The Evolution of Evolutionary Neuroscience
Suzana Herculano‐Houzel
2.1 The Evolution of “Evolution”
The history of evolution is as long as the history of the Earth—and yet, “evolution” hasn’t always been there. Before “evolution,” naturalists framed their thoughts on the assumption of a fixed scala naturae as conceived by Aristotle: a strict hierarchical structure of all that is, descending from God down to minerals (“the great chain of being”; Lovejoy, 1964), with animals arranged in between “according to the degree of perfection of their souls” (Bunnin & Yu, 2004).
Once it appeared, the concept of evolution itself evolved—that is, changed over time—and along with it have evolved the questions and interpretations posed by neuroscientists. In the 19th century, the uncovering of growing numbers of particular fossils in different geological strata led to the concept of the mutability over time of the panoply of beings that had lived, and evolution came to be conceptualized by Charles Darwin (Darwin, 1859).
In the light of evolution, the scala naturae became a phylogenetic scale that organisms supposedly ascended as they evolved, over time, from simple to complex. Thus reasoned Ludwig Edinger, by many considered the father of comparative neuroanatomy, when he formulated at the end of the 19th century a unified theory of brain evolution that combined Charles Darwin’s 1859 concept of evolution with the then current version of Aristotle’s scala naturae. Edinger viewed evolution as progressive and linear: from fish to amphibians, reptiles, birds, then mammals—culminating with humans, naturally, in an ascent from “lower” to “higher” intelligence. In the process, the brains of extant vertebrates supposedly retained ancestral structures; for that reason, and in the face of progressive evolution, the comparison of the brain anatomy of extant species would reveal the origin of more recent structures. This supposed evidence of “past lives” in modern brain structures resonated with the Law of Recapitulation proposed by Étienne Serres, supported by Étienne Geoffroy Saint‐Hilaire (1830), and formulated by Ernst Haeckel in the aphorism “ontogeny recapitulates phylogeny” (1866). Haeckel claimed that the development of more recent (“advanced”) species passes through successive stages represented by adult forms of older (more “primitive”) species.
Recapitulation was refuted both at the level of embryogenesis and of brain evolution in the 20th century (reviewed in Gould, 1977). Darwin himself acknowledged that early embryonic stages may be similar across related species, but are not similar to the adult forms of those species—a view that is shared by modern evolutionary developmental biology (see below). In 1922, Walter Garstang advanced the idea that differences among adult animal species arise because of evolutionary modifications in their development program—that is, that phylogeny occurs through changes in ontogeny. This amounted to the exact opposite of what Haeckel had advocated in 1866. More recent evidence against recapitulation was the recognition that mammals and birds/reptiles are sister groups—that is, that mammals don’t derive from reptiles as they are today, just as humans don’t derive from modern monkeys, and also that the last common ancestral form to all mammals was not a reptile (Carroll, 1988). Rather, mammals arose from ancestral therapsids, while reptiles (including the later birds) arose from ancestral sauropsids, and both therapsids and sauropsids were sister branches of the ancestral stem amniote (see Figure 2.1; see also Carroll, 1988; Evans, 2000). The notion of an ancestral “reptilian” brain has been hard to shake off, however (see below).

Figure 2.1 Phylogeny of Tetrapods.
Phylogeny of tetrapods places modern reptiles (including birds), the living descendants of sauropsids, as a sister group to modern mammals, the living descendants of therapsids.
Modern evolutionary biologists also realize that phylogenetic trees are actually not trees, much less ladders, but rather erratic bushes with branches growing here and there in divergent directions (Gould, 1989), only some of which last through the ages. Edinger’s phylogenetic scale also fails in the face of secondary simplification: the fact that species do not always “progress” into more complex beings in evolution (Jenner, 2004). Regardless, however, scala naturae thinking persisted in the neurosciences (Hodos and Campbell, 1969), reflecting a lack of proper training in evolutionary biology (Striedter, 2009).
