The Optic Nerve
Optic nerve function is tested by examining the various modalities of vision: the visual acuity, the visual fields (VFs), and special components of vision, such as color vision and day and night vision. The optic nerve is the one cranial nerve that can be visualized directly, and no neurologic, or indeed general, physical examination is complete without an ophthalmoscopic inspection of the optic disc and the retina.
Ideally, the eyes are examined individually. When testing acuity and color vision it is important to occlude the eye not being tested. Before performing the optic nerve examination, look for local ocular abnormalities such as cataract, conjunctival irritation, corneal scarring or opacity, iritis, foreign bodies, photophobia, arcus senilis, glaucoma, or an ocular prosthesis. The presence of a unilateral arcus corneae with ipsilateral carotid disease has been reported. In Wilson disease (hepatolenticular degeneration) a yellowish-orange brown coloration 1 mm to 3 mm wide (Kayser-Fleischer ring) may be seen around the rim of the cornea, more easily in light-eyed individuals. It is due to copper deposition in the posterior stroma and in Descemet’s membrane. It is best seen with a slit lamp. Cataracts may be present in patients with myotonic dystrophy, certain rare hereditary conditions with disturbed lipid or amino acid metabolism, and in many other conditions.
VISUAL ACUITY
Visual acuity charts, such as the Snellen chart, consist of letters, numbers, or figures that get progressively smaller, and can be read at distances from 10 ft to 200 ft by normal individuals (Figure 9.1). The difference between near and distance vision and between vision with and without correction are points of primarily ophthalmological interest. For neurologic purposes, only the patient’s best-corrected visual acuity is pertinent. Refractive errors, media opacities, and similar optometric problems are irrelevant. Acuity is always measured using the patient’s accustomed correction.
For distance vision measurement in the United States, a Snellen chart is placed 20 ft from the patient; at that distance there is relaxation of accommodation, and the light rays are nearly parallel. The eyes are tested separately. In countries using the metric system, the distance is usually given as 6 m. The ability to resolve test characters (optotypes) approximately 1-in high at 20 ft is normal (20/20 or 6/6) visual acuity. These characters subtend 5 minutes of visual arc at the eye; the components of the characters (e.g., the crossbar on the A) subtend 1 minute of arc. The acuity is the line where more than half of the characters are accurately read. If the patient can read the 20/30 line and two characters on the 20/25 line, the notation is 20/30 + 2. By conventional notation, the distance from the test chart, 20 or 6, is the numerator, and the distance at which the smallest type read by
the patient should be seen by a person with normal acuity is the denominator. An acuity of 20/40 (6/12) means the individual must move in to 20 ft to read letters a normal person can read at 40 ft. This does not mean the patient’s acuity is one half of normal. In fact, an individual with a distance acuity of 20/40 has only a 16.4% loss of vision.
the patient should be seen by a person with normal acuity is the denominator. An acuity of 20/40 (6/12) means the individual must move in to 20 ft to read letters a normal person can read at 40 ft. This does not mean the patient’s acuity is one half of normal. In fact, an individual with a distance acuity of 20/40 has only a 16.4% loss of vision.
Since few neurology clinics, offices, or hospital rooms have 20-ft eye lanes, testing is commonly done at a closer distance. Neurologists frequently assess vision with a near card (see Toolkit). Though examination of distance vision is preferable, the requisite devices are generally not at hand. There are pocket cards designed for testing at 6 ft, a convenient distance that usually
eliminates the need for presbyopic correction (see Toolkit). Near vision is tested with a near card, such as the Rosenbaum pocket vision screening card, held at the near point (14 in or 35.5 cm). Good lighting is essential. A penlight shone directly on the line being read is useful for bedside testing.
eliminates the need for presbyopic correction (see Toolkit). Near vision is tested with a near card, such as the Rosenbaum pocket vision screening card, held at the near point (14 in or 35.5 cm). Good lighting is essential. A penlight shone directly on the line being read is useful for bedside testing.
