6 Therapy
Leon Sazbon and Giuliano Dolce
Medical Therapy
As treatment for the vegetative state (VS) has become more and more effective, the number of patients surviving in conditions that make them largely or totally dependent on others has steadily increased. Recently, concerns have been raised as to whether the traditional aims of preserving life, recovering consciousness, and management of complications in this patient group are indeed justified [1]. This process has been accompanied by a growing preoccupation with public expenditure and the optimal usage of limited health-care resources. As a result, there has been a shift from the conservation of life per se to an emphasis on quality of life. Attention is being redirected toward reducing the risks of secondary cerebral lesions in the early course of VS and creating conditions that allow maximum recuperation of nervous functions and social rehabilitation [1,2].
Recovery from the vegetative state depends on several factors: the severity of the brain damage; the degree of dysfunction; successful activation of the neural systems blocked by ischemia, edema, or diaschisis; reconstruction of systems through regeneration or substitution with related intact systems; and transfer of functions to distant neural systems. Giacino and Zasler [3] distinguished between two types of intervention. Standard interventions include the assessment and treatment of basic medical problems such as skin breakdown, autonomic system dysfunction, muscular contractures, gastrointestinal problems, cardiopulmonary and genitourinary complications and infections; prophylactic management of high-risk complications such as deep vein thrombosis, pulmonary embolism, and heterotopic ossification; and treatment of late neurological complications that inhibit neurological recovery, such as normotensive hydrocephalus and post-traumatic epilepsy and agitation, and of electrolyte disturbances and neuroendocrine disorders. Supplementary interventions include enriched environmental stimuli, such as playing audiotapes of voices of loved ones or videocassettes showing family members, hanging posters displaying the patient’s favorite pastimes, introducing contact with domestic animals or objects the patient would appreciate (for example, a basketball), use of sensory regulation or stimulation, administration of centrally active agents, and implantation of electrodes in deep brain structures. High-quality nursing is essential to optimize the chances of recovery.
The Quality Standards Subcommittee of the American Academy of Neurology [4] recommends that patients in the vegetative state receive appropriate medical, nursing, or nursing home care to maintain their personal dignity and hygiene.
Physicians and the family must determine the appropriate levels of treatment with regard to the administration or withdrawal of medications and other commonly ordered treatments; supplemental oxygen and antibiotics, complex organ-sustaining treatments such as dialysis, and administration of blood products and artificial hydration and nutrition.
Before any therapy is instituted, the risks must be weighed against the benefits. Drugs are often used in patients in the vegetative state, but since neuronal destruction is inevitable and irreversible, no specific pharmacotherapy exists. So far, the benefits have proven modest. There have as yet been no double-blind or placebo-controlled trials, and the results that have been reported are inconsistent. Indeed, medication may even have an adverse effect on the recovery rate. Giacino and Zasler [3] recommended that drug administration be kept to a minimum (preferably not polytherapy) to avoid inhibiting neural recovery or blurring the neurobehavioral presentation.
Types of Drug Used in the Vegetative State
Although none of the drugs available today can restore lost neural cells or influence one specific cerebral function over another less impaired one, some have been found to be of limited benefit. Those most worthy of consideration are the neurometabolic regulators and neurotransmitters, which activate cerebral metabolism or favor the transmission of nervous impulses in the synapses. The most extensive attention has been directed to levodopa or L-dopa, a dopaminergic agonist that has an activating effect on subcortical mechanisms involved in consciousness, behaviors related to the sleep-wake cycle, and motor regulation. By promoting the restoration of aminergic activities in the brain, L-dopa induces the recovery of damaged systems. Its use is supported by findings of a marked decrease in dopaminergic metabolites in the cerebrospinal fluid of patients in the vegetative state [2,5–10].
In addition, Vecht et al. [7] described changes in the metabolism of dopaminergic and serotonergic neurotransmitters in the chronic phase of the disease, while others authors [11–15] have reported a direct correlation between the severity of brain damage in the vegetative state and the level of homovanillic acid in cerebrospinal fluid.
In two clinical studies, Lal et al. [16] and Haig and Ruess [17] demonstrated the efficacy of levodopa/carbidopa (Sinemet) in the treatment of patients with severe brain damage. Using systemic administration of L-dopa, Higashi et al. [18] reported that at least two patients in the vegetative state in their series showed good recovery. Both responders were young adults with good cortical function, according to the initial electroencephalography (EEG) findings. Haig and Ruess [17] presented a similar anecdotal case of recovery from vegetative state in a 24-year-old man after 6 months of L-dopa treatment.