Other inversions in the evolutionary tree have been driven by discoveries made by molecular phylogenetics: the use of differences and similarities in the coding and noncoding sequences of particular genes to establish likely evolutionary relationships amongst them. Hence, the categorization of modern animals went from division into acoelomates, pseudocoelomates, and coelomates to, instead, division of Bilateria into protostomes (Lophotrochozoa and Ecdysozoa) and deuterostomes (echinoderms, hemichordates, urochordates, cephalochordates, and chordates) (Halanych et al., 1995; Hervé, Lartillot, & Brinkmann, 2005). The modern grouping is based on phylogenetic relationships unsuspected from simple morphological studies, upturning several popular theories on the evolution of the nervous system, and leads to the proposition of the Urbilateria as an ancestral group (De Robertis and Sasai, 1996).
Now disabused of the idea that ontogeny recapitulates phylogeny, modern evo‐devo (evolutionary developmental biology) views animal evolution as the result of changes in the developmental process, as envisioned by Garstang (1922). The study of comparative neurobiology thus still fuels the modern search for the origins of nervous system diversity—no longer through the search for successive, progressive steps in development, but, instead, by looking for those evolved modifications in development that gave rise to different adult life forms.
2.2 Evolution of “the Nervous System”
The nervous system is a characteristic of animals—although not of all animals, as Placozoa and sponges lack any semblance of a nervous system. Cnidaria and Ctenophora have a distributed network of nerve cells throughout their bodies. It is only in bilaterians that the nervous system assumes a cord‐like structure, although one that is arranged differently in protostomes and deuterostomes: It is situated ventrally in the former, and dorsally in the latter, raising the issue of how the nervous system was arranged in the common ancestor of bilaterians. One popular view was that this ancestor had a ventral nerve cord which became inverted in deuterostomes, as proposed by Geoffroy Saint‐Hilaire and by Anton Dorhn (Gerhart, 2000)—a view in line with the mistaken but popular concept of progressive phylogenesis, in which the “simpler” protostomes arose before deuterostomes instead of simultaneously. The remaining—and then apparently less likely—possibility was that a nerve cord was formed twice independently: once (ventrally) in protostomes and again (dorsally) in deuterostomes (see Figure 2.2).

Figure 2.2 Organization of Nervous Systems.
Amongst animals, only Cnidaria, Protostomia, and Deuterostomia have a nervous system, which is organized in cords only in the latter two groups (the bilaterians).
Comparing the expression of genes in Drosophila and mouse for extracellular signals that provide positional information in embryonic development, Eric de Robertis and Yoshiki Sasai showed in 1996 that the ventral cord of the insect and the dorsal cord of the vertebrate express similar genes, and are thus putatively homologous on molecular bases. Dorsal‐ventral patterning in insects and vertebrates therefore appears to be controlled by homologous morphogens with mutually antagonistic actions. As a consequence, they argued that the position of the mouth (which is also homologous in protostomes and deuterostomes) changed between lineages, causing “dorsal” and “ventral” surfaces to become inverted (that is, “the animal lies on its back”). De Robertis and Sasai (1996) coined the term Urbilateria for the earliest bilaterally symmetrical animals. They proposed Urbilateria had a ventral nerve cord, but “turned over” by changing the location of the mouth in deuterostomes. This was based on the (incorrect) assumption that the protostome form would have been ancestral, in line with Geoffroy Saint‐Hilaire’s idea of an inversion of the dorsal‐ventral axis in deuterostomes.
The origin of the bilateral nervous system thus goes back at least to the Urbilateria. Based on the simple comparison between mammals and Drosophila, Hirth and Reichert proposed a single, monophyletic brain origin, with a tripartite brain (comprising the hindbrain, forebrain/midbrain, and an intervening boundary region) and extended central nervous system already evident in the last common bilaterian ancestor (Hirth et al., 2003; Hirth & Reichert, 2007). But how to reconcile this monophyletic origin of all bilaterally symmetric animals with the many different shapes and types of nervous systems represented by extant Bilateria, which include insects, cephalopods, tunicates, mammals, and even the radially organized adult nervous systems of echinoderms?
