secondary to the somatic, parallel to, or, most commonly, before (the “autonomic posture”) [3]. The ANS is designed to produce sustained actions, compared with those of the somatic nervous system. On the other hand, the ANS performs this multisystem control in a continuous and constant manner throughout the life cycles, both at rest and during activity in the three body configurations within a 24 h cycle discussed in Chap. 2.
One of the most surprising features of the ANS is the speed and intensity with which it modifies the visceral functions. In a few seconds, for example, the heart rate and BP can increase up to double the normal rates, there may be intense sweating, the urinary bladder may empty, or digestive motility and secretion may be activated.
The sympathetic stimulation causes general responses in the organism for two reasons. First, there is a high degree of irradiation of the connections between preganglionic and postganglionic neurons. The proportion of preganglionic fibers to postganglionic neurons in sympathetic ganglia is 1/4–1/30, and since the degree of axonal branching is high, it is estimated that each preganglionic axon contacts an average of 120 neurons. For this reason, the activity of a few preganglionic neurons is highly amplified numerically via their ganglionic connections. Second, sympathetic activity produces a stimulation of the secretion of catecholamines from the adrenal medulla. In circulation, almost all epinephrine (E) found is derived from the adrenal medulla, but only 20% of the NE is. The rest of the circulating NE comes from the peripheral sympathetic terminals, i.e., as the neurotransmitter that has escaped presynaptic reuptake.
A massive activation of the sympathetic system produces a set of reactions defined as an alarm response (fight or flight) [6]. The most obvious visceral phenomena of this massive activation are:
Pupil dilation, to increase the visual field
Piloerection, to simulate a larger body size
Sweating, to lose heat that is produced by muscular activity
Increased cardiac activity and BP, to provide greater blood flow to the muscles
Bronchodilation, to increase the entrance of air into the lungs
Hyperglycemia
Inhibition of the digestive function
Inhibition of the urinary and genital functions
In contrast, the activity of the parasympathetic system is related to protective and conservation functions, which favor the proper functioning of the different visceral organs. The functional components of the parasympathetic system do not act simultaneously under normal conditions, but participate in specific reflexes or in integrated reactions to promote a specific visceral function. Thus, stimulation of different nuclei of parasympatheti c neurons promotes responses such as:
Pupillary constriction, to protect the retina from excessive illumination
Decreased heart rate, to avoid excessive activity
Bronchoconstriction, to protect the lungs
Increased motility and digestive secretions, to promote digestion
Urinary activity and urination
Genital activity (erection)
Therefore, the parasympathetic effects are, in consonance with their fundamental purpose, more localized. In the parasympathetic ganglia, each preganglionic neuron contacts a few postganglionary neurons, whose divergence is much smaller than that of the sympathetic system [6].
Both efferent systems exert a control of the function of the visceral organs in variable forms. Some effector organs receive innervation from a single system. For example, the smooth muscle of most blood vessels is only controlled by sympathetic vasoconstrictor innervation (except for vessels of genital organs, which also receive parasympathetic innervation, and cholinergic vasodilator fibers in the skeletal muscles and brain). In these cases, control of the visceral activity depends on the variations of the frequency of discharge of the impulses by sympathetic innervation. As the autonomic nerves have a basal tonic activity (of low pulse frequencies, 0.5–5 Hz), the activity may increase or decrease. In most vessels, an increase in sympathetic frequency determines vasoconstriction and a decrease, vasodilation [7].
Sympathetic and parasympathetic nerve fibers simultaneously innervate many effector organs. The activity of the organ depends on the interaction or balance between the signals of both systems, which exert antagonistic effects. This interaction can be developed by opposite actions on the same effector cells, as in the heart, where the sympathetic excites the nodal cells thus increasing the heart rate, whereas the parasympathetic inhibits the same cells, thus reducing their frequency.
Another possibility is the action on different cells, with opposite effects. In the iris, the sympathetic excites the meridian muscular fibers, which produces mydriasis, whereas the parasympathetic excites the circular fibers and produces miosis. In these cases, the functional balance is relatively complex. In normal circumstances, the two systems are reciprocally active, because the central and reflex signals excite one system and inhibit the other. When both sympathetic and parasympathetic systems innervate the same target cells, more complex interactions arise. For example, the simultaneous activation of sympathetic fibers may exaggerate the cardiovascular response to parasympathetic activity and vice versa. This phenomenon is mediated by mutual influences at the presynaptic and postsynaptic domains [7].
There Are Functional Similarities in the Hierarchical Organization of the Autonomic and Somatic Motor Pathways
To understand the hierarchical organization of the ANS, several concepts derived from the somatic motor system are useful [3]. Any movement, even the simplest one, involves an enormous amount of information processing and the participation of numerous neuronal groups. On the other hand, each movement of our body is based on a posture; thus, it is important to consider how the motor system provides global responses for both components, the posture needed, and the movement itself.