A similar action to L-dopa was reported for amantadine sulfate, which works presynaptically and postsynaptically, inducing the release of dopamine from its peripheral or central depots and elevating dopamine sensitivity in effector cells [19–22]. It reportedly clears neuroreceptor sites that have been blocked by false neurotransmitter substances [21] and is often used to antagonize the hypnotic effect of barbiturates. Zafonte et al. [23] reported on one patient in minimally responsive state who was successfully treated with amantadine five months after brain injury. In an interesting case report, Ross and Stewart [6] described the unsuccessful outcome of 2 months of treatment with methylphenidate (Ritalin) and carbidopa/levodopa in a patient in the vegetative state. Replacement of these drugs with lergotrile mesylate (amantadine) and bromocriptine, a dopamine agonist, led to progressive improvement and recovery of consciousness. Kugler [20] presented 16 patients in coma who were treated with amantadine intravenously and/or per os, with a remarkable brightening of the consciousness in six cases, while in another four the improvement was slight and transient. A successful response to bromocriptine alone was reported by Passler and Riggs [24], M�ller and von Cramon [25], Pulasky and Emmett [26], and others [27,28]. Other neurotransmitters or their precursors, such as atropine [29], physostigmine [9], and galanthamine, a cholinesterase inhibitor [30], could theoretically produce similar results.
Amphetamines, which release dopamine and norepinephrine from storage vessels in the central nervous system, have shown promise in the treatment of agitation. Agitation is manifested by thrashing of the extremities, truncal rocking, dislodging of catheters, yelling, combativeness, and attempts to get out of bed. Reyes et al. [31] reported that patients with agitation in the vegetative state have a good outcome. This has been our experience as well. Haloperidol may also be effective in reducing aggressiveness in these patients and increasing attention and alertness [32]. However, it blocks or disrupts dopamine receptors in the brain. So far, there is no concrete evidence that patients who are treated with haloperidol have a better outcome than those who are not. Jackson et al. [33] used tricyclic antidepressants to treat agitation. Antidepressants have also been used in other phases of coma [34], but there is little chance that they substantially influence either the level of consciousness or the contents of the vegetative state.
Hornstein et al. [35] advocated the use of dextroamphetamine to enhance recovery in severely brain-damaged patients. The mechanism of action of the sympathomimetic amines is still unclear. They appear to increase the release of norepinephrine from the presynaptic neurons and block the synaptic reuptake of both norepinephrine and dopamine, thereby increasing catecholaminergic activity. They excite not only higher nervous structures, but also lower centers and peripheral receptors [23,35]. This results in improved alertness, concentration, initiative, and motor activity [35], but it also warrants stringent control of patients. Kaelin et al. [36] and Plenger [37] used methylphenidate to improve attention in brain-injured patients. However, negative results with stimulants were reported by Speech et al. [38] and Gualtieri and Evans [39]. Uranova et al. [40] noted that in patients with vegetative state, stimulants may augment neuronal plasticity and regeneration.
Calliauw and Marchau [41] and Hakkarainen and Hakamies [42] suggested the use of piracetam, a cyclic derivative of γ-aminobutyric acid (GABA), in the treatment of the restlessness preceding recovery. Piracetam is known to increase the adenosine triphosphate (ATP) turnover of cortical nerve cells, thereby increasing their energy potential and normalizing the lowered polyribosome/ribosome ratio. This protects the cerebral cortex against hypoxia. These authors reported that patients given piracetam regained a state of consciousness significantly superior to that of patients given placebo. However, clinicians should beware of prescribing piracetam to patients with hepatic or severe renal dysfunction. Once treatment is initiated, the drug should not be withdrawn abruptly. Another agent, meclofenoxate hydrochloride (Lucidril), may improve cellular metabolism in the presence of diminished oxygen concentration [43].
The lazaroids (21-aminosteroids), which have no glucocorticoid activity, are thought to promote neurological recovery by inhibiting lipid peroxidation at the cell membrane level [44–46]. However, these assumptions were not supported by Huang et al. [47] in a clinical trial. Although several therapeutic attempts have been made with exogenous gangliosides (glycosphingolipids) on the assumption that they increase neuronal sprouting or plasticity [48–50], there is little evidence that their use in humans is effective [51].
Cytidine diphosphate choline (CDP-choline, citicoline) is a chemotherapy regimen used experimentally and clinically in Japan that can apparently be safely administered by intrathecal infusion in therapeutic doses [52]. Citicoline is believed to induce the synthesis of lecithin, passing through the cerebrospinal fluid (CSF) barrier and acting directly on nerve tissue. It targets sites around the cerebral hemisphere and brain stem [43], making it a feasible agent for study in the treatment of vegetative state.
With regard to anticonvulsants as treatment of the vegetative state, Chatham and Netsky [53] recently treated a series of 13 patients with severe brain injury with lamotrigine, which has been found in animal studies to block sodium channels and inhibit glutamate release for up to several weeks after injury. This is the only study so far with this drug.