The tripartite brain of bilaterians is supposed to have arisen after the diversion of the cnidarian and protostome/deuterostome lineages (Hirth, 2010), circa 630 million years ago (Erwin, 2009; Peterson et al., 2004). Alternately, cladistic analysis suggests that neurons, centralized nervous systems, and brains arose independently as many as seven times amongst Bilateria (Moroz, 2009). This analysis indicates that Urbilateria did not possess a tripartite brain, and probably no brain at all, but rather an uncentralized nerve net, similar to that maintained by Cnidarians today. In this scenario, modular mechanisms of development are invoked to explain why homologous genes might organize brains that are not, themselves, homologous (Moroz, 2009). Importantly, positing that the ancestral Urbilaterian nervous system was a neural net turns the otherwise improbable dual independent origins of ventral (protostome) and dorsal (deuterostome) nervous systems into the most parsimonious scenario, in which homologous molecular modules acted independently to form the two nonhomologous nervous systems (Northcutt, 2010).
Along the same lines, the similar patterns of Hox gene expression in the vertebral spinal cord and ventral nerve cord of insects, and of Pax‐related genes in forebrain, eye, and hindbrain (Halder, Callaerts, & Gehring, 1995; Harris, 1997) in vertebrates and invertebrates do not necessarily make these structures homologous across the two clades. Rather, these are other instances in which the same genes may be used independently in the evolution of brain structures (Northcutt, 2010). In this case, the tripartite urbilaterian brain—considered by Heinrich Reichert, Frank Hirth, and Antonio Simeone to have had paired eyes, forebrain, a midbrain–hindbrain boundary (equivalent to the deuto–tritocerebrum boundary of invertebrates), and a hindbrain, with the telencephalon and most of the modern midbrain absent—would not have existed, and tripartite brains would have arisen independently, but from the same genetic modules, in vertebrates and invertebrates at the Ur/Bilateria branching (Northcutt, 2010).
A similar conceptual reorganization has taken place in our understanding of the evolution of the vertebrate brain. Amongst deuterostomes, the vertebrate brain was previously considered to be an invention exclusive of extant cephalochordates, given that urochordates (tunicates) lack a well‐organized brain. Recently, however, Pani et al. (2012) found that genes involved in patterning the neurectoderm in vertebrates are also expressed in a hemichordate. This finding suggests that the genetic programs that were eventually modified into patterning the vertebrate brain already existed in an ancestral creature that lived over 600 million years ago and survived into Cambrian times, but then degenerated in amphioxus and tunicates, remaining (but patterning divergent structures) in hemichordates and vertebrates.
Besides having a well‐organized brain, vertebrates differ from other chordates in that only the former are active, mobile predators, directed by an array of specialized sense organs, most of which are concentrated in the head. These sense organs are formed in development from neurogenic epidermal placodes, or thickenings of the ectoderm. The neural crest also contributes to the formation of vertebrate sense organs and other structures, and it was on this basis that Carl Gans and Glenn Northcutt proposed in 1983 that the elaboration of neural crest and neurogenic placodes was the seminal event in the origin of vertebrates, producing an enlarged brain and paired eyes and so leading to the formation of the new vertebrate head (Gans & Northcutt, 1983). Obviously, however, the head is not a vertebrate invention, as many protostome invertebrates also exhibit a well‐defined head. It will be interesting to watch as continued research unveils the similarities and differences that go into building protostome and deuterostome heads.
Finally, proteomic studies of the molecular components of mammalian synapses, and their homologous proteins in other species, point to ancestral molecular machinery in unicellular organisms that existed well before the evolution of metazoans and neurons (the protosynapse; Ryan & Grant, 2009). For example, cadherins and ephrin receptors are also found in choanoflagellates, GABA and metabotropic glutamate receptors are found in Poriferans, and several synaptic proteins are found in Fungi (Ryan and Grant, 2009). Thus, regardless of when tripartite brains, a CNS, or even a neuron first appeared in evolution, the ursynapse most likely predated it, appearing sometime after the branching of Poriferans but before the branching of Cnidarians. Tomás Ryan and Seth Grant (2009) propose that, in this “synapses first” scenario, it may have been the evolution of the synapse that led to the evolution of the neuron.
2.3 New Understandings of Brain Structure
The main divisions of the human central nervous system—spinal cord, medulla, pons, cerebellum, diencephalon, mesencephalon, and telencephalon—are recognizable in all vertebrates. Amongst these structures, however, the telencephalon differs the most across species. Ludwig Edinger proposed in 1908 that the preeminence of the telencephalon in mammals, and particularly in humans, was a sign of the human evolutionary status as “highest” amongst animals. At that time, evolutionary relationships among vertebrates placed mammals as the most recently evolved group. That, however, was to change later in the 20th century, as mammals (the only remaining Therapsids) came to be recognized as a sister group to reptiles/birds (Sauropsids), rather than as their descendants (see Figure 2.1; Carroll, 1988).
