Lung and chest wall mechanics
Lung and chest wall compliance
Chest wall excursion
Lung volumes
Gas exchange
Oxygenation efficiency
Carbon dioxide removal
Regional lung function
Ventilation and perfusion distribution
Respiratory muscles
Inspiratory and expiratory force generation
Thresholds for respiratory muscle fatigue
Pulmonary hemodynamics
Pulmonary hypertension and cor pulmonale
Pulmonary host defenses
Cough effectiveness
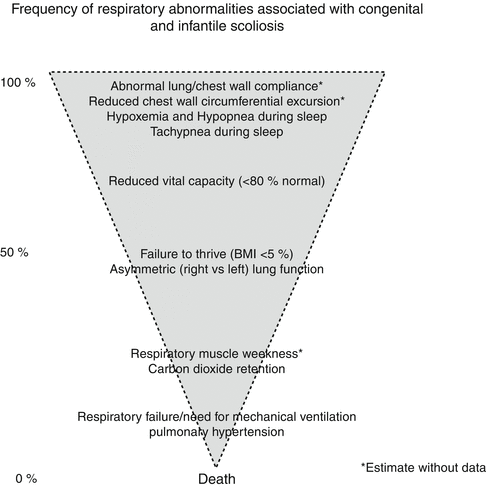
Fig. 5.1
Frequency of respiratory abnormalities associated with congenital and infantile scoliosis and other children with thoracic insufficiency syndrome
5.2 Lung and Chest Wall Mechanics
The shape and compliance of a child’s thorax change with increasing age. The depth and width of an infant’s chest are equal at birth and achieve the adult’s AP/lateral dimension ratio of 0.7 by 2–3 years of age [5]. Ribs project at right angles from vertebrae at birth and achieve degrees of angulation seen at adulthood by the third year of life. Chest wall compliance is greatest in infancy and becomes stiffer with increasing thoracic muscle development and ossification of bony structures. “Normal” stiffness of the chest wall is therefore age dependent. In human newborns, the chest wall is seven times more compliant than the lung and active chest wall and diaphragmatic muscle tone is required to maintain resting lung volumes [6]. In children up to 5 years of age, the chest wall is almost twice as compliant as the lung during quiet breathing [7]. Between 6 and 15 years of age, the chest wall normally falls by approximately 30 % [8]. In adults, the ratio of chest wall to lung compliance is close to 1.0.
In children with scoliosis, chest wall compliance is reduced, as is rib cage excursion. In response to progressively stiff chest walls, children choose to breathe shallowly to reduce the work needed to expand the chest wall with each breath. Consequently, they breathe rapidly to maintain normal minute ventilation. Respiratory rate during wakefulness is higher due to activity, excitement, and speech and is more reflective of respiratory mechanics when measured during sleep. The normal respiratory rates of children, which are age dependent, are portrayed in Fig. 5.2. Tachypnea due to scoliosis is common, but the exact prevalences of tachypnea during wakefulness and sleep have not been reported.
Lung volumes increase as the thorax increases in size. Intra-thoracic volume (at maximum inspiration) at birth increases 33-fold to reach the adult size [9]. Processes that slow thoracic cage growth therefore reduce lung volume. Respiratory compliance or distensibility is composed of lung and chest wall components. Lung compliance, apart from chest wall compliance, is volume dependent. At very low lung volumes, the lung resists expansion more when intra-thoracic pressure changes. At normal lung volumes during tidal breathing, the lung is maximally distensible. Lung volumes and lung compliance have been measured in one series of young children with early-onset scoliosis under anesthesia and found to be low [10]. Surgical procedures that increase thoracic volume, such as growth friendly constructs, also increase resting lung volume [11]. However, these same procedures also reduce chest wall compliance with insertion and serial expansion of metal into the spine or chest. [12]
With prolonged constraint of the lungs by a small thorax, alveolar development can be hampered postnatally [13]. Lung growth after birth primarily occurs in the distal or acinar regions of the lung and with increased alveolar number, size, structural complexity, and alveolar-capillary surface area. These attributes of alveolar growth change at different times, so that alveolar number and complexity increase early in life while increased alveoli enlarge later in proportion to height. [14] Lung histology from adult rabbits with scoliosis who underwent unilateral rib fusion at 7 weeks of age shows simplified larger but fewer alveoli, illustrating how small thoracic size due to EOS produces postnatal pulmonary hypoplasia [15].