McClain et al. [54] reported on exaggerated urinary zinc secretion with concomitant hypozincemia in severely brain damaged patients, and Sandstead et al. [55] observed reduced DNA and RNA in male rats exposed to intrauterine zinc deficiency. Zinc alters neuroexcitability, thereby modifying the affinity of neurotransmitter receptors. This could be clinically manifested by alterations of consciousness [56]. Young et al. [57] stated that zinc supplementation is associated with a decreased rate of mortality and improved rate of neurologic recovery in patients in the vegetative state.
Sedatives are often prescribed to treat epilepsy or spasticity. Great care must be taken to ensure that the drug being used has the lowest feasible sedative activity, combined with the highest potential effectiveness. Rigorous and unrelenting control is mandatory with regard to both drug choice and dosage. Giacino and Zasler [3] suggested that spasticity be managed by physiotherapy, positioning and tone-reducing casts, motor point or nerve blocks, followed by enteral drugs. Muscle stimulants should be avoided, particularly in the early stages of vegetative state, when sleep is qualitatively and quantitatively reduced, and hypotonia is present.
Among other methods tried, the results of hyperbaric oxygenation seem to be encouraging in cases of acute coma; however, not enough experience has yet been gathered in patients in the vegetative state [58,59].
Our experience suggests that drug therapy does not influence the recovery of consciousness or the final functional outcome. The current state of knowledge is poor, however, and further research is needed to determine the precise value of drugs.
Deep Brain Electrical Stimulation
The role of neurostimulation therapy is probably one of the most controversial issues in the management of VS and low-level consciousness. Its use is based on the rationale that stimulation of the ascending reticular system will activate the cerebral cortex and facilitate recovery of consciousness [3].
The first attempt at neurostimulation therapy in VS was reported by Hassler et al. [60] in 1969. In this study, stereotactic electrical stimulation was applied to the internal lamella pallidi and base of the contralateral ventroanterior nucleus in two patients. The protocol was repeated several times daily for 3 weeks. A slight transitory arousal was achieved. Several years later, Stern et al. [61] reported on one patient successfully treated with chronic stimulation of the reticularis polaris nucleus of the thalamus.
More recently, Katayama et al. [62] noted that stimulation of the mesencephalic reticular formation led to transient increases in pain-related P250 in four of eight patients in VS. A persistent increase in P250, with clinical improvement, was produced by chronic stimulation of the mesencephalic reticular formation or nonspecific thalamic nuclei for more than 6 months. Definite improvement with some degree of interpersonal communication was also reported by Cohadon and Richer [63] in 13 of 25 patients who underwent bipolar stimulation with stereotactically implanted electrodes in the centrum medianum parafascicularis complex. Treatment was applied for 2 months, 12 h daily. In another study of eight patients, thalamic stimulation was applied every 2 h in the daytime for 30 min each time, over a period of 6 months [64]. Four of the patients showed apparent activation of the ascending reticular system, with a consequent increase in cerebral blood flow and glucose metabolism in both the cortex and thalamus, in addition to some neural plasticity in the central nervous system. The authors suggested that the treatment needs to be applied for at least 3–4 months to achieve emergence from VS.
Dorsal column stimulation was applied by Kanno and colleagues [65] for 4 h a day in four patients, three of whom showed an improvement in clinical condition and EEG findings. This study suggested that the underlying mechanism of stimulation therapy is a change in cerebral blood flow.
Finally, one group assessed the effects of cervical spinal cord stimulation in a patient in VS using positron emission tomography (PET) and single-photon emission computed tomography (SPECT) [66]. They found that that this technique activates glucose metabolism and increases cerebral blood flow.
Information on deep brain electrical stimulation in VS is still lacking. Before definitive conclusions can be reached on the value of this intervention, further studies are needed to determine the optimal timing of treatment after injury and methods of patient selection, and to define appropriate outcome measures.
Surgical Therapy
Severe brain injury may be associated with complications or sequelae that require surgical treatment. One of the most well-known complications is ventricular enlargement, or ventriculomegaly. This term is sometimes confusing, as it is an anatomic or imaging description. The clinical condition is referred to as hydrocephalus, and it results from an imbalance between the production and absorption of cerebrospinal fluid. According to Jennett and Teasdale [67], post-traumatic ventricular dilatation can be divided into three types, according to cause:
• Ex vacuo hydrocephalus, due to wasting of the white matter
• Obstructive hydrocephalus, due to meningeal adhesions and circulatory CSF abnormalities
• Normal-pressure or communicating hydrocephalus, due to CSF malabsorption
The dilatation may be widespread, involving the whole ventricular system, or it may be concentrated in the lateral ventricular system (central atrophy) or cortical sulci (cortical atrophy). Radiologically, empty images of the porencephalic type may be observed on brain computed tomography (CT) scans, with the cavities communicating with CSF passages [2,68].