If the patient cannot read the 20/200 line at 20 ft, the distance may be shortened and the fraction adjusted. Ability to read the line at 5 ft is vision of 5/200, equivalent to 20/800. Vision worse than the measurable 20/800 is described as counts fingers (CF), hand motion (HM), light perception (LP), or no light perception (NLP). The average finger is approximately the same size as the 20/200 character, so ability to count fingers at 5 ft is equivalent to an acuity of 20/800.
When a patient has impaired vision, an attempt should be made to exclude refractive error by any available means. If the patient has corrective lenses, they should be worn. In the absence of correction, improvement of vision by looking through a pinhole suggests impairment related to a refractive error. Commercial multi-pinhole devices are available. A crude pinhole is included in the Toolkit. A substitute can be made by making three or four holes with a pin in a 3 X 5 card in a circle about the size of a quarter. The multiple pinholes help the patient locate one. The patient should then attempt to read further down the acuity card through the pinhole. The pinhole permits only central light rays to enter the eye. These are less likely to be disrupted by refractive errors such as presbyopia and astigmatism. If a pinhole was used, make some notation, such as 20/20 (ph). If the visual impairment is due to a neurologic process, such as optic neuritis (ON), vision will not improve with a pinhole. Under some circumstances, such as with opacities in the media (e.g., cataract), vision may get worse with pinhole.
COLOR VISION
Color blindness (achromatopsia) is an X-linked condition present in about 3% to 4% of males. Disturbances of color vision may also occur in neurologic conditions. Loss of color vision may precede other visual deficits. Color deficits may be partial or total. Color plates or pseudoisochromatic plates (Ishihara, Hardy-Ritter-Rand, or similar) formally and quantitatively assess color vision. Having the patient identify the colors in a fabric, such as a tie or a dress, can provide a crude estimate. The Toolkit contains some screening plates for color vision.
In neurologic disease, red perception is usually lost first. Desaturation to red, or red washout, describes a graying down or loss of intensity of red. The bright red cap on a bottle of mydriatic drops is a common test object. The patient compares the brightness or redness in right versus left hemifields, temporal versus nasal hemifields, or central versus peripheral fields. No right/left or temporal/nasal desaturation to red occurs normally. Red does normally look brighter in the center of the visual field than off center; reversal of this pattern suggests impairment of central vision. Patients may also compare the brightness or intensity of an examining light in one eye versus the other. A diminution of brightness on one side suggests optic nerve dysfunction; its significance is the same as for red desaturation.
THE VISUAL FIELDS
The VF examination is a very important and, unfortunately, often omitted part of the neurologic examination. The VF is the limit of peripheral vision, the area in which an object can be seen while the eye remains fixed. Macular vision is sharp. Peripheral images are not as distinct, and objects are more visible if they are moving. The normal VF extends to 90 degrees to 100 degrees temporally, about 60 degrees nasally, 50 degrees to 60 degrees superiorly, and 60 degrees to 75 degrees inferiorly. The field is wider in the inferior and temporal quadrants than in the superior and nasal quadrants (Figure 9.2). With binocular vision, the VFs of the two eyes overlap except for the unpaired temporal crescent extending from 60 degrees to 90 degrees on the horizontal meridian, which is seen by one eye only. The monocular temporal crescent exists because of the anatomy of the retina. The nasal retina extends farther forward, more peripherally, than the temporal. This is the true reason that the temporal VF is more expansive, not because the nose is blocking the nasal field.
Visual field examination results are most accurate in an individual who is alert and cooperative and will maintain fixation. Wandering of the eye impairs the evaluation. Crude assessment is possible even in uncooperative patients if the target is interesting enough (e.g., food or paper money). Fatigue and weakness may lengthen the latency between perception of the test object and the response to it, giving a false impression of VF deficit. Close cooperation, good fixation, and adequate illumination are essential for mapping of the blind spot and delineation of scotomas.
Clinicians use several different methods for visual field evaluation. The time and energy expended on bedside confrontation testing depends on the patient’s history and on the facilities available for formal field testing with tangent screen (central 30 degrees) or perimetry (entire field). Even sophisticated confrontation testing cannot approach the accuracy of formal fields.
