The Localization of Lesions Affecting the Cerebral Hemispheres
Lesion localization in the cerebral hemispheres relies on the understanding of the function of different portions of the cerebral cortex. Initially, hemispheric localization was learned from the clinical effects of vascular or other lesions, true “experiments of Nature.” Limited cortical activation procedures were performed intraoperatively since the 1920s, but in the last few years, cortical mapping has expanded prodigiously with the advent of functional neuroimaging. The two techniques most extensively used are positron emission tomography (PET), beginning in the mid 80s [324], and functional magnetic resonance imaging, beginning in the early 90’s [364,421]. Another technique, focused magnetic stimulation, by temporarily modifying the function of a restricted area of the brain, became another powerful instrument to clarify regional brain function [368,408]. More recently, white matter fiber tracking with diffusion tensor imaging—magnetic resonance imaging (MRI) tractography—was added to our localization toolbox [75]. Still in its infancy, but already promising great advances in our understanding of localization in individuals, the field of imaging genetics studies the effect of genetic variants on brain structure and function [56]. Whereas, anatomical measurements used to be made with rather simple techniques in relatively few individuals [175], much more sophisticated measurements can now be performed in large populations using voxel-based morphometry and other computer-aided techniques [513]. This edition of Localization in Clinical Neurology reflects the new insights into cortical localization gained from the use of these techniques.
Anatomy of the Cerebral Hemispheres
The paired cerebral hemispheres derive from the telencephalon [61]. They are in continuity with the diencephalon and are interconnected by white matter commissures, including the corpus callosum, the anterior and posterior commissures, and the commissure of the fornix. In the adult, the cerebral hemispheres, shaped like a cap, cover the midbrain-diencephalic structures. A midline sagittal slit, the longitudinal fissure, separates the two hemispheres. Thus, each hemisphere has a larger lateral aspect and smaller medial and inferior aspects. Folds (gyri) and furrows (sulci) pattern the surface of the cerebral hemispheres. The larger sulci (fissures) serve as anatomic landmarks separating the main regions of the cerebral hemispheres.
On the lateral aspect of each hemisphere, two large sulci separate three regions: the temporal lobe, inferior to the Sylvian fissure; the frontal lobe, anterior to the Rolandic or central sulcus; and the parietal lobe, posterior to the Rolandic sulcus (Fig. 20.1A). The insula lies buried in the depth of the Sylvian fissure. The most posterior portion of the lateral aspect corresponds to the occipital lobe. An imaginary line, drawn from the superior extent of the parietooccipital sulcus in the medial aspect of the hemisphere to a notch in the inferior aspect (preoccipital notch), constitutes the lateral boundary between the occipital lobe and the parietal and temporal lobes.
Two sulci running anteroposteriorly divide the frontal lobe into superior, middle, and inferior frontal gyri. Perpendicular to these, and separated from them by the precentral sulcus, lies the precentral gyrus, which is just anterior to the central sulcus. In the average normal person, but not in those with autism or other developmental language disorder, the posterior part of the third frontal gyrus (triangular and opercular portions) is larger in the left hemisphere [109,133]. This difference is more pronounced in men [47]. Also, the left precentral gyrus is thicker than the right in right-handed men [10]. The precentral gyrus contains the primary motor area. Primary cortical areas constitute the first areas of cortex to receive information from the sense organs, in the case of the primary sensory areas, or, in the case of the primary motor area, projects to the motor nuclei of the brain stem and spinal cord lodging the lower motor neurons. The inward or outward projections of the primary cortical areas contrast with the rest of the cortex, named association cortex, giving rise preferentially to cortico-cortical connections.
Two transverse sulci divide the temporal lobe into superior, middle, and inferior temporal gyri. On the inferior bank of the Sylvian sulcus, the transverse gyrus (Heschl) runs anterolaterally over the superior aspect of the first temporal gyrus. It constitutes the primary auditory area and the anterior limit of the planum temporale or supratemporal plane, which, in the average right-handed person, is one-third larger in the left hemisphere [175] (Fig. 20.2). Heschl gyrus is also larger on the left, particularly in men [187,271]. Functional specialization may explain this finding because in the average person there is an auditory bias toward the left hemisphere, such that, not only speech but even pure tones activate more Heschl gyrus on the left than on the right hemisphere [125]. Lateralization could begin in the cochlea [445]. Additionally, individual anatomy may determine or be determined by function. Normal individuals who analyze musical sounds relying on spectral pitch rather than on fundamental pitch have relatively larger right-sided Heschl gyri [420]. Not unexpected, considering that the left auditory cortex is relatively specialized in rapid temporal processing, whereas right auditory cortex shows a stronger sensitivity for spectral processing and a slower temporal processing mode [511].
FIG. 20.1. Lateral (A) and inferomedial (B) views of the cerebral hemispheres. The orbital aspects of the frontal lobes can be seen only in a direct inferior view, not shown in this figure. Depicted, however, is the inferior aspect of the temporal and occipital lobes.
FIG. 20.1. Lateral (A) and inferomedial (B) views of the cerebral hemispheres. The orbital aspects of the frontal lobes can be seen only in a direct inferior view, not shown in this figure. Depicted, however, is the inferior aspect of the temporal and occipital lobes.
Posterior to the Rolandic sulcus lies the postcentral gyrus, separated from the rest of the parietal convexity by the postcentral sulcus. The postcentral gyrus houses the primary somatosensory cortex. The most inferior extent of this gyrus, abutting the Sylvian fissure, contains the secondary somatosensory cortex. A transverse sulcus divides the rest of the parietal lobe into superior and inferior parietal lobules. Anteriorly, the inferior parietal lobule curves around the posterior extent of the Sylvian sulcus (supramarginal gyrus); posteriorly, around the posterior extent of the superior temporal sulcus (angular gyrus).
The medial or mesial aspect of the cerebral hemisphere sweeps around the corpus callosum and, posteroinferiorly, blends rather smoothly with the inferior aspect of the hemisphere (see Fig. 20.1B). Among the major sulci in the medial aspect, three run radially and one runs parallel to the corpus callosum. The latter, called the cingulate sulcus, separates the cingulate gyrus, centripetal to it, from the mesial aspect of the first frontal and paracentral gyri. The mesial aspect of the frontal and parietal paracentral gyri (paracentral lobule) is well demarcated from the rest of the mesial parietal lobe (precuneus) by one of the three radial sulci, namely, the marginal sulcus, which arises in the cingulate sulcus. The other two radial sulci are more posterior. The large parietooccipital sulcus separates the parietal precuneus from a mesial wedge of occipital lobe (cuneus), limited inferiorly by the calcarine sulcus. These two sulci meet anteriorly to join the posterior extent of the cingulate sulcus, which limits dorsally the isthmus of the cingulate gyrus as it sweeps around the posterior end (splenium) of the corpus callosum. As the cingulate gyrus courses infero-anteriorly around the splenium, it blends with the parahippocampal gyrus, in the most medial aspect of the temporal lobe. Hidden in the recess between the temporal horn of the lateral ventricle and the lateral aspect of the midbrain, the hippocampal gyrus courses anteriorly latero-superior to the parahippocampal gyrus, separated from it by the hippocampal sulcus. Anteriorly, they converge into a small nub (the uncus) that contains the amygdalar nuclear complex.