Post-traumatic hydrocephalus usually occurs between 1 and 3 months from the date of injury. Diagnosis is based on a combination of clinical imaging findings and physiologic data. The majority of patients (81%) in the series reported by Matshushita et al. [69] showed subarachnoid hemorrhage on the first CT scan. In obstructive hydrocephalus, the presence of blood breakdown products in the CSF interferes with the normal rate of CSF absorption by the arachnoid villi [70]. In 1965, Adams et al. discovered that certain patients developed hydrocephalus despite a relatively normal CSF pressure [71]. They hypothesized that the decreased rate of CSF formation reaches an equilibrium with the impaired rate of absorption. Katz et al. [72] concluded that in these cases, the hydrocephalus was apparently the result of impaired CSF flow and absorption round the cerebral convexities, or of arachnoiditis due to blood in the subarachnoid space.
Incidence of Hydrocephalus
The reported incidence of post-traumatic ventricular enlargement ranges widely, from 0.7% to 86% [72–78]. Using a CSF dynamics technique, Marmarou et al. [79] found a 44% incidence of ventriculomegaly in survivors of severe brain injury. However, only 20% of these patients had true post-traumatic hydrocephalus (PTH), and their outcome was significantly worse.
There are only a few reports on ventricular enlargement specifically in patients in patients in the vegetative state. The reported rates were 25% in the study of Tribi and Oder [80], 37% in the study by Kaliski et al. [81], and 51% in the study by Sazbon and Groswasser [82,83]. Kaliski et al. [81] explained the wide differences among studies by the use of different methodologies to determine ventricular size.
Diagnosis and Treatment
The detection and correction of true PTH pose a challenge to clinicians.
Communicating hydrocephalus may be managed by ventricular shunting. It is particularly important to distinguish it from ex vacuo hydrocephalus, which is less responsive to shunting and for which there is no real treatment [3,67,75]. At the same time, patient selection for shunting is complicated. Communicating hydrocephalus is impossible to recognize clinically, because the classic triad of dementia, incontinence, and gait disturbances is not applicable in unconscious patients [80] and is often absent [67]. In cases of PTH, the CT scan typically shows enlarged ventricles without cortical atrophy, with maximal expansion in the frontal horns, an increase in the content of interstitial fluid in the periventricular tissue, and fibrosis and flattening of the choroid plexuses [84]. However, the etiologic accuracy of CT is uncertain, and the diversity of results is not encouraging [85,86]. Gudeman et al. [74] used CT to diagnose PTH on the basis of enlargement of the frontal and temporal horns and the third ventricle in the presence of normal or absent sulci. They reported that the presence of a periventricular translucency with dilatation of the fourth ventricle is not conclusive, because these findings are observed in the majority of patients with brain atrophy and do not distinguish an atrophic process from true hydrocephalus with transventricular edema. In addition, the abnormalities in CSF flow provoked by subarachnoid hemorrhage develop slowly. According to Marmarou et al. [79], 45% of patients with PTH show the characteristic dynamic CSF changes on CT scan. These become evident in 93% of those with ventriculomegaly within 2 months and then reach a plateau. Therefore, using CT to check for progressive changes in ventricular size as the only indicator of communicating hydrocephalus is misleading. Serial measurements are also not trustworthy, because patients with cerebral atrophy will also show an augmentation in ventricular size [79].
Radionuclide cisternography, a popular diagnostic technique, shows reflux into the ventricles and delayed pericerebral diffusion, with no radioactivity in the region of the superior sagittal sinus. However, some researchers, such as Narrayan et al. [87], failed to find any value in cisternography. Another potential diagnostic tool is continuous drainage or aspiration of large amounts of CSF in the lumbar space.
Pneumoencephalography is not currently used, because it often worsens the clinical state.
Marmarou et al. [79] introduced a novel approach, using an intrathecal bolus injection of 2–5 mL saline solution at a rate of 1 mL per second to evaluate CSF dynamics, which is correlated with ventricular size and outcome. Prior to the study, baseline intracranial pressure (ICP) was measured, and the pressure volume index (PVI) and resistance to CSF absorption (RO) were calculated. CSF pressure was measured just before each injection, and maximal pressure immediately after and some time later. The authors found that patients with communicating hydrocephalus (9.3% of the total sample of brain-injured patients) were characterized by normal baseline ICP, high RO and progressively decreasing PVI.
Jennett and Teasdale [67] recommended that the decision to perform shunt procedures should be based on the clinical evolution – arrest or regression in clinical progress – combined with progressive ventricular enlargement with ballooning of the third ventricle, without a clear dominant brain atrophy or massive loss of brain tissue.