Scoliosis impacts respiratory mechanics in several ways and does so more when it begins in early life than with onset during adolescence [16]. Abnormal lung volumes have been measured using infant lung function techniques as early as 6 months of age in infants with chest wall disorders [17]. Lung volumes are subdivided as illustrated in Fig. 5.3; combinations of lung volumes are described as lung “capacities.” Vital capacity is that portion of total lung capacity that can be voluntarily inhaled or exhaled with maximal efforts and reflects ventilatory reserve that could be used if needed. In contrast, residual volume is that volume of gas in the chest that remains after maximal exhalation. Residual volume provides a gas reservoir to sustain transfer of oxygen and carbon dioxide from air to blood compartments.
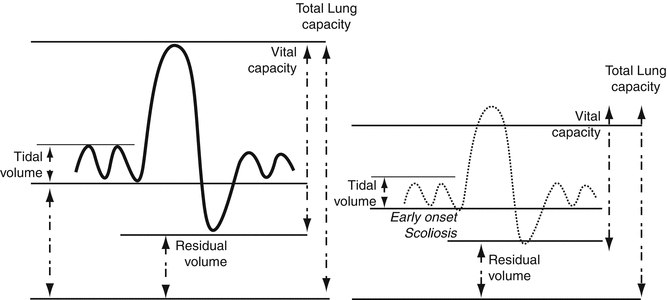
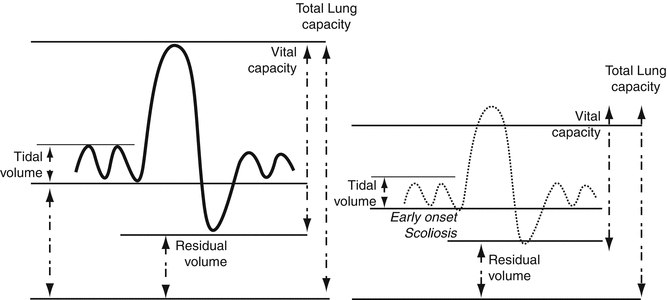
Fig. 5.3
Lung volumes and capacities in normal children and children with early-onset scoliosis
In children and adults with scoliosis, vital capacity is preferentially reduced as a result of reduced lung volumes, reduced lung and chest wall compliance, and reduced respiratory muscle function. This is illustrated in Fig. 5.3 compared to normal values. Residual volume is reduced less than the reduction in vital capacity, as it depends on thoracic cage volume and stiffness and less on respiratory muscle function. Total lung capacity, the combination of vital capacity and residual volume, is reduced less than the vital capacity because residual volume is least compromised [18]. Vital capacity is a more sensitive respiratory test to follow patients with TIS serially, as it reflects multiple changes in respiratory function. Studies in children with TIS suggest that there is greater reduction in vital capacity per degree Cobb angle if scoliosis begins earlier in life than when it begins in adolescence [16].
Vital capacity is measured by spirometry in children old enough to understand and perform maximal inspiratory and expiratory efforts. Reproducible artifact-free efforts that reflect lung function rather than how a child performs the test occur between 4 and 6 years of age. The major source of variability in spirometric results is the experience or lack thereof of the child performing the tests [19]. With practice, variability in test performance declines and coefficient of variation for vital capacity results can be as low as 5–8 % in well-practiced normal children [20]. All spirometric indices, including vital capacity, are compared to published norms that are created using height, ethnicity, and gender to normalize across different ages. In children with EOS, height reflects spine curvature as well as growth and is inappropriate to apply to reference values of normal children. Surrogates for expected height, such as arm span and ulnar length, are used instead to estimate expected height from which percent of predicted values can be derived [21–23]. In contrast to spirometry, measures of total lung capacity and all of its volume components require either gas dilution techniques or measurements with a body plethysmograph. These two techniques are available for children in tertiary care centers with a pediatric focus but are much less available than routine spirometry. Vital capacity is therefore the most clinically available spirometric test for clinicians managing children with TIS.
Spirometry also measures inspiratory and expiratory airflow. Diseases that narrow conducting airways produce obstructive lung disease. The degree of airway obstruction is usually quantified using the forced expiratory volume at 0.5 and 1 s (FEV 0.5 or FEV1) after the onset of a forced expiratory effort and the forced expiratory flow in the mid-half of the exhaled vital capacity (FEF, 25–75 % VC). FEV1 is usually normalized for vital capacity, and the normal ratio of FEV1/FVC is 80–85 %. In 4- and 5-year-olds who can perform spirometry, this ratio is 90 % or more. Importantly, FEV1/FVC is independent of age or height. Early-onset scoliosis that results in TIS produces obstructive lung disease in up to 30 % of children [24]. However, asthma is also a common condition among children, and if spirometric indices of airway obstruction are abnormal, then asthma should be ruled out using a bronchodilator challenge. Obstructive lung disease related to EOS is usually due to compression of one or both mainstem bronchi by vertebrae and mediastinal structures [24].