The inferior aspect of the hemisphere comprises the orbital surface of the frontal lobe and the inferomedial aspects of the occipital and temporal lobes (see Fig. 20.1B). A few irregular orbital gyri and a medially located straight gyrus (gyrus rectus), which lies medial to the olfactory bulb and tract, make up the orbitofrontal surface. The demarcation between the temporal and the occipital lobes is indistinct on their inferior aspect. A fusiform or occipitotemporal gyrus, anterolaterally, and a lingual gyrus, posteromedially, can be distinguished on the swath that lies between the collateral sulcus (lateral to the parahippocampal gyrus) and the inferior temporal gyrus.
In addition to the frontal and temporal lobe asymmetries mentioned above, the right frontal lobe is often larger than the left, and the left occipital lobe is larger than the right [187,271]. Such anatomic asymmetries may reflect the localization of language and other functional specialization of each cerebral hemisphere. In general, the right hemisphere is dominant for tasks requiring spatial and constructional skills, as well as for directed attention and body image, while the left hemisphere is dominant for language and motor functions, as well as linguistic thought and reasoning, analytic and mathematical skills, and the temporal sequencing of stimuli. The right hemisphere is also dominant for non-visuospatial perception, including somesthetic, auditory (melody and tone discrimination), and emotional functions (e.g., the comprehension of emotional tone in voice and body gestures).
The convoluted pattern on the surface of the cerebral hemispheres emerges during ontogenesis to accommodate into the smallest volume the large expansion of cortical gray matter (cortex) that characterizes the human brain. Six layers of cells (neurons) can be distinguished in most of the cortex (neocortex) (Fig. 20.3). From surface to depth, they have been termed (a) the molecular layer, rich in fibers; (b) the external granular layer, composed of small round or star-shaped neurons; (c) the external pyramidal layer, containing medium-sized pyramidal neurons, their larger apical dendrites oriented toward the surface; (d) the internal granular layer, which, in addition to small, round neurons contains a thick plexus of horizontally directed fibers; (e) the internal pyramidal or ganglionic layer, constituted by the larger pyramidal neurons; and (f) the multiform layer, made up of spindle-shaped neurons. Two small areas in the inferomedial aspect of the hemispheres have a simpler cortex: the olfactory area (paleocortex) and the hippocampal formation (archicortex). Except for the primary visual cortex in binocular primates, in which this number is doubled, the number of neurons in a column (see Fig. 20.3) through the depth of the neocortex is the same in different cortical areas and mammalian species [401]. In humans, the cortex is thicker to accommodate the same number of neurons that are further spread apart by the richer network of connections.
FIG. 20.3. The histologic appearance of the five fundamental types of neocortex, according to von Economo [488]: 1 = agranular (pyramidal); 2 = frontal; 3 = parietal; 4 = polar; 5 = granular (koniocortex).
Although it is six-layered throughout, the neocortex is not homogeneous. In areas that receive a heavy sensory projection, the granular layers are more bulky than the pyramidal layers (granular cortex or koniocortex, see Fig. 20.3). The opposite holds true for the areas in which the larger motor projections to the brainstem and spinal cord originate (agranular or pyramidal cortex). Actually, the cortex may be parceled according to the cellular composition (cytoarchitecture) of the various cortical areas. Brodmann cytoarchitectural map (Fig. 20.4) depicts 50 areas [62]. Better image processing techniques combining histological and “in vivo” imaging data and the use of other cortical markers, such as neurotransmitter receptor density, herald the arrival of more accurate, computer-guided, probabilistic maps of the human cortex [513].
The cerebral hemispheres process intraindividual and extraindividual information. Most of the latter input reaches the primary cortical areas through the thalamus. Information concerning the interior homeostasis travels from the brainstem and hypothalamus through the medial thalamus, reaching mainly the pericallosal, mesial temporal, insular, and orbital cortex (limbic lobe). In order to act, both of these systems need to be “activated” by the brainstem reticular formation.
The main anatomic connections of the cortex are listed in Table 20.1. As a summary, the retro-Rolandic portion of the cerebral hemispheres is chiefly involved in the processing of sensory information about the outside world and about the motor acts being performed by the individual. Both of these, but particularly the latter, require the integration of sensory information of different modalities (visual, somatosensory, and so on). Lesions in the “primary” sensory areas cause loss of a specific sensory modality. These primary areas are listed in Table 20.1 (Fig. 20.5). The cortex surrounding the primary sensory areas processes the modality-specific information and integrates it with information from other sense organs and information about the physiologic milieu of the individual.
FIG. 20.4. Brodmann parcellation of the lateral (A) and medial (B) aspects of the cerebral hemispheres according to the specific cytoarchitecture of each area [62]. Primary sensory areas are in darker blue, primary motor in darker red, and limbic structures in yellow hues.
TABLE 20.1 Cerebral Hemispheric Connections
TABLE 20.1 Cerebral Hemispheric Connections (Continued)
Simply stated, the cortex adjacent to the primary sensory areas (secondary sensory areas) processes unimodal sensory information, often keeping a somatotopic organization, whereas the cortex lying between the different secondary sensory areas (tertiary sensory cortex) integrates multimodal sensory information. For instance, somatosensory information from spinobulbar-thalamic pathways reaches somatotopically the postcentral gyrus (Fig. 20.6), which projects somatotopically to the superior (arm and leg) and inferior (head) parietal lobules. Somatotopic information is integrated with auditory and visual information in the inferoposterior portions of the inferior parietal lobule (angular gyrus) [314] and with visual and vegetative information in the posteromedial portions of the parietal lobe (precuneus). Lesions of the primary somatosensory area result in sensory loss, whereas lesions in the multimodal association areas result in motor performances that show the lack of multimodal integration. For instance, bilateral lesions of the posterior portion of the superior parietal lobule give rise to impairment of hand movements under visual guidance [98].