The rationale for shunting is based on the fact that the insertion of one-way valves set at the desired pressure allows the escape of CSF into the pleural or peritoneal cavity or into the cardiac atrium, leading to a decrease in intraventricular pressure to the predetermined level. Katz et al. [72] stated that shunting is generally successful if the lumbar CSF pressure is above 180 mmH2O and there is a progressive increase in ventricular size on the CT scan. They claimed that ventriculoperitoneal shunting is the treatment of choice, because of the ability of the peritoneal cavity to accept a large loop of tubing, combined with the relatively benign complications of the procedure and the ease of shunt revision. Ventriculoatrial shunting is indicated in the presence of abdominal abnormalities (previous extensive surgery, extreme obesity, etc.).
Outcome of Surgery
There is little information about the outcome of severe traumatic brain injury after shunt implantation. Usually, the ventricles decrease in size within 3–4 days, and clinical improvement occurs in the next few weeks [84].
Cardoso and Galbraith [85] reported a marked improvement in consciousness in 50% of 17 patients and a slight improvement in 25%. In the series reported by Sazbon and Grosswasser, 41% of patients recovered consciousness [83]. Improvement rates of 50% were also reported by Noubourg et al. [88] and Narrayan et al. [87]. More recently, Tribl and Oder [80] noted a 52% rate of improvement of various degrees at 3 months after shunting. The highest rates were reported by Matshushita [69] (77%) and Meyer et al. [89], Stein and Langfitt [90], and Vasshiloutis [91] (100% recovery of consciousness).
Graff-Radford [92] reported a 30% rate of complications, and Tribl and Oder [80] reported 41%. Complications of shunting include subdural hygroma or hematoma due to rupture of the bridging dural veins. This tends to occur when drainage is done too rapidly. Ventricular hemorrhage is also possible due to technological problems. Infection of the catheter or the valve can lead to encephalitis, peritonitis, pleural empyema, or sepsis [71,84]. The majority of infections occur 2–3 months after surgery [72], and the most common culprit organisms are Staphylococcus epidermidis and S. aureus. Treatment consists of removing the infected shunts and administering appropriate antibiotics. According to Katz et al. [72], infections occur in 5–39% of cases.
Another frequent complication is occlusion of the catheter tip by brain tissue or choroid plexus, resulting in revision of the shunt. In the study of Tribl and Oder [80], 31% of patients required shunt revision. These authors also reported the occurrence of epileptic seizures in 10% of patients immediately after shunt implantation and in 35% at a mean of 23 weeks later.
Stimulation Techniques
The last 20 years have witnessed a growing interest in sensory stimulation for the treatment of patients in the vegetative state. Together with the current high-technology approach of monitoring, machine support, and aggressive medical care, stimulation programs have found a place in the rehabilitation of acute and long-term unconscious patients. Their use is based on the rationale that both the degree and quality of recuperation is increased when attention is directed to the whole patient, and not to bodily well-being alone [93–96].
The concept of sensory stimulation programs for brain-injured patients was prompted by early studies on cognitive growth showing that children who were exposed to stimuli-enriched environments had better neurophysiological development than children who were not [97]. Later support was provided by experimental data from animals with cerebral lesions or after sensory deprivation [98–100]. In 1977, Greenberg et al. [101] and Hume et al. [102] independently reported that comatose patients respond to certain sensory stimuli. To explain this finding, Kater [103] suggested that head injury causes a disorganization of neuronal accessory pathways essential for cognitive functions and that sensory stimulation could activate those parts of the nervous system on which consciousness depends. This assumption was supported by the study by Walsh [99] confirming that the brain increases in both dimension and weight in a stimulus-rich environment. In addition, in the work of Finger and Stein [104], brain-injured patients exposed to a wide variety of stimulating experiences were found to develop an extensive functional neuronal circuitry that was lacking in restricted subjects. LeWinn and Dimancescu [105] argued that if sensory deprivation could change cerebral electric activity and produce widespread impairment of intellectual and perceptual processes in non-brain-damaged patients, we could expect that in brain-injured patients, the greater and richer the stimulation, the better the prospects for recovery.
Sensory stimulation in comatose patients is directed at the reticular system, which has a much higher sensory-stimulation threshold than other systems in the body, lowering it and thereby reducing deprivation levels. Because unconscious patients have short potential reactivity periods, the stimuli are given for very short periods of time, and efforts are made to avoid overstimulation. Apparently, better cognitive functioning is achieved when stimulation is focused on previously learned or recognized patterns [103].
Recently, Wood [106] introduced a new technique of sensory regulation (not stimulation) to prevent patient overstimulation and consequent habituation to stimuli. The technique involves limiting all sources of continuous ambient noise, such as television and radio, so that the environment is kept quiet and restful. The staff are instructed to regulate their activities and movements and to speak slowly and quietly to the patient, using certain key words (telegraphic speech). The author claimed that for best results, patients need to be exposed to long periods of silence interspersed with therapy sessions.