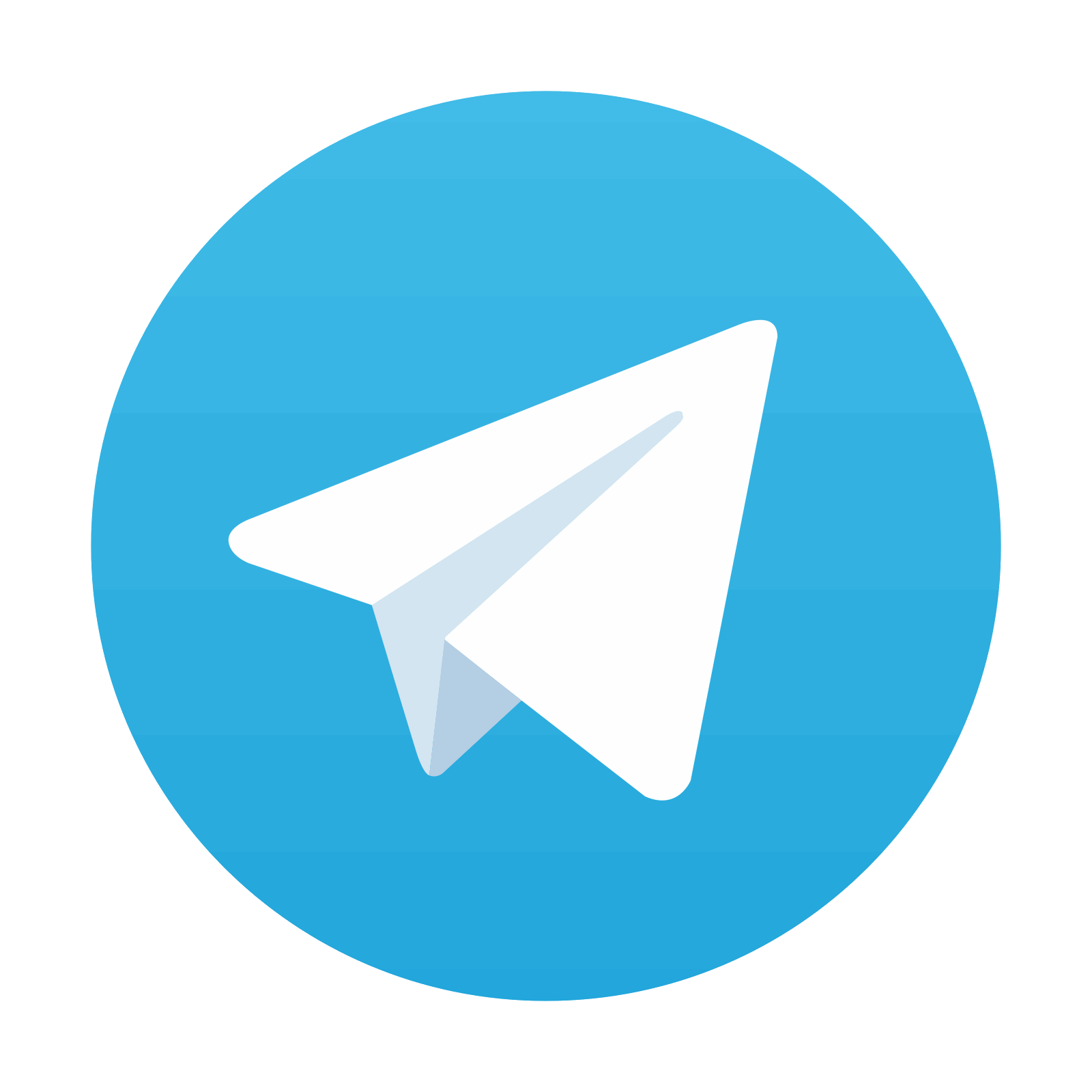
Stay updated, free articles. Join our Telegram channel

Full access? Get Clinical Tree
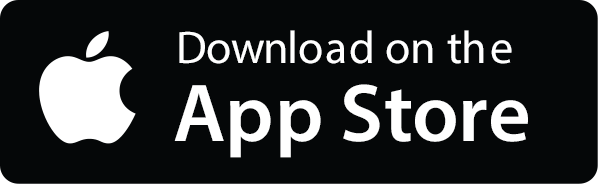
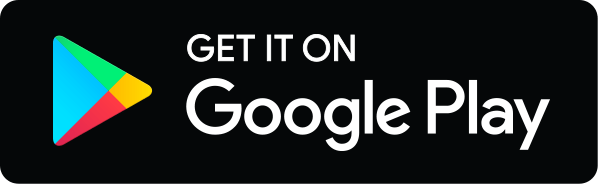