The activation of primary, secondary, and multimodal sensory areas is highly stimulus-specific and task-specific. As an example, the left posterior superior temporal gyrus is activated by acoustic changes in speech as well as nonspeech sounds, whereas the left supramarginal gyrus, a multimodal association area, is more specifically engaged in the detection of changes in phonological units, a task that is multimodal [80]. Attention to complex or nuanced sensory tasks, like detecting a specific target syllable, enhances sensory performance and requires more cortical activation [247,494]. It is likely that this activation is accomplished through an attentional network described below in more detail under Disturbances of Attention [339]. Cortical areas of the retro-Rolandic brain that “gate” the activation of primary and secondary sensory areas are located around the intraparietal sulcus and in the temporo-occipital region [130,163,231]. Prefrontal, insular and cingulate cortex also modulate the activation of sensory areas [88,130,402].
FIG. 20.5. Distribution on the lateral (A) and medial (B) aspects of the cerebral hemispheres of the five fundamental types of cortex shown in Figure 20.3. Type 1 (pyramidal) is depicted in white and type 5 (granular) is depicted the darkest. Note that the primary sensory areas, including the auditory (transverse temporal gyrus of Heschl), visual (calcarine cortex), and somesthetic (postcentral gyrus) areas have granular cortex. Association cortex sprawls among them. (Corresponds to Figures 70 and 71 from von Economo [488].)
The pre-Rolandic portion of the hemispheres contains programs concerned with planning, initiation, and execution of movements. The mesial frontal cortex (cingulate gyrus, supplementary motor area) is closely linked with the reticular activating system and the limbic lobe. It appears to mediate the drive to move in a meaningful direction (cortical attention). Although seemingly alert, patients with large bilateral lesions in this region remain motionless and mute (akinetic mutism) [275]. From the limbic system, information about past events and their bearing on the well-being of the individual reaches the anterior portions of the frontal lobe, where it is integrated with sensory information from the thalamus and from the multimodal association areas of the hemisphere. Thus, the best course of action within a temporal framework is delineated. The frontal cortex rostral to the precentral gyrus mediates complex motor programs, which are elicited under the “command” of the mesial frontal region and executed by way of the subcortical nuclei (basal ganglia, brainstem nuclei) and primary motor cortex. The primary motor cortex plays a greater role in the execution of fine, distal movements, whereas axial movements, such as walking, are mediated, to a greater extent, by subcortical structures. The cerebellum and the sensory nuclei of the brainstem, including the vestibular complex, provide the motor system with essential feedback information.
This brief introduction has attempted to highlight the main framework of the incredibly complex structure of the cerebral hemispheres, which is still far from clear. Some understanding of the anatomic structure facilitates the identification of the most likely location of a cerebral hemispheric lesion based on its clinical consequences.
Symptoms and Signs Caused by Cerebral Hemispheric Lesions
Lesions of the phylogenetically most recent part of the central nervous system differ in their manifestations from those that affect more primitive levels. Thus, the greater plasticity of the hemispheres, mediated by the large number of cortical neurons, and the existence of redundant pathways result in less pronounced deficits with lesions that, had they affected a similar volume of the brainstem or spinal cord, would have caused a major motor or sensory disturbance [89,144]. Plasticity and redundancy also explain that destruction of cortical areas that are activated by a specific task on functional neuroimaging may not cause lasting clinical findings related to that task unless the lesion is relatively large [145,482].
The neurologic deficit caused by cerebral hemispheric lesions tends to be more inconsistent than deficits related to lesions in the lower echelons of the nervous system. Reduced attention, which may be related to the time of the day, a noisy environment, or lack of adequate stimuli, is only one of the many factors that can influence the outcome of a given neurologic examination. Typical of partial multimodal deficits, such as aphasia, is that the patient comes up periodically with the correct performance, leaving the junior clinician wondering about the extent of the patient’s deficit. Repeated interviews minimize this problem and allow the examiner to arrive at a much more accurate picture of the nature, and therefore the localization, of the patient’s disturbance. Of course, the luxury of repeated examinations is unaffordable when a quick decision has to be reached in an emergency management situation, but it should be available when planning the long-term management and rehabilitation of these patients.
When obtaining the history pertinent to a cerebral hemispheric lesion, the examiner must realize that the patient is often unaware of the extent of the deficit, particularly when it involves complex (multimodal) behavior (aphasia, apraxia). Patients with right-hemisphere lesions tend to be impervious to their deficit more often than patients with left-hemisphere lesions. Anton syndrome, in which the patient denies an otherwise obvious blindness that is related to a cortical parietooccipital lesion, is only one instance of such lack of insight. Something similar occurs with other hemisphere-related sensory deficits. Their extent and quality are less precise than when the sensory loss is caused by lesions in more elementary structures of the nervous system, such as the brainstem or a sensory peripheral nerve. Most patients with an ulnar neuropathy can outline a precise area of numbness in the medial aspect of their hands. By contrast, a patient with a hemispheric lesion, even affecting the primary somatosensory cortex of the postcentral gyrus, may have a difficult time localizing the area of sensory loss. This characteristic of hemispheric lesions, combined with the difficulty of eliciting all the deficits in a short interview, make it often necessary to obtain information from people who know the patient well in order to localize a cortical lesion correctly.
For adequate localization, multimodal deficits, such as alexia, must be analyzed. The patient may be unable to understand written material because his saccades to the left side are incomplete, leading him to miss the beginning of words and sentences (right frontoparietal lesion), or because he cannot grasp the meaning of an array of strokes that make up a written word (left occipital lesion). It behooves the examiner to go beyond the obvious disturbance and try to understand its structure and the primary defect responsible for it.
The same function is represented in various areas of the cortex or even in contralateral hemispheres in different patients. This individual variability makes the localization of hemispheric disease particularly taxing. The most common anatomic correlations of clinical signs and symptoms are described below, but any attempt to pinpoint the exact square centimeter of the cortex that accounts for a deficit in a particular patient is a futile endeavor. Likewise, identification of the area of the cerebral hemispheres most likely to be injured in the context of a set of symptoms and signs does not mean that the function lost “is localized” in that area of the brain. Most cortical functions are subserved by extensive networks. Functional neuroimaging may be used to help define some of the functions likely to be impaired in an individual if a part of the cortex is lesioned, for instance, in the course of surgery to remove a tumor or an epileptogenic area [288,396].
Because most hemispheric functions are subserved by extensive networks, often complementary and redundant, single lesions may be clinically silent and become symptomatic when additional lesions impair the function of the network [483]. Multiple lesions may occur simultaneously or separated by any length of time.
For the sake of rationalization, the complex and fluid clinical picture displayed by patients with cerebral lesions has been compartmentalized into syndromes. It should be realized, however, that often the difference between syndromes is merely one of degree. A similar amount of tissue loss underlies the global aphasia that a patient has a few days after infarction and the Broca (motor) aphasia that eventually develops some months later. Also, initially the localization of the deficit is compounded not only by edema and metabolic abnormalities at the site of the lesion but also by dysfunction (diaschisis) of areas of the brain away from the primarily damaged region, particularly those heavily interconnected with it, such as the homologous area of the contralateral hemisphere [224,285].