There are certain preliminary conditions for the success of sensory stimulation. The presence of a brain-stem lesions might block the path of the sensory stimuli [107]. In addition, prior to initiating the program, clinicians must ensure that patient use of sedatives and antiepileptics is minimized, because these drugs tend to lower sensory stimulation thresholds and reduce the efficiency of the environmental input [107].
Types and Techniques of Sensory Stimulation
There are two types of sensory stimulation, and it is important to differentiate between them. Environmental stimulation is based on the assumption that sensory deprivation should be prevented. Patients are subjected to ongoing, variable, unstructured stimuli of all types, which are presented in an unsystematic fashion [3,108]. Some activities for higher-functioning patients encourage passive participation. Environmental treatment strategies continue to be widely used in clinical practice, despite the absence of any published reports on their effectiveness. By contrast, structured stimulation introduces stimuli in a labor-intensive, systematic fashion [108]. Both types apply stimuli directed at all five senses: auditory (familiar voices, radio, music), visual (familiar members and friends, posters, familiar objects), tactile (personal clothing, blankets, stuffed animals, and real pets), olfactory (favorite odors, perfumes, foods), and gustatory (favorite flavors, sharp spices such as lemon, cinnamon) [103,105–107,109–114].
In 1990, Aldridge et al. [109] developed a form of stimulation therapy using music for the rehabilitation of comatose patients. The concept was based on the belief that the self is more than a corporeal entity and that human beings are organized not mechanically, but musically, “in a harmonic complex of interacting rhythms and melodic contours.” To maintain our coherence with the world, we must creatively improvise our identity, and “music therapy is the medium by which a coherent organization is regained, i.e., linking brain, body and mind.” This method yielded a range of reactions, from a change in breathing and fine motor movements to grabbing movements of the hands and turning of the head, to opening the eyes and the regaining of consciousness.
On the basis of this study, Sazbon (personal communication) recently applied six different types of melody in a sensory stimulation program. He used classical pieces broken into 15-min segments of quiet and constant listening periods. Each piece had a different tempo and rhythm. In each patient, the rhythm used was lower than the individual heart rate. This method is still in the experimental stage.
Wood [106] postulated that in normal individuals, a neural system can be “dishabituated” by exposure to an alternative stimulus or a more intense stimulus, either in the same or a different modality.
The effect of unimodal versus multimodal stimulation was examined using stimuli of known personal salience to the patients. Arousal was measured by the total frequency of eye-opening and spontaneous eye movements. Significant changes were noted immediately after treatment in four patients subjected to multimodal stimulation; in some of them, the effect continued to be observed as late as 28 months after injury [112]. Older patients showed a greater frequency of eye-opening. There was no relationship between time since injury or magnitude of response and mode of stimulation, although some individuals seemed to be particularly responsive to the unimodal type [106].
The frequency and duration of sensory stimulation vary considerably among the published reports [115,116]. For example, LeWinn [95] advocated at least 36 repetitions of the stimulation procedure per day, and Wilson et al. [113] used two treatment sessions daily 3 weeks apart, repeated with certain variants. The program used by Kater [103] was conducted for 5 min, 6 days a week for 3 months, whereas that of Mitchell et al. [111] was applied for 1 h twice daily, with no limit on duration. The music therapy program described by Aldridge et al. [109] lasted 12 min. By contrast, Feldman [107] used music stimulation for 25 min or 45 min, depending on the intensity and frequency of the sound, once or twice daily. These authors reported that after 20 min, a decrease in patient agitation could be observed (not reaching the level of relaxation), with a decrease in cardiac rhythm and arterial pressure. The reaction lasted 2–3 h after termination of the stimulus.
Assessment and Outcome
The response to treatment is measured by the reflex responses to auditory stimulation, voluntary motor response, phonation, recognition capability, and reduction of spasticity [107]. Sensory stimulation may provoke the appearance of a K-complex in the EEG. Many researchers have reported a positive influence of sensory stimulation programs in comatose patients [105,111,117–119]. Sisson [120] obtained a behavioral or alerting response on EEG in five treated patients, and Kater [103] reported a significant effect in 15 patients. Wilson et al. [113] noted a significant increase in arousal in 24 subjects immediately after treatment. The group who emerged from VS showed higher rates of spontaneous movements. However, these kinds of response may show up only during stimulation, and they do not imply clinical improvement [107]. Indeed, the effectiveness of sensory stimulation remains a strongly controversial issue [108,121], and there is as yet no evidence that sensory stimulation leads to a better outcome than conventional treatment [115]. The major problem is the lack of comparability between studies, due to wide differences in the intervals since the onset of brain injury, poor descriptions of cognitive status and of complications, and lack of information on patient selection. In addition, a great number of the studies conducted to date have been methodologically poor, with small samples, lack of a control group, and absence of peer review [111,117–120]. These factors are compounded by the difficulty, specific to this patient group, in distinguishing the effect of sensory stimulation from spontaneous recovery, possible effects of environmental stimuli, and effects of other treatments over which the coma therapist has no control [112,122]. Two other major areas that require clarification are the standards for identifying progress secondary to treatment and for decision-making with regard to continuation or reduction of treatment [96]. Some of these problems could be resolved by stringent examination of patients before and immediately after sensory stimulation sessions. Moreover, examiners must be made aware that they need to assess changes in awareness, not in arousal.