But at the beginning of the 20th century, and in line with the idea of progressive evolution through gradual increases in complexity and size from fish to amphibians, to reptiles, to birds, to mammals—culminating with humans, of course—Edinger suggested that each new vertebrate group in evolution acquired a more advanced cerebral subdivision, much as the earth’s geological strata formed over time. He thus proposed that an ancestral brain (the palaeoencephalon, or “striatum”) controlled instinctive behavior, and had been followed by the addition of a newer brain (the neoencephalon, or pallium, or “cortex”), which controlled learned and intelligent behavior (Edinger, 1908). The ensuing view was to become dominant in neuroscience, codified in an important comparative neuroanatomy text (Kappers, Huber, & Crosby, 1936): that the primordial telencephalon of fishes had a small pallium (a palaeocortex) and a larger subpallium (the palaeostriatum), to which an archistriatum and archicortex were added in reptiles. Birds would have evolved a hypertrophied striatum, but not any further pallial regions; in contrast, mammals were thought to have evolved the latest and greatest achievement, on top of the primitive palaeo‐ and archicortices: the “neocortex.” Thus, the striatal structures in fish, reptiles, and avians would correspond to the mammalian striatum, and their limited pallium would be a mostly olfactory version of what would become the mammalian neocortex (reviewed in Jarvis et al., 2005).
The (mistaken) idea that the neocortex was a recent mammalian invention gained popularity when neuroanatomist Paul MacLean evoked them in his view of a “triune brain” (MacLean, 1964, 1990), consisting of a reptilian complex (from the medulla to the basal ganglia) which evolved first, to which was added a “paleomammalian” complex (the limbic system), and finally a neomammalian complex (the neocortex). The intuitive (but incorrect) equation of evolution with progress, along with the alluring notion of a primitive reptilian brain, supposedly incapable of anything as complex as what a mammalian neocortex can achieve, attracted much attention from the popular media once it was used in Carl Sagan’s popular book, The Dragons of Eden (Sagan, 1977). Building on the “evolutionary” version of the scala naturae, Edinger thus established the basis of a nomenclature that was used for an entire century to define the cerebral subdivisions of all vertebrates—and one that, through the words of MacLean, to this day influences popular concepts of brain evolution.
In parallel to changing views on vertebrate evolution, neuroanatomy slowly accumulated evidence against Edinger’s progressive school of telencephalic advancement. Path‐tracing and behavioral studies in the mid‐1960s found that, like the mammalian neocortex, the neostriatum and hyperstriatum of the avian dorsal ventricular ridge (DVR) receive sensory input from the thalamus, and carry out the same type of information processing as is performed by cortical layers. The archistriatum and hyperstriatum give rise to descending projections to premotor and motor neurons, like the mammalian cortico‐bulbar and cortico‐spinal pathways. Moreover, also like the mammalian neocortex, the avian DVR is crucial in motor control and sensorimotor learning (reviewed in Jarvis et al., 2005).
These functional similarities were captured in the nuclear‐to‐layered hypothesis formulated by Harvey Karten in 1969 (Karten, 1969, 1991) that proposed that the striatum of birds and the neocortex of mammals are homologous. According to this hypothesis, the common ancestor of birds, reptiles, and mammals had cells organized into a globular structure, the DVR (the ensemble of hyperstriatum, neostriatum, and archistriatum) and this structure was reorganized into a laminar pallium early in the mammalian lineage, while maintaining the functional connectivity that determines distinct functional areas and relationships.