Lesions that affect the same portion of the cerebral hemispheres may present very different clinical pictures depending on the tempo and nature of the damage. Sudden, “through” lesions, such as infarcts that destroy all the neurons in a portion of the cortex, tend to cause a more severe deficit than tumors that slowly infiltrate the same area of the brain. For instance, small infarcts often cause aphasia, but tumors have to be quite large before they cause an aphasic syndrome. Weakness may be rather profound after a small infarct, but a tumor seldom causes severe weakness until it extensively involves a cerebral hemisphere. Thus, localization for diffuse lesions is less accurate. Because of the plasticity of the hemispheres, however, as time elapses after an acute, “through” lesion, its clinical manifestations may resemble those of an infiltrative lesion of the same area. Rather than being restricted to a lobe or gyrus, many pathologic processes (e.g., Alzheimer disease, encephalitis) affect the hemispheres in a diffuse or disseminated fashion. A combination of deficits, which are predominantly manifestations of bilateral damage to the multimodal association cortex, then constitutes the clinical presentation. In these instances, neuroimaging findings are particularly helpful to try to correlate functional deficits with regional brain anatomy or function. For instance, the clinical varieties of primary progressive aphasia correspond to atrophy of relatively specific areas of the left perisylvian cortex [189].
Cortical plasticity results in a more complete recovery from elementary neurologic deficits, such as weakness or numbness, although more complex motor or sensory deficits may remain. At the bedside or in a quick office visit, these are more difficult to detect than elementary deficits, even though they may be very disruptive to the patient’s professional and family life [184]. Functional recovery following cortical lesions or developmental disorders is mediated by functional reorganization of the cortex, where a sound cortical area, either in the same or opposite hemisphere assumes the functions formerly subserved by the lesioned area [67,134,161,272,504]. Particularly with subcortical lesions, the newly responsible cortical area is generally larger than the original one, at least shortly after the insult, possibly reflecting a less efficient neuronal network [306]. The area of activation shrinks as training progresses and the deficit improves [493]. However in some instances activity in the contralesional hemisphere may seem to interfere with the functional recovery, suggesting the need of new rehabilitation paradigms [352].
Because cortical plasticity is mediated by extensive multisynaptic arrays, which are susceptible to metabolic disturbances that interfere with the elaboration and processing of neurotransmitters, toxic metabolic insults affecting the whole brain impair particularly the functions newly acquired in the process of “repair” of a focal lesion [149]. Thus, they may bring about again a clinical deficit that had been well compensated. For instance, a patient with a mild residual difficulty in naming objects following a large lesion of the dominant temporal lobe, which initially caused a severe sensory aphasia, may again become unable to understand conversational speech when she suffers a bout of pneumonia.
Lesions that affect the cortex selectively (e.g., hypoxic laminar necrosis) give rise to a clinical picture that differs from lesions circumscribed to the white matter (e.g., multiple sclerosis).
Characteristic of cortical lesions are (a) seizures and (b) multimodal motor and sensory deficits, such as aphasia and apraxia. Although subcortical lesions may cause aphasic symptoms, these are seldom as pronounced or long lasting as they are with cortical lesions.
Characteristics of white matter lesions are (a) weakness, (b) spasticity, (c) visual field deficits, (d) “pure” motor syndromes, and (e) urinary incontinence. Lesions that involve the white matter of the hemispheres cause symptoms that are referable to the cortical region giving rise to the white matter tract involved.
More than any other part of the nervous system, the cerebral hemispheres are amenable to lesion localization provided by neuroimaging, including computed tomography (CT) and MRI [325]. PET and single-photon emission computed tomography (SPECT) depict not only the primary anatomic lesion but also the metabolic or perfusion change in areas functionally related to it (diaschisis). The extent of changes on PET or SPECT often correlate better with the severity of clinical symptoms and signs than the CT or MRI abnormalities [282,340]. The clinical evaluation of a patient with a cerebral hemispheric lesion is still paramount for a lucid management plan. Lesions as common as brain infarcts may remain invisible on CT scan for some time after the ictus or may pass unnoticed altogether if they are restricted to the cortex or cause coagulative necrosis. Diffusion-weighted MRI depicts them earlier and provides information on the timing of the lesion [226]. Tumors are easily detected on CT scan or MRI, but disorders such as Alzheimer disease cause more subtle findings on these neuroimaging modalities. Although there are quite specific structural and metabolic changes in samples of Alzheimer patients, a single neuroimaging study still does not provide the diagnosis in the individual patient [321]. Cortical lesions giving rise to focal epilepsy, or white matter lesions in multiple sclerosis, are more often evidenced by MRI than CT, but they occasionally remain undiscovered [317,409]. Finally, a good understanding of the anatomic correlation of behavioral symptoms allows the clinician to correlate the neuroimaging findings with the presenting complaints, thus avoiding the mistake of managing as an active lesion one that bears no relation to the present illness (such as hydrocephalus in a patient with Alzheimer disease), or of having a false sense of security when a negative scan fails to disclose an active lesion (such as in multiple sclerosis).
Because the output of the brain is ultimately a motor output, no matter where in the cerebral hemispheres a lesion has occurred, the physician becomes aware of it by observing the patient’s motor performance. Such a motor performance depends on (a) the patient’s level of alertness, mediated by the ascending reticular activating system; (b) the ability to concentrate on a task (cortical attention); (c) the perception of sensory stimuli and of their relation to past experiences; and (d) the ability to carry out the sequence of movements that makes up the motor act itself, whether it be a handshake or an oral account of the current illness. Hemispheric lesions can disturb any or several of the last three steps. The resulting disorders are considered successively (Table 20.2).
Vegetative Disturbances
Hemispheric strokes, and particularly subarachnoid hemorrhage, may be attended by cardiac disorders, including Takotsubo cardiomyopathy, which is characterized by transient left ventricular apical ballooning [507]. These disorders have been associated primarily with insular cortex damage or damage of posterior circulation structures [507]. The correlation of automic disturbances, including for instance a tendency for gastrointestinal bleeding, with acute stroke is complex, as the large hemispheric strokes associated with these disturbances typically compress the hypothalamus and other subcortical areas, or, as in the case of subarachnoid hemorrhage, impair their metabolism [274]. Epilepsy is also associated with autonomic disorders. Asystole may rarely occur during temporal lobe seizures, which are more often accompanied by ictal bradycardia [424,472]. Pallor and ictal vomiting are characteristic of a type of childhood seizures with occipital or extra-occipital spikes (Panayiotopoulos syndrome) [70]. Ictal mydriasis has been described ipsilateral to a chronic hematoma spanning the subcortical insula, temporal lobe and hypothalamus [171].
