18 The Intensive Care Management of Spine-and Spinal Cord-Injured Patients Joshua E. Medow and Daniel K. Resnick In North America acute spinal cord injury affects between 12,000 and 14,000 people per year,1 and 200,000 people have suffered a significant spinal cord injury.1,2 The average age of injury is 34, and men are four times more often affected than women. Approximately 3% to 25% of spinal cord injuries occur after the initial traumatic insult.3–8 Many of these patients have other life-threatening injuries to the limbs, abdomen, thorax, and head and the vascular structures contained therein. Early management begins with immobilization in the field and rapid transport to a local facility or tertiary care center. Significant improvements have been made in the prevention of morbidity as a result of these injuries because of the institution of emergency medical services9–12 and early spine immobilization. Improved outcomes may also be achieved through the use of a multidisciplinary approach. Centers that specialize in the management of patients with spinal cord injuries have, in some cases, reported more favorable outcomes.13–21 This chapter describes the intensive care management of patients with acute spine and spinal column injuries. Ruling out a significant spinal injury is important to allow the discontinuation of unnecessary immobilization devices and to facilitate nursing care and patient mobilization. In sober, alert, and oriented patients without a distracting injury, who have not received pain/sedative medications and who do not have spine pain, there is no indication for spine imaging. The vast majority of patients that present to the intensive care unit (ICU), however, have multiple injuries and may have an altered level of consciousness. Consequently these patients should remain immobilized with spinal precautions in place until the spine can be cleared. Patients should be removed from the hard, immobilization backboard as soon as possible to prevent skin breakdown. Anteroposterior (AP) and lateral radiographs are required for the clearance of the thoracic and lumbar spine. These films must show views of all vertebrae so that deformity, malalignment, or fracture can be ruled out. In the thoracic and lumbar spines, entirely normal radiographs preclude the need for any further workup. Fractures of the spinous and transverse processes are often inconsequential and usually do not require further workup unless the patient is symptomatic. If there is any question as to the presence or absence of a fracture, computed tomography (CT) may be used as a definitive means to rule out bony injury. Isolated ligamentous injury without bony injury or malalignment of the spine is rare but can occur. In the presence of persistent pain, or if there is any relevant neurological deficit, magnetic resonance imaging (MRI) should be employed to rule out a ligamentous injury or epidural hematoma. In the cervical spine AP, lateral, and odontoid views are necessary and should be supplemented with CT for regions that are suspicious or not well visualized. At many institutions, the speed and availability of spiral CT make this modality useful, particularly given that the scan may be performed at the same time as a head CT in the obtunded patient. The craniocervical junction as well as the cervicothoracic junction must be clearly imaged to radiographically clear the cervical spine.22,23 Unlike thoracic and lumbar spines, ligamentous injury is not uncommon in the cervical spine. In patients with mechanisms of injury sufficient to cause ligamentous injury, fluoroscopic or MRI clearance in addition to standard x-ray or CT imaging is recommended prior to removal of immobilization devices.2 Because cervical collars can cause skin breakdown, cervical spine clearance should be obtained as soon as possible. In patients that are alert and oriented and not receiving pain medications clinical clearance can be obtained by having the patient move the neck in all orthogonal planes. If pain is present then this method of cervical spine clearance will be inadequate. Within 48 hours of the injury, an MRI of the cervical spine can be performed to assess for soft-tissue edema, which is a predictor of ligamentous injury.24 A negative MRI study is predictive of a lack of injury given the exquisite sensitivity of MRI. A positive finding is not necessarily indicative of injury, however, because the MRI is not particularly specific. In patients that at a later date have no pain and are alert and oriented but have remained in a cervical collar, flexion and extension x-rays with patients moving the neck under their own volition is indicated to assess for abnormalities in vertebral movement. In patients that do not maintain a level of consciousness adequate for standard flexion/extension x-rays, fluoroscopic flexion and extension views can be obtained to evaluate for abnormal vertebral movement in controlled, real-time fashion. If the neck muscles are not splinting during static or fluoroscopic flexion and extension views and there is no alignment abnormality, the cervical spine can be considered clinically cleared. Patients with evidence of a fracture dislocation of the spine should remain in an immobilizing device until reduction and stabilization can be safely performed. Depending on the level and the type of injury certain external orthotic devices may be indicated, whereas other injuries may require surgical intervention. A hard