Pulmonary function tests in children can be subdivided by those that do and do not require cooperation and those that are more invasive in nature. Spirometric measures are non-invasive but require cooperation and maximal effort. Measures of maximal respiratory muscle strength are also effort dependent. Lung and chest wall compliance measurements do not require cooperation but do require placement of a pressure transducer within the esophagus to estimate pleural pressure. The invasive nature of esophageal balloons has led to measurements of lung compliance in the operating room under anesthesia but not during outpatient visits with children who were awake [10]. Passive inflation and deflation performed in sedated or anesthetized children do not account for the respiratory muscle dysfunction that occurs in TIS. Consequently, results obtained in supine children with EOS in the OR cannot be compared to results obtained in the same awake upright children in the clinic. The issue of cooperation in awake patients is particularly important as it limits the use of many tests in very young children with TIS who are being considered for surgical therapies. Table 5.2 lists commonly used tests of respiratory function based on the need for cooperation and for invasive procedures.
Table 5.2
Invasive and pulmonary function tests
Pulmonary function testing in infants and children | ||
---|---|---|
Invasive | Non-invasive | |
Effort—Dependenta | Trans-diaphragm pressures | Spirometry Respiratory muscle strength and fatigability Exercise tests |
Effort—Independenta | Chest wall and lung compliance | Respiratory rate Oximetry Lung volumes Blood gas tensions Lung ventilation/perfusion scans Sleep study Echocardiogram |
5.3 Gas Exchange
Respiratory gas exchange is measured by efficiency of oxygenation and sufficiency of ventilation. Oxygenation is usually measured as percent of hemoglobin saturated with oxygen (SpO2) using non-invasive pulse oximeters. These devices have replaced direct measures of partial pressure of oxygen in arterial blood except in intensive care units. Oxygenation varies with wakefulness and sleep, and SpO2 may fall by 2 % in normal children and adults during sleep [25]. In children with TIS, SaO2 is normal (>96 %) in most children when awake. In the initial study of 218 children with TIS who underwent expansion thoracoplasty, only 22 % received supplemental oxygen therapy [26]. However, 10 of 11 children with TIS whose breathing was studied during sleep experienced recurrent but brief episodes of hypoxemia, particularly during rapid eye movement (REM) sleep [27]. Additionally, elevated hemoglobin levels were reported in 23 % of children with EOS, suggesting recurrent hypoxemia during sleep with increased erythropoietin levels and mild polycythemia [28]. Non-invasive positive pressure ventilation such as bi-level positive airway pressure (BIPAP) effectively improves hypoxemia during sleep in children with EOS [29].
Ventilation is assessed using arterial or arterialized measurement of carbon dioxide partial pressures in blood. Capillary sampling of arterialized blood is commonly used to measure PCO2 in the outpatient setting. Retention of carbon dioxide above normal levels, i.e., PCO2 > 50 mmHg, is uncommon and a late finding in children with TIS, reflecting acute and/or chronic respiratory failure. An alternative way to measure CO2 status is with a capnograph which measures CO2 concentration in exhaled gas. The end-tidal PCO2 can be used as a non-invasive surrogate measure of arterial PCO2 in children who have no underlying lung disease and only spine and chest wall disease. End-tidal PCO2 has been used during sleep studies to identify frequency, duration, and severity of hypercapnia. Subacute and chronic hypercapnia can be assessed by the total CO2 content in the blood, a measure of compensatory metabolic alkalosis in response to sustained respiratory acidosis. The utility of this measure is that it is less susceptible to sampling errors than capillary or arterial CO2 tensions. It may also be elevated after transient hypercapnia during sleep when assessed early in the morning.
5.4 Lung Function Asymmetry
One consequence of progressive chest wall deformity due to EOS is an asymmetric chest wall shape and increasingly different volumes in the right and left hemi-thoraces. Different capabilities to move the right and left chest walls due to differences in rib alignment, hemi-diaphragm configuration, and regional chest wall compliances lead to asymmetric lung volumes and also to asymmetric ventilation and perfusion between the right and left lungs. Regional lung ventilation and perfusion are measured with lung scans. Ventilation is measured using inhalation of a radiotracer gas; regional lung perfusion is measured using intravenous radiolabeled agents. Lung perfusion scans require minimal cooperation and can be used in children of all ages. In infants, the normal distribution of ventilation and perfusion to the right and left lungs is 50:50 %. As the chest wall becomes more oblong transversely, there is a shift to the adult distribution of 55 % right lung:45 % left lung for both ventilation and perfusion [30]. Perfusion is normally well matched to ventilation.