Disturbances of Attention
Attention may be defined as the waking state in which sensory or mnestic information is selectively perceived, allowing the coherent performance of planned motor behavior. This selectivity is associated with unawareness of a great deal of irrelevant stimuli and memories. It results from active neural facilitatory and inhibitory processes taking place at various levels of the nervous system, from the peripheral sense organs to the cortex. Although attention requires a certain level of alertness, alertness is not always associated with attention. Such is the case of the akinetic mute state, in which the patient appears alert, yet lies immobile and mute, while his eyes dart in the direction of any novel stimulus. Alertness, which precedes attention, can be nonspecific (such as in the alerting reaction that occurs when a person adopts an exploratory, attitude to the immediate environment, becoming receptive to a great deal of stimuli) or specific (when the meaning of the most significant stimulus is recognized and alertness is specifically and steadily directed toward it). The latter is more properly called attention. Concerning its origin, attention may be “passive” (involuntarily triggered by external stimuli and basic drives) or “active” (voluntarily generated and directed). Active attention is mediated by a dorsal system including the superior parietal lobule and the superior portion of the dorsal prefrontal cortex [89]. Both hemispheres participate in active or goal-directed attention. By contrast, the system activated by external stimuli is lateralized to the right hemisphere, particularly in right-handed individuals [155]. Instead of being dorsal, as the active one, it involves cortex of the inferior parietal lobule, posterior portion of the superior temporal gyrus and inferior portion of the prefrontal cortex [89]. This alerting network seems to interrupt ongoing cognitive activity when a stimulus that might be important is detected. As the right hemisphere provides alerting mechanisms for both sides of the body, attentional impairment occurs more often and is more pervasive with right-sided hemispheric lesions. Finally, attention to and processing of information stored previously is carried out by a network that includes the precuneus, medial prefrontal cortex and medial temporal regions (Fig. 20.7) [65]. This network is currently called the default or resting network because it becomes inactivated by tasks that require attention to external stimuli. It has at least two components: the medial parieto-frontal cortex is more involved in processing self-relevant, affective decisions, while the medial temporal lobe subsystem becomes engaged when decisions involve constructing a mental scene on the basis of memory [12].
TABLE 20.2 Clinical Manifestations of Cerebral Hemispheric Lesions
Cortical alerting mechanisms are activated by brainstem structures. The mesencephalic reticular formation projects to the cortex in a diffuse polysynaptic fashion, with this projection likely traveling via the thalamus or basal forebrain [215,458]. Stimulation of the mesencephalic reticular formation is associated with arousal, bilateral destruction of the mesencephalic reticular formation results in coma, and unilateral lesions of the mesencephalic reticular formation result in contralateral inattention, probably due to unilateral hypoarousal of the hemisphere [215,497]. The mesencephalic reticular formation also facilitates the relay of sensory information to the cortex by inhibiting the nucleus reticularis thalami, which, in turn, projects to and inhibits the thalamic relay nuclei. Thus, the transmission of sensory data that are relayed through the specific thalamic nuclei to the cerebral cortex is enhanced by mesencephalic reticular stimulation (or behavioral arousal), and unilateral mesencephalic reticular lesions may cause neglect because the thalamic sensory nuclei are being inhibited by the nucleus reticularis thalami [215]. Dorsal mesencephalic lesions may cause contralateral visual “inattention” by interrupting uncrossed pathways from the superior colliculus to the dorsolateral prefrontal cortex [172].
FIG. 20.7. Default network. Areas of the brain that are most active when the subject is at wakeful rest and become less active when performing an activity focused on the external world. They are depicted on the lateral (A) and medial (B) aspects of the hemisphere. The main hubs of the default network are represented in red and the anatomical regions are labeled. Modified from Buckner RL et al [65], with permission.
The primary sensory cortex contributes importantly to attentional mechanisms, particularly by signaling striking or salient sensory stimuli [330,363]. Primary sensory cortical areas (e.g., for vision, Brodmann area 17) project to neighboring modality-specific cortical areas, for instance, in the case of vision, Brodmann area 18. Modality specific areas project in turn to multimodal association cortex of the parieto-temporal lobes. Multimodal sensory areas combine information from vision, hearing and somatosensory stimuli. Modality-specific association areas may detect stimulus novelty and, by corticofugal pathways that inhibit the nucleus reticularis thalami, potentiate thalamic relay of sensory information. Multimodal sensory areas may also be important in detecting stimulus novelty and significance. In contrast to unimodal association cortex, which projects to specific parts of the nucleus reticularis thalami and thereby gates sensory input in one modality, multimodal areas inhibit the action of the nucleus reticularis thalami in a more general fashion, providing further cortical arousal. The multimodal sensory areas also may project directly to the mesencephalic reticular formation to influence arousal, although some experiments show a more important role of modality-specific areas for attentional mechanisms [82].
Brain regions that participate in attentional mechanisms can be separated into two broad categories: activated sensory areas and the brain structures that activate them. Areas of sensory cortex are activated that are relevant in a given moment. For instance, when attending to the color of an object, the lingual gyrus, containing secondary visual association cortex is activated [58]. The activating structures form a network of distributed processing, with nodes that play a larger role in one or another area of attentional mechanisms. As Mesulam said in his earlier reviews and implies in his most recent, the inferior parietal lobule sculpts the subjective attentional landscape, whereas premotor areas of the frontal lobe plan the strategy for navigating it [339]. The cingulate gyrus detects conflicting processes during task performance that might be associated with errors [71,74]. It may inject into the attentional network the relevance for survival of a particular situation. A posterior temporo-occipital area seems to mediate the shift of the attentional focus across the visual scene [178]. Activation of these cortical areas requires input from the basal ganglia through the thalamus. In addition to relaying to the cortex sensory and basal ganglionic information, the thalamus plays a key role in attentional mechanisms, not only through the reticular nucleus but also through the pulvinar, heavily connected to the parietal and frontal attentional areas. All these anatomic structures, interconnected to each other, work in unison to provide the complex mechanisms involved in maintaining attention (Fig. 20.8). Lesions of any of the components of the resultant network or their interconnections, including the fiber bundles in the hemispheric white matter, can result in neglect [339]. For instance, disruption of the superior occipitofrontal fasciculus, a poorly known parietal-frontal pathway, causes neglect in humans [469]. Neglect resulting from unilateral posterior parietal lesions is characterized mostly by sensory extinction, whereas neglect associated with frontal or basal ganglionic lesions includes a disruption of exploratory and orienting movements toward the neglected hemispace [96,448].