cervical collar provides some stability for many fractures or minor ligamentous injuries from the occiput to T1 but generally has its greatest effect from the occiput to C3.25 A cervicothoracic orthotic (CTO) provides additional stability from the occiput to about T3.25 A properly placed halo device will provide more stability than a CTO at the same levels (occiput to T3).25 The Lerman Minerva orthotic device (Trulife, Inc., Poulsbo, WA) provides stability at the cervicothoracic junction from about C2 to T3, but support above C2 is significantly less.26 The thoracolumbar spine orthotic (TLSO) provides support from T9 to S1 but poorly controls lower lumbar levels and the lumbosacral junction.26 Additional stability from T2 to T8 can be obtained if a chin extender is added to the TLSO construct. A Jewett brace (Florida Brace Corporation, Winter Park, FL) can be employed for injuries located at the thoracolumbar junction spanning from T8 to L2 and is generally ineffective for two- or three-column injuries.26 Thus there are several bracing options available to patients with damage to the structural components of the spine. The correct brace is determined by the level and biomechanical characteristics of the injury. Orthotic devices are not entirely benign and their use has been associated with a variety of complications. Some complications are more common in patients with spinal cord injuries and in patients who need to wear a brace for a relatively long period of time. Decubitus ulcers are found under cervical collars in 44% of patients within 6 days of placement of the orthotic device.27 The consequences of these ulcers can be significant and may involve osteomyelitis, significant scarring, nerve compression and dysfunction, local infection, and sepsis. Thus it is extremely important to ensure a properly fitted orthotic device, especially if the patient needs to wear the device longterm. It is imperative to check for decubitus ulcers regularly and to treat them early. It is also important to remove the orthotic device as soon as possible to help prevent decubitus ulcers from occurring without sacrificing the safety of needed immobilization. Spine immobilization also increases the risk of pressure ulcers in other parts of the body when the patient is not turned frequently enough, and can occur in as little as 2 hours.28 The length of time on a hard backboard has also been associated with the development of pressure sores. The best ways to prevent the development of decubitus ulcers include turning the patient frequently, applying a properly fitted orthotic device, and keeping the skin clean and dry. 29,30 Stiff cervical collars can result in marked elevation of intracranial pressure (ICP), with a mean increase of 4.5 mm Hg associated with cervical collar application.31 This is most likely due to venous congestion caused by compression of the jugular veins. Appropriately applied orthotic devices can significantly impact respiratory parameters in normal human subjects and may also increase the risk of aspiration.32 The effects are consistent with restrictive lung disease on pulmonary function tests. The implication is that orthotic devices can complicate potentially impaired respiratory function in patients with acute spinal cord injury. Closed reduction of cervical spine facet dislocation injuries is safe in the awake patient without rostral cervical spine injury.33 Because tongs are often used in the reduction, care must be taken to ensure that there is no fracture of the cranium that could result in an adverse outcome from pin placement. No MRI is needed to rule out an acute herniated disk prior to awake closed reduction but is frequently indicated in patients who are not completely awake, patients who fail attempted closed reduction, or patients who are treated with open reduction while anesthetized. Thirty to fifty percent of patients with a fracture subluxation will be found to have a traumatic herniated disk. The importance of this finding is unclear.33 During closed reduction, muscle relaxants may be used to prevent splinting of the neck muscles, and mild sedation is often indicated for relief of anxiety. Tongs are placed just above the pinna of the ear using local anesthetic. Slight variation in placement of the tongs may be used to promote upper cervical flexion orextension to some extent. As a rule of thumb, weight is added in 5- to 10-lb increments up to approximately 10 lb per level.34 Thus, if C6 is subluxed on C7, then 60 lb of traction may be safely applied. Some authors advocate the use of more weight, however, and there is some variance in technique from center to center. X-ray or fluoroscopic imaging is obtained after each change in weight. Once the deformity is reduced, the weight should be reduced to prevent overdistraction; however, the patient should be maintained in traction or in a brace until definitive stabilization can be achieved. The onset of neurological symptoms, the inability of the patient to tolerate the procedure, and the presence of overdistraction on imaging are all indications that the attempted reduction has failed. In these instances, the weight should be removed, the spine immobilized, and further study performed to determine the reason for failure. MRI is often indicated in patients that fail closed reduction because they will usually require open reduction. As with any trauma patient, assessment should begin with airway, breathing, and cardiovascular