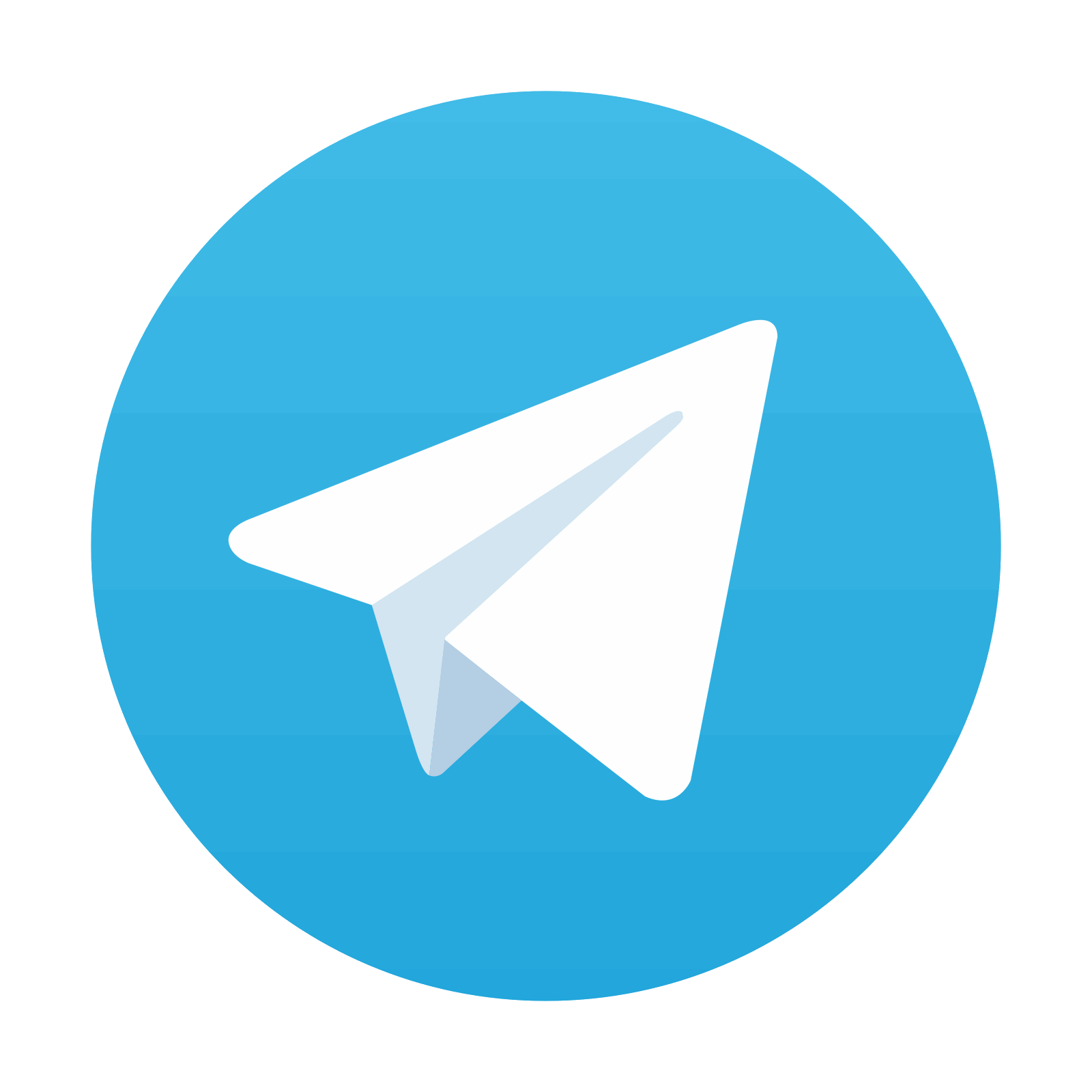
Stay updated, free articles. Join our Telegram channel

Full access? Get Clinical Tree
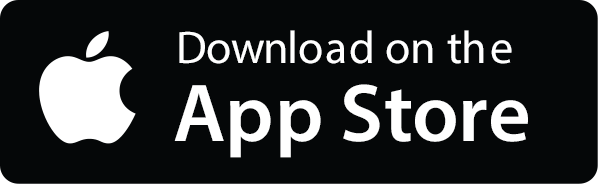
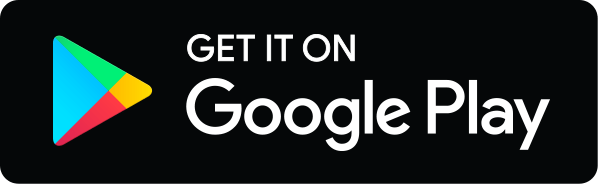