Stimulus significance is determined by the needs of the organism. The limbic system, closely related to the hypothalamus and other areas important for the maintenance of homeostasis, and the frontal lobes, critical for the evaluation and planning of a future course of action, are important in stimulus processing. Not surprisingly, the inferior parietal lobe has prominent connections with the frontal lobe and cingulate gyrus [215,216,499].
Disorders of attention due to hemispheric lesions may affect the patient’s behavior toward events in one side of personal and extrapersonal space (hemi-inattention, or spatial inattention) or nonspatial cognitive functions.
UNILATERAL INATTENTION
Unilateral inattention or neglect is characterized by one or more of the following findings: (a) Hemi-inattention, which is the patient’s lack of orienting responses to unilateral novel stimuli (auditory, visual or tactile) in the absence of a primary sensory or motor deficit that could explain such behavior [215]. (b) Extinction on double simultaneous stimulation, tested most often with tactile or visual stimuli that the patient perceives on the affected side on single, but not on simultaneous stimulation of both sides. (c) Hemiakinesia or motor neglect, when the patient tends to direct all his or her activity to one hemispace. (d) Allesthesia, when contralesional stimuli are attributed to the ipsilateral side. Although frequently associated, these deficits result from damage to different portions of the attentional network, and therefore some but not others may be seen in a given patient [460]. Anosognosia, etymologically, lack of awareness of disease and, in this case, of neurological impairment is often seen with right hemispheric lesions [135]. However, it need not be associated with a neglect syndrome and often it is not [460]. Neglect may be primarily sensory (attentional defect) or primarily motor (intentional disorder or hemiakinesia) [215].
FIG. 20.8. Representation of some systems important in attention and arousal. NR = nucleus reticularis thalami; MRF = mesencephalic reticular formation; VPL = ventral posterolateral nucleus of thalamus; MG = medial geniculate; LG = lateral geniculate. (Adapted from Heilman KM, et al. [215])
Sensory Inattention. Sensory inattention may be unimodal (e.g., visual inattention), in which case stimuli of a specific sensory modality are less well perceived on one side. Most commonly, these patients have extinction to double simultaneous stimulation, failing to report stimuli delivered to the side contralateral to the lesion. This clinical finding may be explained because stimuli from one side of the body compete with stimuli from the contralateral side for cortical activation [150]. However, cortical activation of the affected hemisphere may be present even when the patient does not see a visual stimulus in the affected hemispace [395]. In a given patient, it may be difficult to distinguish between hemianesthesia or hemianopia and severe somesthetic or visual hemi-attention. Occasionally, visual inattention may be distinguished from hemianopia by changing the hemispace of presentation [270]. Thus, a patient who could not detect single stimuli presented in the left visual field when the eyes were directed straight ahead (midsagittal plane) or toward the left hemispace could detect stimuli in the same retinotopic position when the eyes were directed toward the right hemispace, so that the left visual half-field was in the right hemispace [270]. This patient had hemispatial visual inattention masquerading as hemianopia.
Unilateral lesions of primary cortex cause contralateral unimodal sensory loss, whereas lesions of unimodal association cortex impair the perception of contralateral versus ipsilateral stimuli of that modality. For example, lesions affecting areas 18 and 19 (the association areas of vision) in the parietooccipital region cause extinction of a contralateral visual stimulus on bilateral visual stimulation. Lesions in the anterior association areas of the parietal lobe (the association area for soft touch) cause contralateral extinction of double simultaneous tactile stimuli [215].
The other and more common variety of unilateral sensory inattention is multimodal. These patients neglect the hemispace contralateral to the lesion when performing complex tasks such as dressing, in which they may fail to cover the neglected side (dressing apraxia), or drawing, in which elements of a picture may be placed in an abnormal spatial relationship to one another (constructional apraxia). Patients with personal inattention may deny that their own limbs belong to them or may identify the examiner’s limb as their own.
Patients with spatial neglect may be able to detect contralesional visual stimuli and may not even have extinction but may fail to act on contralesional stimuli presented in space. They may fail to act in left hemispace (egocentric hemispatial neglect) or may fail to act on the left side of the stimulus (allocentric spatial neglect) [215]. Spatial neglect is tested by the line bisection test, by cancellation tasks (the patient is asked to cross out all lines presented randomly on a sheet of paper), or by having the patient copy drawings. Although spatial neglect is most often described in the horizontal plane (left spatial neglect), vertical (altitudinal) neglect (due to bilateral parietooccipital lesions with Balint syndrome) and radial neglect have been described [394,442].
Perhaps akin to allesthesia, involving in this case auditory stimuli is the “response-to-next-patient-stimulation syndrome” seen with right hemispheric stroke, in which patients responded to stimuli directed at other patients as if the stimuli were directed at them [52].
Contralateral inattention occurs most commonly with lesions of the inferior parietal lobule but may also occur with lesions of the temporoparietal-occipital junction, dorsolateral frontal lobe, cingulate gyrus, insular cortex, thalamus, and mesencephalic reticular formation [98,215,308,309,495–497]. These areas have shown activation in attentional tasks [74,130,178,231,446].
Lesions causing hemispatial neglect are similar to those causing inattention and extinction. Lesions of the right inferior parietal lobule are especially apt to cause this syndrome. The severity of neglect is increased with both the size of the lesion and the degree of prior diffuse cortical damage [298]. Sensory extinction and hemispatial neglect may also occur with lesions of the insula [309], striatum and internal capsule [211], or with lesions affecting the caudate nucleus, lenticular nuclei, and surrounding white matter tracts (i.e., lesions that disrupt the cortico-striato-nigral-collicular pathway) [298,412,480].
These disorders appear more readily and profoundly with lesions of the right hemisphere, which is nondominant for language. Electrophysiologic studies have indicated that the nondominant hemisphere mediates attentional mechanisms directed to both hemifields, whereas the left hemisphere is mainly concerned with the right hemispace [264]. This hypothesis has been further supported by clinical studies that have demonstrated that patients with right brain pathology are more likely to make ipsilateral attentional errors than patients with left brain lesions [502], although left-sided extinction has rarely been reported after left hemisphere lesions [426]. Bilateral dichotic auditory stimulation in commissurotomized patients evidences extinction of stimuli in the left ear only [345]. Patients with neglect from right hemisphere lesions are not only inattentive to their bodies but are also distracted by extracorporeal stimuli, especially on the right [315]. Thus, the right side of the brain is dominant for distributing attention across the extrapersonal world [215,339,502].