status and should include inline stabilization of the entire spine until it can be clinically and/or radiographically cleared. The assessment should continue with a scaled score of function that reflects the level of injury. The Guidelines for the Management of Acute Cervical Spine and Spinal Cord Injuries recommends the use of the American Spinal Injury Association (ASIA) score, which assesses motor and sensory function.35 It also incorporates the functional independence measure (FIM), which addresses activities of daily living. This measurement system gives the most accurate and reliable description of spinal cord injuries in the acute setting.35 Combined with a recommended outcome assessment such as the modified Barthel index, the ASIA scale can facilitate the communication of patient status and prognostic information between caregivers.35 It is recommended that acute spinal cord injuries be managed in the ICU setting, especially in high cervical lesions.36 Monitoring should include blood pressure and pulse, respiratory status, and neurological function. Additional management of patients with acute spinal cord injuries is often difficult and not necessarily backed by clear evidence as to method or duration of treatment. Consequently it is often difficult for caregivers to address the multiple medical issues that present in this patient population. The National Acute Spinal Cord Injury Study (NASCIS) reported evidence for the efficacy of steroid use in acute spinal cord injury. NASCIS I reported no significant change in motor or sensory function with steroid treatment.37 However, animal studies suggested that the doses of methylprednisolone used in NASCIS I were too low to demonstrate a significant improvement in outcome.38–42 This prompted NASCIS II.39 In this study methylprednisolone was bolused 30 mg/kg IV over 15 minutes followed by 5.4 mg/kg/h started 45 minutes later for the next 23 hours and then 4.0 mg/kg/h for the next 24 hours. Improved neurological outcome was noted with this protocol when it was started within 8 hours of the injury.43 The beneficial effects of steroid use are generally agreed to be small, however, and there is a definite increase in pulmonary and infectious complications associated with their use. For this reason, the use of the methylprednisolone protocol is recommended at an option level in the Guidelines for the Management of Acute Cervical Spine and Spinal Cord Injuries.44 At our institution, steroid use is limited to otherwise appropriate patients without diabetes; severe pulmonary problems, including pneumonia or contusions; or penetrating injuries. Spinal cord blood flow may be compromised following injury due to multiple factors. Often there is a combination of systemic hypotension and local vascular changes that include direct injury and focal vasospasm.38,45–51 Spinal cord injury itself may cause decreased blood pressure due to neurogenic shock resulting from the loss of normal sympathetic tone. Typical findings of neurogenic shock include bradycardia and rhythm disturbances, decreased systemic vascular resistance (SVR), which results in decreased mean arterial pressure (MAP), and consequently decreased cardiac output.16,38,45,47,49–53 It is the lack of sinoatrial and vasomotor innervation that results in decreased cardiac output. Once the ability to maintain perfusion is compromised due to an inability to autoregulate blood flow, spinal cord ischemia develops.38,50,51 The first week after surgery is when most patients have cardiovascular instability.16 Clinical outcomes may be improved by maintaining systolic blood pressure greater than 90 mm Hg and MAP greater than 85 mm Hg using a combination of pressors and fluid resuscitation.15,19,20,22,52,54,55 Typically pressors, such as dopamine, that have both α and β agonist properties are employed. Other agents that independently cause ionotropic/ chronotropic responses separate from vasoconstriction can be used to emphasize one response over another. For example, if the pulse is too fast a more potent vasoconstrictor can be used. Norepinephrine (Levophed [Sanofi-Aventis, Bridgewater, NJ]) has primarily α agonist functions but does have some β activity as well. It causes profound vasoconstriction. Phenylephrine has pure vasoconstrictor properties but is not quite as potent as norepinephrine. Depending on the circumstances these different medications can be used to maintain MAP. However, it is important to maintain an appropriate intravascular volume to perfuse the renal and splanchnic vascular beds because vasoconstriction occurs primarily here and in the limbs. If the vascular volume is low and the SVR is too high, these organ systems can become ischemic. Typically it is important to maintain pulmonary wedge pressures (PWPs) between 12 to 18 mm Hg to obtain the best cardiac performance and systemic perfusion.56 Autonomic dysreflexia occurs in 85% of patients with acute spinal cord injuries above T6.57–59 Symptoms include substantial elevations in blood pressure, tachycardia or bradycardia, headaches, flushing, diaphoresis above the level of injury, and pupillary changes.57–59 It is most common in the early period after spinal cord injury and is the consequence of significant reflex sympathetic discharge triggered by a noxious stimulus (i.e., bladder distension). Treatment can involve α and β blockade and perhaps other neuromodulating medications such as gabapentin. Several issues must be considered in the