Abstract
Fatigue and attention disorders are common in modern society and can cause significant difficulties in the lives of those afflicted. Increases in cognitive fatigue are typically characterized by a decline in attention, difficulties concentrating, and increased reaction times (Caldwell, 2005). In fact, cognitive impairments caused by just moderate levels of fatigue have been shown to exceed those caused by alcohol intoxication at a blood–alcohol content (BAC) of 0.08 (Dawson and Reid, 1997). While the cause of the fatigue or attentional symptoms vary, there is a growing body of evidence that suggests a benign form of noninvasive brain stimulation known as transcranial direct current stimulation (tDCS) can augment brain activity in a manner that provides therapeutic relief of major symptoms, improves mood, and reduces associated cognitive performance declines.
Keywords
Central fatigue (CF), Central fatigue syndrome (CFS), DLPFC, Mackworth Clock Test, Transcranial direct current stimulation (tDCS)
Outline
Introduction 1637
Medical Causes of Fatigue and Chronic Fatigue Syndrome 1637
Nonmedical Sources of Fatigue 1638
Transcranial Direct Current Stimulation as a Countermeasure for Fatigue 1640
Attention and Vigilance 1643
Transcranial Direct Current Stimulation’s Effects on Attention and Vigilance 1644
References 1645
Introduction
Fatigue and attention disorders are common in modern society and can cause significant difficulties in the lives of those afflicted. Increases in cognitive fatigue are typically characterized by a decline in attention, difficulties concentrating, and increased reaction times ( ). In fact, cognitive impairments caused by just moderate levels of fatigue have been shown to exceed those caused by alcohol intoxication at a blood–alcohol content (BAC) of 0.08 ( ). While the cause of the fatigue or attentional symptoms vary, there is a growing body of evidence that suggests a benign form of noninvasive brain stimulation known as transcranial direct current stimulation (tDCS) can augment brain activity in a manner that provides therapeutic relief of major symptoms, improves mood, and reduces associated cognitive performance declines.
Medical Causes of Fatigue and Chronic Fatigue Syndrome
Fatigue is a common symptom in a variety of neurological disorders such as major depressive disorder (MDD), cancer, anxiety, multiple sclerosis (MS), mitochondrial diseases, stroke, traumatic brain injury (TBI), chronic pain, and chronic fatigue syndrome (CFS). Patients often describe fatigue as quickly reaching exhaustion while engaged in activity, attention and vigilance declines, reduced energy levels, feeling sleepy or tired even at rest, high inertia, low endurance, and low vigor ( ). Sometimes fatigue symptoms can manifest with no apparent underlying cause. When such symptoms persist for more than six months, it is referred to as “chronic fatigue syndrome” ( ).
divide fatigue into two types: central fatigue (CF) and peripheral fatigue. As the name implies, CF is caused by abnormal function or physiology in the central nervous system (CNS). Conversely, peripheral fatigue arises from bioenergetics, excitation-contraction uncoupling, or peripheral nerve changes ( ). For the purposes of this chapter, the focus will be on CF.
CF can be caused by a variety of medical disorders. Perhaps one of the more debilitating is Myalgic encephalomyelitis/chronic fatigue syndrome (ME/CFS). This is characterized by persistent incapacitating fatigue that lasts for more than 6 months. This level of fatigue impairs normal activities, is accompanied by nonspecific somatic symptoms, and has no identifiable medical or psychological problems to account for it. In addition to fatigue, people may complain of loss of memory or concentration, headaches, unrefreshing sleep, depression, enlarged lymph nodes, unexplained muscle or joint pain, sore throat, and hypotension. ME/CFS is classified as a disease of the CNS but is only diagnosed when other conditions have been ruled out. There is no diagnostic test and the symptoms vary in presence and severity from patient to patient. ME/CFS is poorly understood as it seems to be a combination of the malfunctioning of the short-term (autoimmune and/or autonomic nervous system) and long-term (hypothalamus–pituitary–adrenal (HPA) axis, psychological) stress response systems ( ).
There is evidence for both altered immune functioning and for perpetuating psychological factors in the development or maintenance of CFS that may be mutually reinforcing ( ). The onset of CFS is often sudden and precipitated by an infectious episode ( ). Subsequent infections may play a large part in worsening of fatigue symptoms ( ), signaling an autoimmune response. There is also a growing body of evidence that the immune system of patients with CFS might respond differently to exercise when compared to healthy, sedentary controls, potentially triggering an increase in symptoms ( ). The HPA axis is a psychoneuroendocrine regulator of the stress response and immune system, and dysfunctions have been associated with outcomes in several physical health conditions ( ). Dysfunction of the HPA axis in ME/CFS patients may manifest as hypocortisolism, which is relevant to fatigue and due to cortisol’s role in energy metabolism ( ) and response to stressors ( ).
The presence of infectious agents, immune dysfunctions, psychological influences on the illness, and the dysfunctional HPA axis may be the outcome of hypersensitivity of the CNS (i.e., central sensitization). The increased sensitivity to a variety of stimuli (including mechanical pressure, chemical substances, light, sound, cold, heat, and electricity) results in a decreased load tolerance ( ), as the nervous system gets regulated in a persistent state of high reactivity. This persistent hypersensitivity may maintain dysfunction, even after its trigger has been alleviated.