The confrontation visual field exam can be tailored to the circumstances and done as superficially or as thoroughly as the situation requires. Sophisticated bedside techniques can explore the VFs in detail if circumstances warrant. If the patient has no specific visual complaint, and if other aspects of the history and examination do not suggest a field defect is likely, then a screening exam is appropriate. This can be accomplished rapidly and with great sensitivity using small amplitude finger movements in the far periphery of the VF. Recall that the VFs extend temporally to 90+ degrees. Extending elbows and index fingers, the examiner should position the fingers nearly directly lateral to the lateral canthus at a distance of about 24 in. Superficially, this appears to be a binocular examination, but, properly placed, the finger targets are actually in the unpaired monocular temporal crescent part of the visual field. With the targets positioned, make a small amplitude flexion movement with the tip of one index finger, perhaps 2 cm in amplitude. Have the patient “point to the finger that moves.” This language is more efficient than attempting a right-left verbal description where the patient’s and examiner’s rights and lefts are reversed. Stimuli should be delivered in each upper quadrant individually, then both together, and then similarly for the lower quadrants. Including bilateral simultaneous stimuli is necessary to detect subtle defects, which may be manifested only by extinction of one stimulus on double simultaneous stimulation. This technique of small finger movements in the far periphery in both upper and lower quadrants is an excellent screen; when properly done, even binocularly, this technique misses few VF defects. Always bear in mind that primary ophthalmological disorders such as glaucoma, diabetic retinopathy, and retinal detachment can also alter the visual fields.
With any hint of abnormality, or if the patient has or could be expected to have a visual problem, higher-level testing is in order. Examining monocularly, techniques include having the patient assess the brightness and clarity of the examiner’s hands as they are held in the right and left hemifields, in both upper and lower quadrants, or having the patient count fingers fleetingly presented in various parts of the field.
More exacting techniques compare the patient’s field dimensions with the examiner’s, using various targets—still or moving fingers, the head of a cotton swab, colored pinheads, or similar objects. Positioning the patient and examiner at the same eye level, and gazing eyeball to eyeball over an 18- to 24-in span, targets introduced midway between and brought into the VF along various meridians should appear to both people simultaneously in all parts of the field except temporally, where the examiner must simply develop a feel for the extent of a normal field (Figure 9.3).
For obtunded, uncooperative, or aphasic patients, paper money (the larger the denomination the better) makes a compelling target. Even if the examiner has only a $1 bill, suggest to the patient that it might be $100. The patient who can see will glance at or reach for the object. Children may respond to keys (no jingling), candy, or other visually interesting objects. Infants may turn the head and eyes toward a diffuse light within a few days after birth. Moving a penlight into the VF and noting when the patient blinks is sometimes useful. Checking for blink to threat—the menace reflex—provides a crude last-resort method. The examiner’s hand or fingers are brought in rapidly from the side, as if to strike the patient or poke him in the eye. The patient may wince, draw back, or blink. The threatening movement should be deliberate enough to avoid stimulating the cornea with an induced air current.
Testing central fields can include having the patient gaze at the examiner’s face and report any defects, such as a missing or blurred nose. Having the patient survey a gridwork (Amsler grid) while fixing on a central point is a sensitive method to detect scotomas (see Toolkit). Probing the central field with a small white or red object may detect moderate or large scotomas. With a cooperative patient, one can estimate the size of the blind spot.
By convention, visual fields are depicted as seen by the patient (i.e., right eye drawn on the right). This convention is backwards from most things in clinical medicine, and violations of the rule occur sufficiently often that labeling notations are prudent. When confrontation fields are not
adequate for the clinical circumstances, formal fields are done. These might include tangent screen examination, kinetic perimetry, or computerized automated static perimetry.
adequate for the clinical circumstances, formal fields are done. These might include tangent screen examination, kinetic perimetry, or computerized automated static perimetry.