respiratory management of patients with acute spinal cord injuries. Many of these pertain to direct injury to the lungs sustained during the trauma, aspiration, pneumonias, pulmonary edema (often neurogenic), and adult respiratory distress syndrome (ARDS). Abnormal airway reactivity has been reported in patients with spinal cord injury as well.60 Bronchospasm that develops as a consequence of the injury typically responds to bronchodilators. Many spinal cord injury patients have bronchial mucus hypersecretion as well. Patients with spinal cord injuries are also at increased risk of obstructive or mixed sleep apnea.61,62 A significant concern in patients with spinal cord injuries is the denervation of the muscles of respiration because pulmonary dysfunction accounts for the largest cause of morbidity in spinal cord injured patients.63 In one study, only 25% of patients that required chronic positive pressure ventilation survived for 1 year and only 60% of them were alive at 14 years.64 The anatomical location of the injury influences the physiology of respiration. During inspiration, the rib cage expands as a result of the contraction of the external intercostal muscles and the diaphragm. When the external intercostal muscles are paralyzed the ribs may move inward during respiration rather than outward. This paradoxical motion decreases the development of negative inspiratory pressure and compromises ventilation.65,66 The tone of the abdominal muscles is also important in providing the appropriate amount of intra-abdominal pressure. Intra-abdominal pressure provides apposition and tension on the diaphragm allowing it to contract effectively. After a spinal cord injury, the abdominal muscle tone present in normal individuals is often lost.67–70 Consequently inhalation is often more difficult and less efficient. A change from the seated to the supine position generally results in a decrease in functional residual capacity (FRC) by 500 mL, whereas vital capacity (VC) increases. Over time, the neural input to the diaphragm may increase. This is known as operational length compensation (OLC).71,72 When normal subjects are upright, abdominal pressure decreases, and this may be exaggerated in patients with spinal cord injuries. In this situation, OLC may be inadequate resulting in the inability of the patient to ventilate adequately when upright.73 In patients with abdominal muscle paralysis, the use of an abdominal binder may augment ventilation, whereas a rocking bed may help patients with marked diaphragm weakness.69,74–76 Patients with high cervical injuries have the highest incidence of respiratory complications, including recurrent pneumonias, atelectasis, and respiratory failure. They also recover more slowly and have the highest mortality rate compared with other spinal cord injured patients.63,77,78 Quadriplegic patients have blunted responses to hypercapnia and small increases in respiratory drive.79 Patients with injuries above C3 require diaphragmatic pacing or chronic ventilatory support. Patients with injuries between C3 and C5 have variable impairment of diaphragmatic strength. Chronic ventilator dependence is most common among patients older than 50 with underlying lung disease. For many patients, however, mechanical ventilation is often necessary only in the acute phase and is usually not necessary longterm.80–82 Improvements are noted due to functional descent of the neurologically injured level over time as spinal cord edema resolves and there is recruitment of accessory ventilatory muscles, strengthening of deconditioned muscles, and the evolution of spasticity from flaccidity.80–84 These patients may utilize intermittent glossopharyngeal breathing (which is a combination of oral, pharyngeal, and laryngeal muscle movements to project a bolus of air past the glottis) to help with coughing, increasing VC for deeper breathing, and for raising the vocal volume.85–87 Patients with diaphragmatic pacers can also demonstrate improvements in the fluidity of speech. Patients with injuries between C5 and C8 have intact diaphragm innervation and can use accessory muscles in the neck and the clavicular portion of the pectoralis major muscles to inhale adequately. Exhalation is by passive recoil.88,89 Thus spinal cord injured patients may have increased residual volumes (RVs) due to an inability to exhale and may appear to have restrictive ventilatory defects during pulmonary function testing.81,84,89–92 Patients with thoracic spinal cord injuries may still have ventilatory complications but not necessarily as a consequence of neurological compromise. Many of these patients have other direct chest trauma, including pulmonary contusions, hemo-/pneumothorax, and so forth.62,63 Furthermore, these patients may develop ARDS either from direct injury, chemical pneumonitis from aspiration, and aspiration pneumonia. All patients with central nervous system injuries are at some risk for the development of neurogenic pulmonary edema; however, it rarely occurs in complete spinal cord injuries above C7.93–95 It is thought to be related to secretion of protein-rich fluid in the presence of vasogenic instability from aberrant sympathetic discharge. Primary management is supportive therapy until the problem clears. Cardiogenic pulmonary edema can also occur as a consequence of spinal cord-induced bradycardia. Chest physiotherapy appears to decrease the risk of mucus retention, atelectasis, and pneumonia in