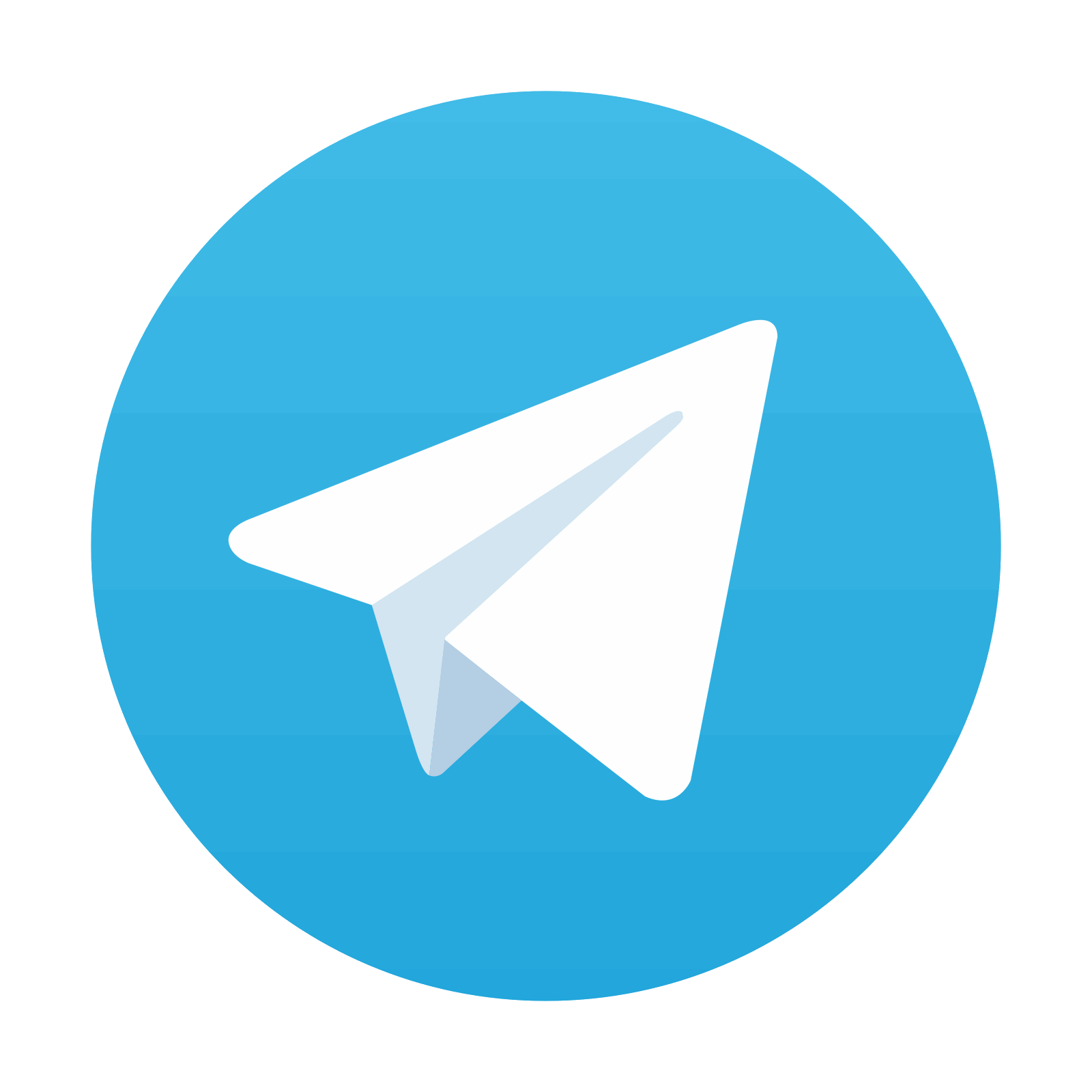
Stay updated, free articles. Join our Telegram channel

Full access? Get Clinical Tree
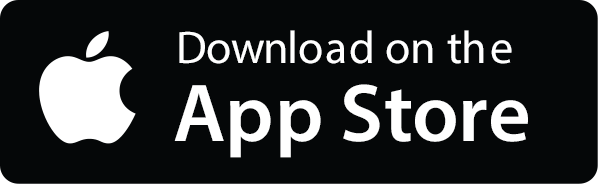
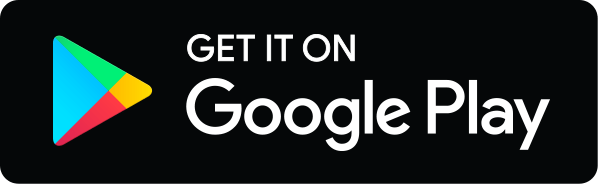