Several scales have been designed specifically to evaluate the response to sensory stimulation in patients in the vegetative state and to standardize assessments. These include the Coma/Near-Coma Scale erase [124], the Coma Recovery Scale [125], the Disability Rating Scale [123], the Sensory Stimulation Assessment Measure [116], the Western Neuro-Sensory Profile [126], the Rancho Los Amigos Levels of Cognitive Functioning [127], the Sensory Modality Assessment Rehabilitation Technique [110], and the Coma Exit Chart [128,129].
It should also be stressed that the results of sensory stimulation may be affected by many injury-related, treatment-related, or patient-related factors, as well as environmental factors. These include the type and severity of brain damage, time since trauma, and neurological status; the rate and duration of sessions; the patient’s age and educational level; and frequency of family visits. Studies by Rader et al. [96] indicated that some of these factors may be predictive of improvement over time. For example, earlier initiation of rehabilitation programs after injury can maximize the ultimate potential for recovery [111,130,131]. With regard to external factors, studies have shown that better results are achieved in patients who were exposed to an enriched environment prior to the head injury [132]. Kater [103] reported that patients with moderate scores on the Glasgow Coma Scale, of between 7 and 10, achieved the highest mean cognitive scores, similar to those in patients who came from an enriched environment. Accordingly, patients who have strong family support seem to do better than those who do not [132]; and today, clinicians tend to encourage visible active intervention by the family in the programs [96]. Finally, optimal responsiveness cannot be achieved without a high degree of interpersonal (verbal, emotional enthusiasm) relatedness on the part of the therapist.
Other Potential Advantages
Clinicians should not overlook the comfort that participation in a structured stimulation program provides to both staff and family members. It gives them the sense that everything possible is being done to help the patient. It also helps relieve the anxiety and feelings of impotence that are sometimes accompany continuous passive care [133]. Family members feel more control over the situation, and staff members enjoy greater job satisfaction. These in turn lead to a better level of care for the patient.
Conclusions
Despite the few positive findings reported in the literature, there is at present no concrete evidence that sensory simulation definitively alters or facilitates the course of recovery [108,125]. The International Working Party Report on the Vegetative State [134] recently concluded that sensory regulation by itself does not lead to mental recuperation, although it may enhance sensory conditions by regulating the environmental noise with alternate periods of treatment and nursing care. Wood [106] cautioned that the use of sensory stimulation is not endorsed by current knowledge on brain processes and sensory input in normal and damaged brains. This problem is exacerbated by the lack of standardization and poor methodology. Clinicians should therefore beware of raising unreasonable expectations among family and staff members.
Further, better-controlled studies are needed on this subject.
References
10. Sazbon L. Prolonged coma. Prog Clin Neurosci 1985; 2: 65–81.
14. Sachs E. Acetylcholine and serotonin in the spinal fluid. J Neurosurg 1957; 14: 22–7.
17. Haig A, Ruess J. Recovery from vegetative state of six months’ duration associated with Sinemet (levodopa/carbidopa). Arch Phys Med Rehabil 1990; 71: 1081–3.
47. Huang H, Patel PB, Salahudeen AK. Lazaroid compounds prevent early but not late stages of oxidant-induced cell injury: potential explanation for the lack of efficacy of lazaroids in clinical trials. Pharmacol Res 2001; 43: 55–61.
52. Ogashiwa M, Takeuchi K. Intrathecal pharmacotherapy in coma. Acta Neurochirurg 1976; 34: 37–44.
67. Jennett B, Teasdale G. Management of head injuries. Philadelphia: Davis, 1981.
68. Sazbon L. Prolonged coma. Prog Clin Neurosci 1985; 2: 65–81.
75. Guyot LL, Michael DB. Post-traumatic hydrocephalus. Neurol Res 2000; 22: 25–8.
76. Herrmann HD. Neurotrumatologie. Weinheim, Germany: VCH, 1991: 185–6.
77. Kampfl A, Franz G, Aichner F, et al. The persistent vegetative state after closed head injury: clinical and magnetic resonance imaging findings in 42 patients. J Neurosurg 1998; 88: 809–16.