patients with spinal cord injuries.78,96 This strategy includes incentive spirometry, frequent changes of position/postural drainage of secretions, nasotracheal suctioning, and, in patients with a weak cough, manually assisted coughing using forceful abdominal thrusts if no precluding abdominal or spinal injury exists. In quadriplegics, surface stimulation of abdominal muscles with an electrical charge was shown to be as effective as abdominal cough assistance.97 There are no data to support prophylactic use of bronchodilators or intermittent positive pressure ventilation.98,99 Patients with severe high cervical cord injuries, or those with concomitant head or pulmonary injuries, may require prolonged mechanical ventilation. In these patients, tracheostomy should be considered early because of the risk of laryngeal damage resulting from chronic endotracheal intubation. The incidence of deep venous thrombosis (DVT) in chronic spine injured patients within 1 year has been reported at 2.1% and 0.5% to 1.0% per year thereafter.100 The concern of DVT is progressive loss of circulation in the limb with concomitant pain and ischemia, chronic limb swelling, and pulmonary embolism. The diagnosis of DVT may be made with a variety of tests, including Doppler ultrasound, impedance plethysmography, venography, and fibrinogen and D-dimer levels.101–125 The gold standard is venography, but because of its expense and the invasive nature of the test, it is often impractical to use.67 Venography also carries a 10% risk of phlebitis and allergic reaction to the contrast.110 Pulmonary embolism has been known to occur in patients with negative venograms.104,107 Doppler ultrasound and impedance plethysmography are 80% to 100% accurate for the diagnosis of DVT compared with venography.126 Doppler ultrasound is less sensitive for DVTs below the knee than it is above the knee due to the smaller size of distal veins. D-dimer and fibrinogen tests are very sensitive but are often unspecific, meaning that they will not miss a DVT but when positive the chance that a DVT is present might not be very high.119,120 After careful consideration of these different modalities, the Consortium of Spinal Cord Medicine has recommended the use of Doppler ultrasound to diagnose DVT.127 Subcutaneous (SQ) unfractionated heparin, 5000 units b.i.d. or t.i.d., has been shown to substantially reduce DVT formation.103,105,106,110–112,118,123,124,128 However, in patients with acute spinal cord injury, low-dose unfractionated heparin has been considered inadequate by some authors.108,113 Attaining an activated partial thromboplastin time (aPTT) of 1.5x normal has been suggested but does result in a higher bleeding complication rate compared with fixed dose SQ heparin. As compared with oral anticoagulation, low-dose heparin was shown to have better efficacy in preventing DVT.111 The use of low molecular weight heparin (LMWH), otherwise known as fractionated heparin, has been studied as well and has had favorable results as compared with unfractionated heparin in both prevention of DVT and decreased bleeding complications.109 Other reports have shown significant efficacy of LMWH against DVT and pulmonary embolism (PE) in patients with spinal cord injuries.119,129 Because most pulmonary emboli occur within 2 to 3 months, prophylaxis with anticoagulation usually spans an 8- to 12-week period. Patients with other risk factors such as obesity, previous DVT or PE, or malignancy may stay on prophylactic anticoagulation therapy longer.104 Patients who have useful motor function in the lower extremities may run shorter courses of anticoagulation because they are at less risk for developing a DVT.114,127 Inferior vena cava (IVC) filters have been used for patients who do not tolerate anticoagulation, as well for the prevention of massive PE. The filter device can prevent large thromboembolic events from occurring but does not necessarily prevent smaller thromboemboli from resulting in PE. They do not prevent upper extremity pulmonary emboli. IVC filters can also contribute to the formation or enlargement of DVTs because they cause resistance to flow. Complications of filter placement include distal migration, intraperitoneal erosion, and symptomatic IVC occlusion.130–132 In a randomized trial that evaluated routine placement of vena caval filters as an adjunct to anticoagulant therapy in patients with proximal DVT, filters were shown to reduce the frequency of PE during the first 12 days but to almost double the long-term risk of recurrent DVT.133 Hence a newer generation of filter has been designed that can be removed within 2 weeks of implantation for patients in whom the risk for PE is high but anticoagulation cannot be used. Other preventive measures include compression stockings and sequential compression devices, which have been shown to lower the risk of PE.134 The use of rotating beds for 10 days in patients with acute spinal cord injuries has also demonstrated a decreased incidence of DVT by 80%.101 We generally apply pneumatic compression devices immediately and start LMWH within 24 to 72 hours, depending upon other injuries and contraindications to anticoagulation. Treatment of a known DVT should include full anticoagulation with unfractionated or fractionated heparin followed by warfarin therapy, usually for >3 months and with a goal international normalized ratio (INR) of 2.5.135 In patients that cannot undergo anticoagulation, an IVC filter