VISUAL FIELD ABNORMALITIES
For neurologic purposes, visual field abnormalities can be divided into scotomas, hemianopias, altitudinal defects, and concentric constriction or contraction of the fields. Figure 9.4 depicts some examples of different types of field defects. Because of the anatomy and organization of the visual system, neurologic disorders tend to produce straight-edged defects that respect either the horizontal or vertical meridian, or have a characteristic shape because of the arrangement of the nerve fiber layer (NFL). Respect of the horizontal meridian may occur because of the horizontal temporal raphe and the arching sweep of NFL axons above and below the macula. This pattern is characteristic of optic nerve, optic disc, and NFL lesions. The vascular supply of the retina consists of superior and inferior branches of the central retinal artery, which supply the upper and lower retina, respectively. Vascular disease characteristically causes altitudinal field defects that are sharply demarcated horizontally. The calcarine cortex is organized into a superior and an inferior bank, and lesions involving only one bank may produce VF defects that respect the horizontal meridian. The vertical meridian is respected because of the division into nasal and temporal hemiretinas that occurs at the chiasmal decussation and is maintained through the retrochiasmal visual pathways.
Scotomas
A scotoma is an area of impaired vision in the field, with normal surrounding vision. With an absolute scotoma, there is no visual function within the scotoma to testing with all sizes and colors of objects. With a relative scotoma, visual function is depressed but not absent; smaller objects and colored objects are more likely to detect the abnormality. A positive scotoma causes blackness or a sense of blockage of vision, as though an object were interposed; it suggests disease of the retina, especially the macula or choroid. Positive scotomas are often due to exudate or hemorrhage involving the retina or opacity in the media. A negative scotoma is an absence of vision, a blank spot as if part of the field had been erased; it suggests optic nerve disease but can occur with lesions more posteriorly. With a negative scotoma the defect may not be perceived until a VF examination is done.
A scotoma can often be demonstrated on confrontation VF testing using small objects and carefully exploring the central fields, but they are best demonstrated by the use of the tangent screen. The physiologic blind spot is a scotoma corresponding to the optic nerve head, which contains no rods or cones and is blind to all visual impressions. The physiologic blind spot is situated 15 degrees lateral to and just below the center of fixation because the disc lies nasal to the macula and the blind spot is projected into the temporal field. The blind spot is enlarged in papilledema and ON.
Scotomas are described by their location or their shape. A central scotoma involves the fixation point and is seen in macular or optic nerve disease. It is typical for ON but can occur in vascular and compressive lesions (Figure 9.4A). A paracentral scotoma involves the areas adjacent to the fixation point, and it has the same implications as for a central scotoma. A cecocentral scotoma extends from the blind spot to fixation. It is usually accompanied by loss of all central vision with preservation of a small amount of peripheral vision, and it strongly suggests optic nerve disease (Figure 9.4B and Figure 9.5). Central, paracentral, and cecocentral scotomas are all suggestive of a process involving the papillomacular bundle. Any scotoma involving the blind spot implies optic neuropathy.
An arcuate scotoma is a crescent defect arching out of the blind spot, usually due to optic neuropathy with the brunt of damage falling on the fibers forming the superior and inferior nerve fiber layer arcades. A junctional scotoma is an optic nerve defect in one eye (central, paracentral, or cecocentral scotoma) and a superior temporal defect in the opposite eye. This is due to a lesion (usually a mass) that involves one optic nerve close to the chiasm, which damages the inferior nasal fibers from the opposite eye (Wilbrand’s knee) as they loop forward into the proximal optic
nerve on the side of the lesion (Figure 9.6 and Figure 9.4C). The temporal VF defect in the contralateral eye may be subtle and easily missed.
nerve on the side of the lesion (Figure 9.6 and Figure 9.4C). The temporal VF defect in the contralateral eye may be subtle and easily missed.