Hemiakinesia. Hemiakinesia (intentional neglect) is the expression of unilateral motor neglect, characterized by a disinclination to direct orienting and exploratory behaviors with the head, eyes, and limbs into the neglected hemispace [53,339]. The patient may not look toward one side of the space, even though he or she readily reacts to sensory stimuli coming from that space, or may not move the limbs contralateral to the lesion unless specifically asked to do so, then showing good strength. Sensory neglect results from right hemispheric lesions and generally involves predominantly the left hemispace, whereas hemiakinesia may result from lesions of either hemisphere, particularly those affecting the dorsal, goal-directed, attentional network [89]. Akinesia affects movements toward the contralateral hemispace. Rare cases of attentional errors to the ipsilesional space are more likely with basal ganglia or frontal lesions than with retro-Rolandic lesions [261]. However, as attention and intention are closely linked, lesions in many of the areas that induce inattention and extinction may also cause akinesia. For example, lesions in areas 6 and 8 of the medial and lateral “premotor area” of the frontal lobe may cause this syndrome, which is more common and pronounced with lesions of the right hemisphere [100,159]. The dorsolateral frontal lobe has reciprocal connections with unimodal and polymodal sensory association cortices and is an area of sensory convergence. Lack of multimodal sensory feedback may explain rare cases of hemiakinesia without sensory neglect from parietal lesions [474]. As discussed in Chapter 18, thalamic lesions affecting the nonspecific intralaminar nuclei (which project to the frontal lobe) may also cause motor neglect or akinesia [498]. Akinesia may result from lesions of the basal ganglia and ventral thalamic lesions. The basal ganglia project to the ventral thalamus, and this “motor” portion of the thalamus also receives connections from the nucleus reticularis thalami [215]. Thus, degenerative or other diseases of the basal ganglia, thalamus, limbic system, and frontal lobes may cause akinesia [215].
NONSPATIAL INATTENTION
Inattention may affect nonspatial behavior, such as the inability to concentrate on a task, with consequent motor and verbal impersistence. For instance, on instruction the patient cannot keep her arms up and eyes closed for more than a few seconds. Failure to keep the eyelids closed is a common manifestation of motor impersistence [114]. Patients with right-sided hemispheric lesions are more likely to have motor impersistence than those with left-sided lesions, and right frontal lesions are more commonly responsible than more posterior lesions [257]. Although patients are distractible, attending to all kinds of irrelevant stimuli, they also often return inappropriately to a previous motor or verbal performance (perseveration). Whereas distractibility to external stimuli is more often seen with right-sided lesions, left frontal or caudate lesions impair predominantly the ability to divide attention between two sources (detection tasks) and to focus attention on one source (Go/No-Go tasks) [180]. In the verbal sphere, these patients are laconic or even mute and may tend to repeat sentences spoken to them or near them (echolalia) and even to imitate gestures (echopraxia). Such disturbances are most often seen in patients with advanced Alzheimer disease, who have diffuse cortical damage, or with metabolic encephalopathies, which in addition impair the subcortical alerting mechanisms. When a focal cortical lesion is responsible, it usually affects the mesial aspect of both frontal lobes. Large lesions in this location cause akinetic mutism: a state of motionlessness and speechlessness with regular sleep-wake cycles. Medial diencephalo-mesencephalic lesions can also cause this syndrome. Behavioral changes, including short attention span, apathy, disinhibition, and affective disturbances, may occur with unilateral or bilateral caudate lesions, implying caudate modulation of prefrontal behaviors [42,335]. Inertia and loss of drive, with preservation of intellectual function, often associated with stereotyped activities with compulsive and obsessive behavior, have been described with bilateral basal ganglia lesions confined to the lentiform nuclei, particularly affecting the pallidum [42,282,461].
Perseverative behavior has been divided into three categories, each with its own anatomic correlate [416]:
1. Recurrent perseveration is a repetition of a previous response to a subsequent stimulus and is seen with left hemisphere, especially temporoparietal, lesions.
2. Stuck-in-set perseveration is an inappropriate maintenance of a category of activity and is seen with fronto-subcortical and mesolimbic lesions.
3. Continuous perseveration is an abnormal prolongation of a current activity and is seen with right hemisphere damage.
Inattention in dementing processes is also manifested in the form of abnormal verbal utterances known as intrusions [167]. An intrusion is the inappropriate recurrence of a response (or type of response) from a preceding test item, test, or procedure. Cholinergic deficiency plays a role in the genesis of intrusions in Alzheimer disease [167]. In this disorder, intrusions during free recall correlate with impaired activation of the right superior prefrontal cortex, whereas intrusions during cued recall seem to relate to impaired left anterior medial temporal activation [121]. Verbal intrusions have also been described with delirium [492]. Motor intrusions have been described in Parkinson disease [132].
Emotional Disturbances
The hypothalamus, periaqueductal gray, and several pontine and midbrain nuclei mediate some of the most primitive emotional responses, matching the ongoing metabolic variables with the parameters set for the individual species [103]. When a deviation occurs, or when the circumstances are ripe for an action that would favor the survival of the individual or the species, a preset behavioral response occurs in lower animals. Such relatively simple behavioral responses are modified in humans by phylogenetically newer structures, such as the neocortex [27]. Some cortical regions are involved in the recording and retrieval of stimuli that proved to be noxious to the individual (limbic cortex). Others allow the individual to communicate with other human beings semantically (language areas of the dominant hemisphere) or through facial expressions and other forms of “body language” (nondominant hemisphere). Still others mediate the complex balance of emotional responses needed for the survival and development of a social community (frontal lobes, temporal lobes?). Finally, the outward expression of emotion uses the motor system.
Therefore, emotions and their expression depend on the following factors:
1. The state of arousal of the individual (alertness) mediated by the reticular activating system, including some thalamic structures, and the medial frontal cortex.
2. Vegetative functions, mediated in part by periaqueductal gray and other brain stem regions, by the hypothalamus, and by limbic structures [103], as well as reward mechanisms mediated by a complex network, including dopaminergic systems and the striatum [112].
3. A previous-experience retrieval system (memory) mediated by the hippocampus and other portions of the limbic system.
4. The ability to perceive the affective component of various stimuli, such as a friendly face or a threatening utterance. Brain structures mediating this function are related to the sensory modality involved and to the type of emotional content. The amygdala plays an important role for visual stimuli, as well as for the threatening quality of other stimuli, such as auditory stimuli [190,490]. The mechanisms by which the amygdala plays this role may not be “sensory” in the usual sense of the word. A patient with bilateral amygdalar damage recognized the expression of fear in faces when directed to look at the eyes, facial feature that best indicates this emotional state [1]. The ventral anterior portion of the insula is specifically activated to faces showing disgust, not necessarily as a “sensory” area but perhaps as a “mirror” area [273]. Regarding language, aphasic patients with lesions in the left inferior parietal lobule and superior temporal gyrus, for example, are unimpressed when told “I will kill you” in a matter-of-fact tone but react to a threatening pitch of voice or an angry face [197]. In contrast, right parietotemporal-damaged individuals understand the semantic meaning of a verbal threat, but their perception of the emotional overtones that accompany the utterance is impaired (sensory aprosodia) [176,405]. Right parietooccipital lesions lessen the ability to perceive facial expression [118]. Interpreting facial expression fails to activate right fronto-parieto-occipital and orbitofrontal cortex in people with alexithymia, a personal trait characterized by a reduced ability to identify and describe one’s own feelings [252].