should be considered.135 The incidence of thromboembolic events in patients with spinal cord injuries ranges from 7% to 100%,102,105,107–109,112,114,117,118,123,136,137 and morbidity and mortality are quite high in the acute injury setting.127,138 Most PEs occur in the first 2 to 3 months after injury,104,115–117 and patients with acute spinal cord injuries have a 500-fold increase in mortality from a PE than age- and sex-matched, noninjured patients.138 This risk decreases to a 20-fold mortality rate between spinal cord injury patients who are 6 months out from their injury and their noninjured counterparts.138 Signs and symptoms of pulmonary embolism include tachycardia, hypotension/shock, myocardial infarct, tachypnea /dyspnea, apprehension, diaphoresis, fever, chest pain, cyanosis, cough/hemoptysis, and complete cardiovascular collapse with sudden death.139,140 Diagnostic findings can include platelike atelectasis on chest x-ray, right ventricular axis shift on electrocardiogram (ECG), and supraventricular tachycardia.141 With severe pulmonary embolism, ST segment changes and T-wave inversion may be present as well.141 Although hypoxemia and a large A-a gradient on blood gas analysis is classically reported with PE, PaO2 and O2 saturation values are very inconsistent and should not be used to rule out PE.142–145 Death from PE is unlikely in patients without evidence of shock.141 These patients should begin full-dose anticoagulation with heparin, if possible, and a confirmatory diagnostic study should be obtained.141 In this patient population ventilation-perfusion (V/Q) scans, MRI, helical CT angiography, and the gold standard percutaneous venous angiogram are all viable options.146–148 Patients with shock due to PE are far more likely to die within the first hour, making rapid diagnosis extremely important.141 CT and V/Q scans may not be plausible because of the time delay incurred in obtaining a confirmatory study. Percutaneous angiography may be reasonable if the intention is to treat the PE with catheter embolectomy, mechanical clot disruption, or selective tissue plasminogen activator (tPa) injection.141 Oftentimes it is beneficial to obtain a bedside echocardiogram because it can be mobilized to the patient in the ICU rather than moving an unstable patient to an imaging department where ICU staff, equipment, and medical therapies are in short supply. Echocardiography (transthoracic or transesophageal) is useful in the recognition and differentiation of PE and the patient’s response to therapy.149,150 Echocardiography can detect emboli in transit and may provide alternative diagnoses for the cause of shock, including aortic dissection, myocardial dysfunction/infraction, pericardial disease, hypovolemia, and valvular insufficiency.151,152 Echocardiographic findings of PE include right ventricle pressure overload, enlarged right to left ventricle ratio, paradoxical septal motion, pulmonary artery dilatation, and tricuspid regurgitation.149,150,152,153 It appears that an embolism that causes ≥30% pulmonary artery (PA) occlusion is required to produce right ventricle dilatation and hemodynamic instability.154–156 Smaller, hemodynamically insignificant emboli (those that cause ≤20% PA occlusion) may not be detected with echocardiography.157,158 Echocardiography is also not able to establish the severity of a superimposed event in patients with preexisting left ventricle dysfunction.158 V/Q scans have been used for the diagnosis of PE. The majority of patients with angiographically documented PE (59%) do not have a high probability V/Q scan.159 Scan interpretations that are conclusively normal or read as high probability are rare, only 15% and 13%, respectively.159 The remainder of scans are interpreted as intermediate (38%) or low (34%) probability.8 In patients with chronic obstructive pulmonary disease (COPD) V/Q scans are even less diagnostic.160 Part of this may be due to the difficulty in performing ventilation scans in critically ill patients.161 Consequently V/Q scanning will often require an angiographic study to definitively confirm the diagnosis of PE.162 Angiography is recognized as the gold standard test to confirm PE but is invasive, expensive, and requires skilled staff to perform it.141 It is not uniformly available102 and is associated with multiple complications,162–164 particularly in critically ill patients160 and in patients with pulmonary hypertension.165 Although selective tPa delivery with a catheter is an accepted treatment modality, nonselective IV tPa with angiography has been shown to have an increased bleeding complication rate.166 Helical CT angiography is appealing as a diagnostic tool because it is readily available, noninvasive, can define alternative diagnoses,167,168 and can detect right ventricle dilatation similarly to echocardiography.169 When the findings of a helical CT are compared with those of a percutaneous angiogram for PE in the central arteries, helical CT was 94% sensitive, 94% specific, and had a 93% positive predictive value.170–175 Specificities nearing 100% have been reported in cases of PE that were clinically important with right ventricle pressure overload.173,175,176 Optimal scanning involves breath holding,177 but recent technology allows for faster slice acquisition, making motion less of a problem. MRI has also been employed for the diagnosis of PE. MRI accurately visualizes the central vessels, can be used to interpret heart