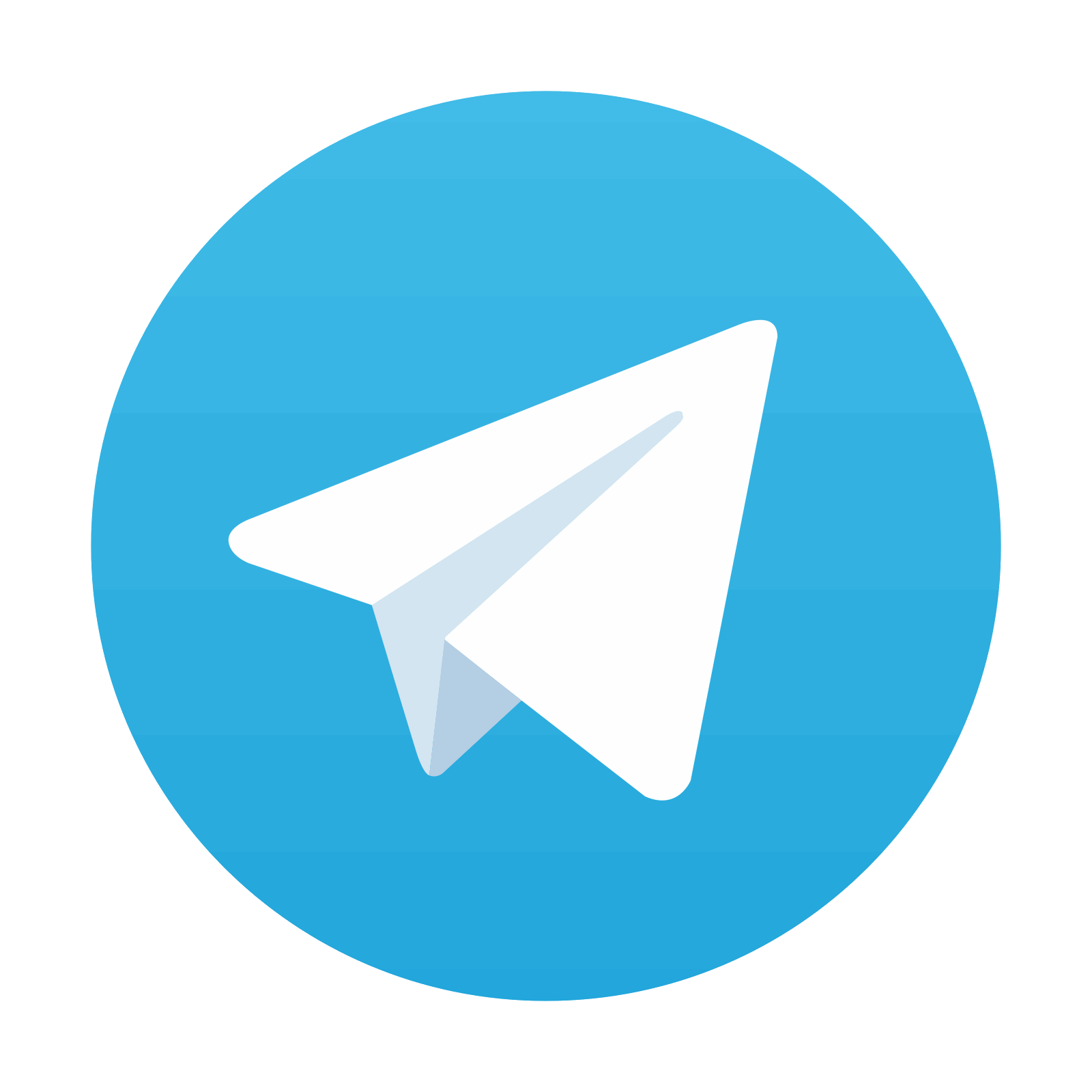
Stay updated, free articles. Join our Telegram channel

Full access? Get Clinical Tree
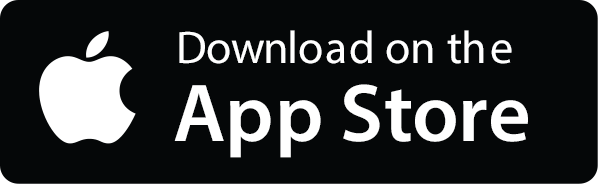
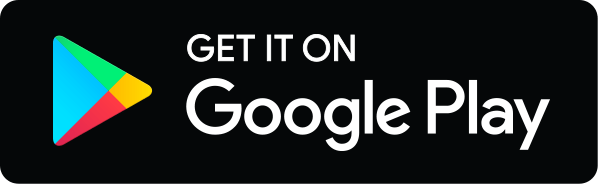