84. Adams RD, Victor M. Principles of neurology. 4th ed. New York: McGraw-Hill, 1989: 507–9.
91. Vasshiloutis J. The syndrome of normal-pressure hydrocephalus. J Neurosurg 1984; 61: 501–9.
93. De Young S, Grass RB. Coma recovery program. Rehabil Nursing 1987; 12: 121–4.
97. Bruner JS. The course of cognitive growth. Am Psychologist 1964; 19: 1–15.
104. Finger SA, Stein DG. Brain damage and recovery. New York: Academic Press, 1982.
107. Feldman D. [Lecture presented at Ospedale Don Calabria, Verona, Italy, 1992.]
110. Gill-Thwaites H. The sensory modality assessment technique: a tool for assessment and treatment of patients with severe brain injury in a vegetative state. Brain Inj 1997; 11: 723–34.
129. Freeman EA. The catastrophe of coma: a way back. Buderim, Queensland: Bateman, 1987.
132. Clum NM, Ryan M. Brain injury and the family. J Neurosurg Nursing 1981; 13: 165–9.
134. Andrews K, Beaumont JG, Danze F, et al. International Working Party report on the vegetative state. London: Royal Hospital for Neurodisability, 1996 (http://www.comarecovery.org/pvs.htm).
< div class='tao-gold-member'>
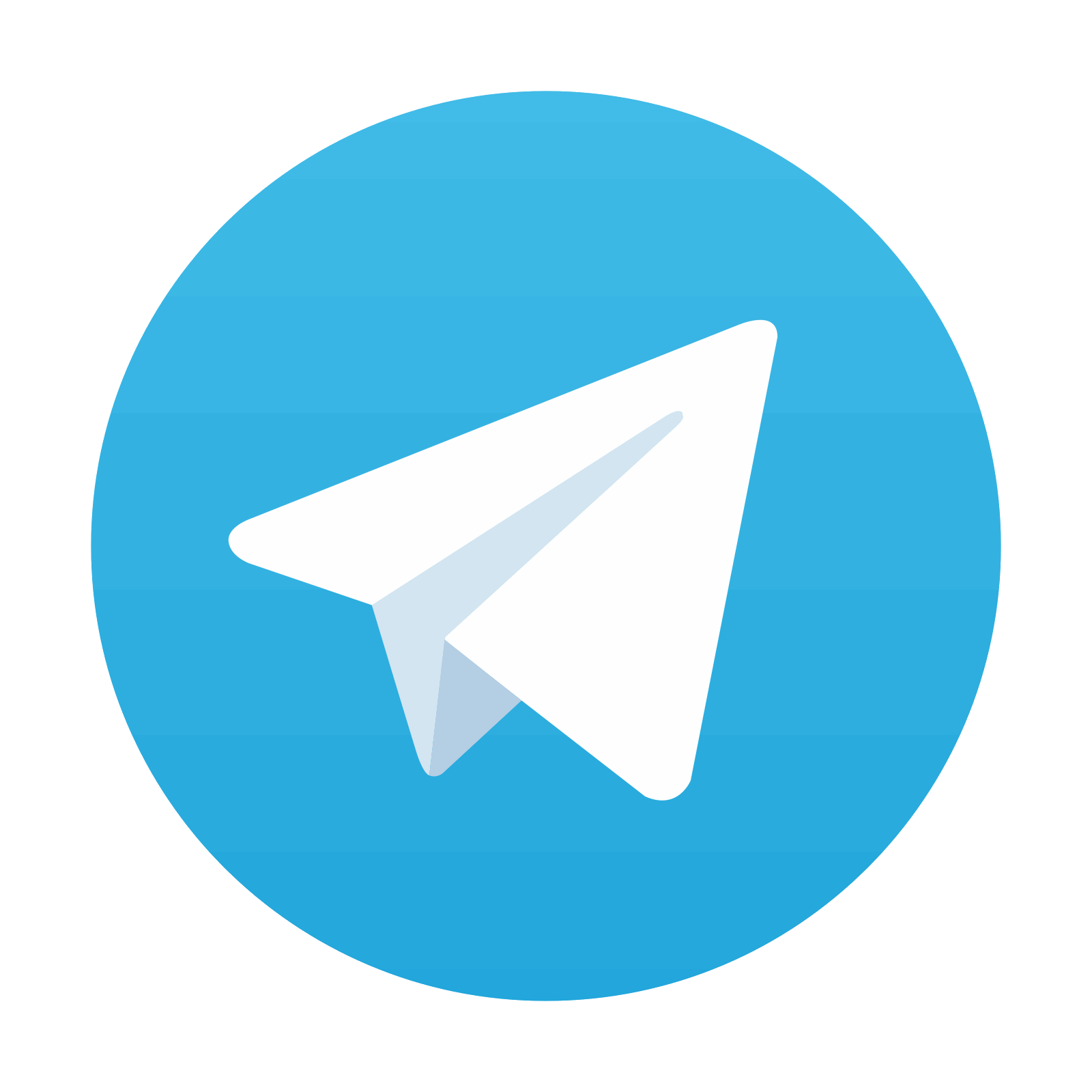