function, and can provide alternative diagnoses with comparable sensitivity and specificity to helical CT angiography.178–180 It does not require iodine contrast, which can be nephrotoxic, and unlike CT can allow for MR venography during the same session.178,181 However, setup time, the duration of the scan, isolation of the patient in the bore of the magnet away from health care workers, and implantable devices that may not be MRI compatible often preclude the use of MRI in patients with hemodynamically significant PE. Patients with PE may maintain hemodynamic stability with an intense catecholamine surge.141 This is necessary to maintain blood pressure to the heart and central nervous system. Mechanical ventilation during a hemodynamically significant PE may be necessary if refractory hypoxia and shock ensue. However, starting mechanical ventilation can often blunt the catecholamine surge and precipitate cardiovascular collapse.141 This can be due in part to sedative/ hypnotic medications that decrease consciousness and catecholamine release and can also be due to direct vasodilatation.141 Furthermore, positive pressure ventilation can cause a decrease in venous return to the right ventricle and can also increase pulmonary vascular resistance resulting in further compromise of right ventricular function with a concomitant decrease in cardiac output and a drop in systemic blood pressure.141 Accordingly, intubation should be undertaken judiciously while weighing its risks and benefits.141 An awake fiberoptic intubation provides no loss of consciousness and permits direct visualization of the vocal cords, often with less stimulation than direct laryngoscopy. Etomidate should be used if sedation is necessary because it does not cause hypotension.141 Volume expansion with 1 to 2 L of crystalloid solution is the traditional treatment for hypotension in undifferentiated shock and is frequently helpful in driving cardiac output in patients with massive PE unless right ventricular failure is severe.141 Pressors such as norepinephrine (NE) are instrumental in improving systemic blood pressure and blood flow to the heart, resulting in less cardiac ischemia.182 Furthermore, NE has β-1 effects resulting in improved cardiac contractility and thus right ventricular function,183,184 which is why it is indicated in patients suffering from severe shock.185,186 Dobutamine and other pressors with strong β activity may cause hypotension from vasodilatation, and their use should be limited in PE.141 Inhaled prostacyclin and nitric oxide have been reported to increase cardiac output, decrease pulmonary pressures, and improve gas exchange in cases of severe PE.187,188 Placing the embolized lung in the dependent position can also improve oxygenation.189 Heparin should be started at full therapeutic doses until PE is excluded provided that no contraindications to heparin therapy exist.190 The efficacy of heparin is attributed to the impairment of clot propagation and the prevention of recurrent PE.191 Heparin therapy should be aggressively pursued in patients with suspected PE because recurrent PE is reported to be the most common cause of death in hemodynamically stable patients.192,193 Heparin boluses can be associated with hypotension as a consequence of histamine release and can be treated with histamine 1 and 2 receptor blockers to help prevent/treat hypotension.194,195 Long-term treatment often requires warfarin therapy for >3 months and with a goal INR of 2.5. Cardiac arrest will occur within 1 to 2 hours after the onset of clinical presentation in two thirds of fatal PE cases192,196 and is almost uniformly due to pulseless electrical activity (PEA).141 PEA is at least momentarily reversible in one third of cases.141 The survival rate for patients presenting with cardiac arrest is reported at 35%.197 Those patients who experience intermittent cardiac arrest have a lower mortality rate than those requiring continuous resuscitation.198,199 Cardiopulmonary resuscitation (CPR) not only promotes circulation by pumping the heart but can also mechanically disrupt the embolus, permitting improved flow through the pulmonary artery.141 Thrombolysis is uniformly accepted as the treatment of choice in hemodynamically unstable PE200–202 but is not without bleeding complications. Relative and absolute contraindications may prevent the use of this medication. Selective tPa delivery during angiography may help reduce the dose necessary to be effective and consequently may decrease risks of bleeding. Catheter embolectomy or fragmentation is an option for patients not in cardiac arrest203 where tPa is contraindicated. Open embolectomy is another treatment possibility but it requires cardiopulmonary bypass and full anticoagulation with heparin. Cardiac arrest does not preclude open embolectomy; however, it requires general anesthesia, which can decrease cardiac output and precipitate cardiac arrest, complicating matters further for patients with hemodynamically significant PE.204 Vertebral artery injuries occur in up to 11% of nonpenetrating cervical spine injuries.6 They are most often due to fractures through the foramen transversarium, facet fracture-dislocation, or vertebral subluxation,205–212 all of which are readily seen with CT scans of the cervical spine. The diagnosis of a vertebral artery injury can be made by angiography, CT angiography, or MR angiography. Ultrasonography has been used as well. Dissection of the