5. The ability to evaluate properly the importance of internal and external stimuli for the survival and well-being of the subject [68,90,232]. For patients with bilateral orbitofrontal destructive lesions, most social nuances are trivial; however, they may go into a rage when some basic instinctive drives are not satisfied. By contrast, for patients with temporal lobe epilepsy even trivia become transcendental issues. Patients with small bilateral anterior cingulate (area 24) lesions may be unconcerned in the presence of painful stimuli [85,486]. Different types of emotional states map to quite specific subregions of the cingulate gyrus, as shown in Fig. 20.9. Patients with bilateral anterior temporal lesions have a bland affect. Lesions of the septal region cause enhanced irritability and rage reactions. Right orbitofrontal penetrating wounds may cause “edginess,” anxiety, and depression, whereas left dorsofrontal penetrating wounds cause anger and hostility [196]. Patients with epileptogenic foci in the left temporal lobe tend to be paranoid and have antisocial behavior, whereas those with right temporal foci show emotional extremes (elation, sadness) and denial [443]. Some patients with acute medial temporooccipital (i.e., the parahippocampal, lingual, and fusiform gyri) lesions become not only disoriented but also agitated and abusive (syndrome of agitated delirium) [333]. An acute confusional state and agitated delirium may also occur with lesions of the brainstem or thalamic reticular activating system, with lesions of the medial frontal lobe, and with right middle cerebral artery infarction [349].
6. The ability to express emotion, which requires more than a grossly intact motor system. Right frontal hemispheric lesions may cause impairment of the voluntary emotional intonation of speech (motor aprosodia) [405]. Lack of voluntary control of the emotional expression may adopt another form, namely, accentuated emotional expression, to the point of irrepressible laughing or crying unaccompanied by the corresponding inner feeling. Pathologic laughing or crying results from bilateral internal capsular lesions that also involve the basal ganglia; from lesions in the substantia nigra, cerebral peduncles, and hypothalamus; and from pronounced involvement of the corticobulbar fibers, such as occurs in severe suprabulbar amyotrophic lateral sclerosis [386]. Pathologic laughing requires a higher level of integration than pathologic crying. Pathologic laughing may rarely herald an acute basal ganglia lesion, brainstem stroke, or left carotid infarction (fou rire prodromique) [491].
FIG. 20.9. Cingulate emotion processing. Depicted on the medial aspect of the brain is a summary of studies showing peak activation sites during three simple emotions. Note that they occur in the cingulate gyrus with rather specific topography [486].
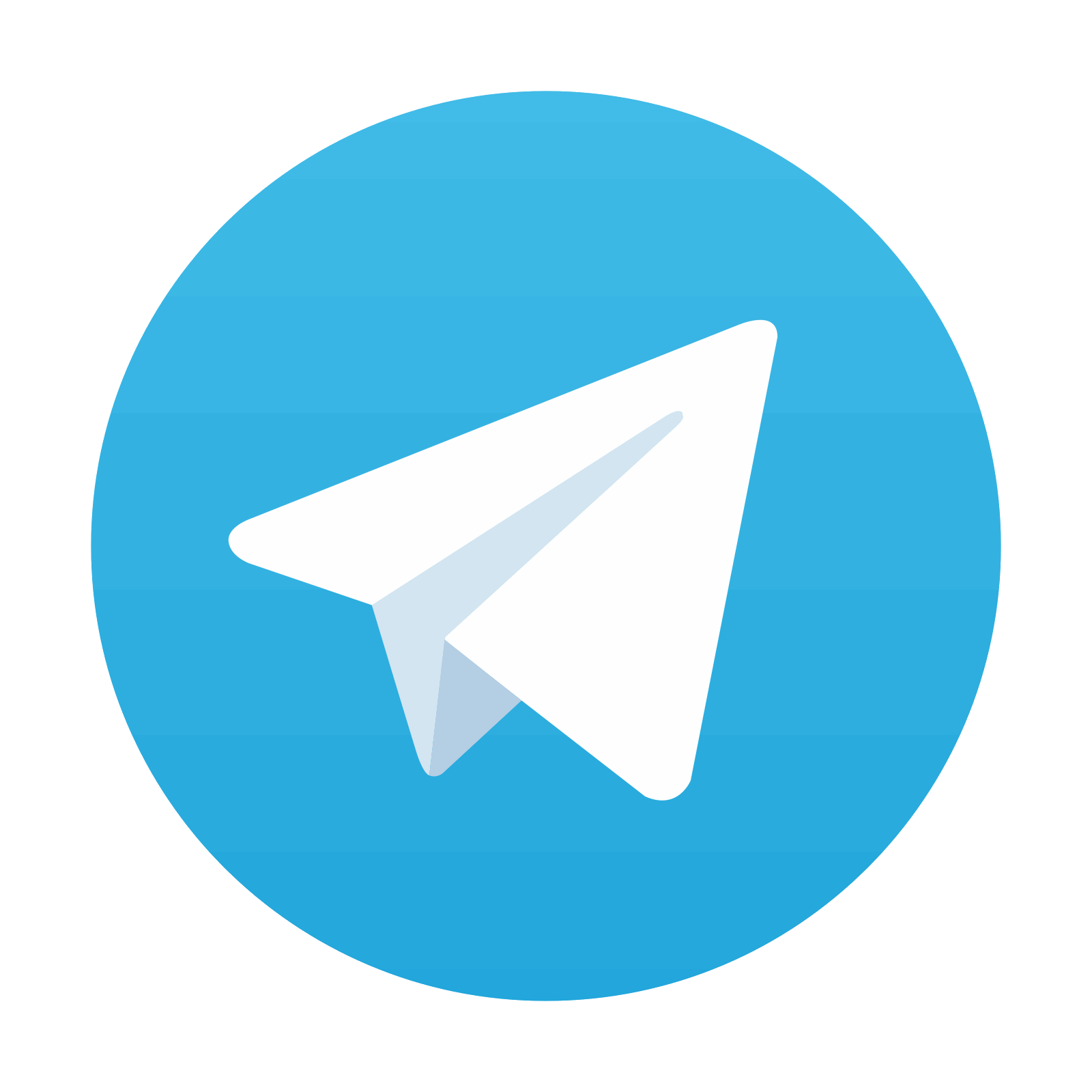