vertebral artery can result in posterior circulation vascular insufficiency or ischemia and stroke. Most patients are, however, asymptomatic. The literature supports the treatment of patients with vertebral artery injury and concomitant posterior fossa stroke with intravenous heparin.205 Patients that have evidence of impaired posterior circulation but no stroke may be treated with observation or heparin.205 Asymptomatic patients need not receive heparin based on the review of literature noted in the Guidelines for Management of Acute Cervical Spine Injuries.205 The role of angioplasty and stenting is unknown at this time. The risk of significant hemorrhage related to anticoagulation is ~14%205 and thus the decision to anticoagulate is not without consequences. Careful attention to all injuries sustained in a trauma and monitoring of neurological status in patients with vertebral artery injuries can lead to favorable outcomes. Patients with spinal cord injuries require nutritional support. Enteral feedings (EFs) should be started as soon as possible and it is recommended that they begin within 72 hours postinjury so that adequate supplementation can be titrated to goal rates by post-trauma day 7. Total parenteral nutrition (TPN), if necessary, is generally not started before day 5 because of higher morbidity concerns related to electrolyte and fluid shifts. Patients capable of safely taking nutrition orally should do so. If patients cannot tolerate oral intake, a nasogastric (NG) or orogastric (OG) tube should be placed to start EF early. NG or OG tubes provide the ability to evacuate the stomach to help reduce enteric distension and to measure residual secretions and feeds. Once the gastrointestinal (GI) tract is functioning satisfactorily a Dobhoff tube can replace the NG tube. Because it is narrower, the Dobhoff tube is thought to induce less nasopharyngeal swelling and may perhaps decrease the incidence of sinusitis compared with the larger NG tubes. Gastrostomy tubes should be employed early for those patients that will definitely need long-term nutritional support. Caloric intake should be 140% of the predicted basal energy expenditure (BEE) and 100% of the BEE in paralyzed individuals because of the elevated energy requirements within the first 2 weeks after injury. BEE can be calculated based on the Harris-Benedict equation. Indirect calorimetry is probably the best way to assess nutritional requirements.213 Fifteen percent of the total calories should come from protein. Overfeeding can result in cholestasis and significantly elevated liver function tests (LFTs). Patients receiving nutritional support will often demonstrate mild elevations in LFTs. This should not prompt halting of nutritional supplementation, but it should be monitored closely. Patients with acute spinal injuries encounter considerable obstacles during recovery. Recognizing the limitations of current therapies and the risks involved with their use is of the utmost importance. Careful attention to detail during the early phases of treatment can lead to lower morbidity and mortality rates. Some of the longer-term problems can potentially be avoided with good skin and wound care, appropriate use of orthotic devices, and careful anticoagulation. A multidisciplinary approach that treats the whole patient is necessary to improve quality of life and to facilitate functional recovery in this patient population. 11. Gunby I. New focus on spinal cord injury. JAMA 1981 ;245:1201–1206 16. Piepmeier JM, Lehmann KB, Lane JG. Cardiovascular instability following acute cervical spinal cord trauma. Cent Nerv Syst Trauma 1985;2:153–160 40. Ducker TB, Zeidman SM. Spinal cord injury: role of steroid therapy. Spine 1994;19:2281–2287 55. Wolf A, Levi L, Mirvis S, et al. Operative management of bilateral facet dislocation. J Neurosurg 1991 ;75:883–890 59. Mathias CJ, Frankel HL. Cardiovascular control in spinal man. Annu Rev Physiol 1988;50:577–592 101. Becker DM, Gonzalez M, Gentili A, et al. Prevention of deep venous thrombosis in patients with acute spinal cord injuries: use of rotating treatment tables. Neurosurgery 1987;20:675–677 115. Naso F. Pulmonary embolism in acute spinal cord injury. Arch Phys Med Rehabil 1974;55:275–278 141. Wood KE. Major pulmonary embolism: review of a pathophysiologic approach to the golden hour of hemodynamically significant pulmonary embolism. Chest 2002;121:877–905 181. Gefter WB, Hatabu H, Holland GA, et al. Pulmonary thromboembolism: recent developments in diagnosis with CT and MR imaging. Radiology 1995;197:561–574 190. Goldhaber SZ. Pulmonary embolism. N Engl J Med 1998;339:93–104 191. Kearon C. Initial treatment of venous thromboembolism. Thromb Haemost 1999;82:887–891 192. Dalen JE, Alpert JS. Natural history of pulmonary embolism. Prog Cardiovasc Dis 1975;17:259–270 196. Soloff LA, Rodman T. Acute pulmonary embolism, II: Clinical. Am Heart J 1967;74:829–847
Clearance of the Spine
Immobilization and Reduction
Choice of Immobilization Device
Complications of Immobilization Devices
Pressure Ulcers
Intracranial Hypertension and Cervical Collars
Pulmonary Issues
Closed Reduction of Cervical Dislocation Injuries
Acute Medical Management
Steroids
Blood Pressure Management
Autonomic Dysreflexia
Pulmonary Care
Deep Venous Thrombosis
Pulmonary Embolism
Treatment of Pulmonary Embolism
Vertebral Artery Injury
Nutrition
Conclusion
References
< div class='tao-gold-member'>
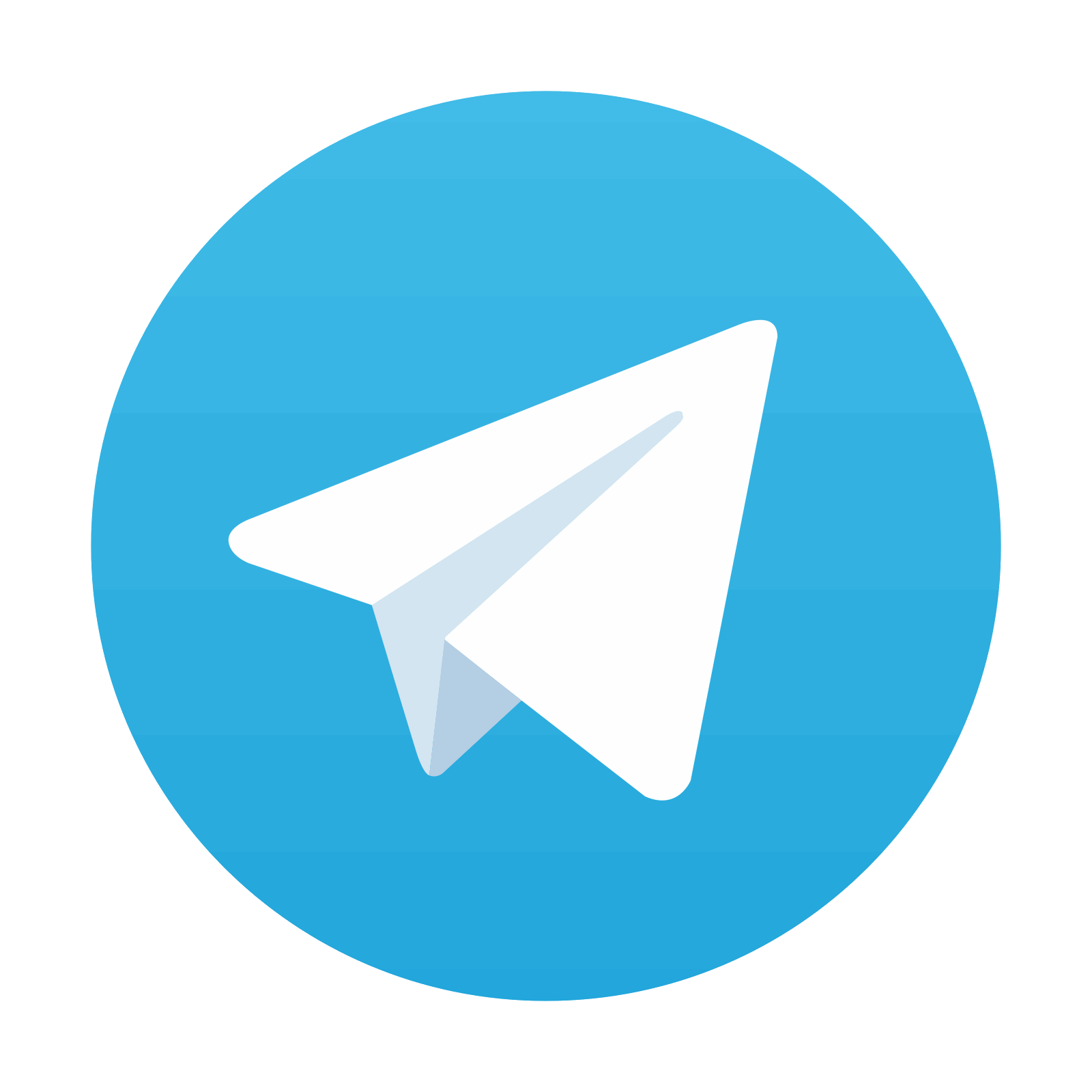