ME/CFS shares abnormalities along with other CNS disorders, including fibromyalgia and MS. All three disorders are associated with widespread pain, fatigue, sleep disturbance, depression, sensory and motor impairments, and reduced quality of life (QOL) ( ) due to a lack of optimal options for symptom management. In a neuroimaging study comparing MS and ME/CFS, the neuroimages are quite similar in both illnesses and show decreased cerebral blood flow, atrophy, gray matter reduction, white matter hyperintensities, increased cerebral lactate and choline signaling and lowered acetyl-aspartate levels ( ).
The pathophysiology of fatigue in MS remains unclear but it has been suggested that functional (reduction of glucose metabolism, communication disruption) and structural (lesions, atrophy) alterations in the frontal cortex play a role in fatigue severity ( ). MS-related fatigue has also been attributed to atrophy within the parietal lobe ( ). Because fatigue may derive from frontal or parietal area hypoactivation, tDCS may benefit patients by restoring activation in these areas ( ).
Nonmedical Sources of Fatigue
Chronic fatigue can also manifest from nonmedical sources such as lifestyle, schedule, use of stimulants, and sleep hygiene. For example, fatigue is a ubiquitous part of many professions that are not conducive to good sleep hygiene (e.g., healthcare, trucking, manufacturing, defense, and aviation, etc.). It is particularly prevalent during night shifts where workers exhibit the lowest levels of performance and alertness ( ). Unfortunately, over 22 million Americans work shifts that are outside of the normal day shift, according to the . Due to “24/7” operations, such shifts are particularly prevalent in the military. In fact, 96% of US Army aviation units surveyed indicate that they worked a night shift in their career ( ). The intelligence, surveillance, and reconnaissance missions have become exceedingly popular with military commanders, which has led to an extremely high operations tempo. As a result, unmanned aircraft carrying out these missions often operate 24 h a day.
Physiological factors such as the body’s natural circadian rhythms play a large role as the body provides natural signals to ready the individual for sleep at night. Arousal mechanisms increase during the daytime morning hours when night shift workers often attempt to go to sleep. Attempting to overcome these physiological mechanisms is extremely difficult and previous research suggests at least a week is needed for workers to change their internal circadian rhythms from day to night ( ). Even then, most workers never completely adjust to night work ( ). Not surprisingly, their day sleep is approximately 1–2 h shorter than their normal sleep periods that occur at night ( ). However, shift work fatigue effects are not due to circadian factors alone. Many night shift workers spend a longer time awake before their shift ends (20–22 h) when compared to other shifts, which can contribute to increased fatigue during their shift ( ). Because of the shorter sleep periods during the day, there is an accumulation of “sleep debt” over time. Research has shown that increasing consecutive nights of work duties leads to more accidents and less productivity, purportedly due to the accumulated sleep debt and resulting CF ( ). To counter the daytime sleep deficiencies, countermeasures such as earplugs to mask noise, eyeshades to reduce environmental light, and hypnotics to induce the sleep cycle (e.g., zopiclone (Lusesta) have been proposed ( ). Nevertheless, daytime sleep remains lest restful and shorter than necessary to fully recuperate.
Reduced/restricted sleep or complete sleep deprivation is obviously a high contributor to CF and is most prevalent in high stress professions such as medicine, emergency response, aviation, and the military. There is a large body of literature showing a link between CF and cognitive performance decrements such as slower response time, reduced response accuracy, multitasking decrements, attention lapses, planning degradations, and decision-making errors (e.g., ). Generally, fatigue is believed to cause a general slowing of cognitive processes ( ). For example, nurses who reported an error or near error slept significantly less than those that did not make errors ( ). showed nurses with long shifts produced significantly more procedural and medication errors. Commercial pilots consistently list sleep deprivation, sleepiness, and fatigue as contributing factors in mishaps, fuel miscalculations, landing errors, course deviations, and other mistakes ( ). Helicopter pilots and crews within the US Army also blame fatigue caused by sleep loss for errors committed in the cockpit and lapses of vigilance/attention ( ). The situation is nearly identical for US Air Force pilots, largely due to long-haul missions and the high operations tempo ( ). provided evidence that long-haul pilots suffer from vigilance decrements, especially when workload is low. Such attentional lapses leads to low-situation awareness, thereby increasing the risk of a mishap or mission failure. In fact, the high operations tempo has been shown to produce cognitive deficits equivalent to those induced by blood alcohol concentrations in the range of 0.05%–0.08% ( ). Not surprisingly, both military and commercial pilots consider sleep a serious problem within their profession ( ).
In addition to behavior, CF also negatively impacts mood. Commonly, sleep deprivation leads to reductions in vigor, energy, attention, optimism, and ability to complete cognitive tasks, coupled with increases in sleepiness, confusion, hostility, and distress ( ). Physiologically, a bi-product of cortical metabolism, known as adenosine, builds up during periods of wakefulness ( ). Adenosine then binds to its receptors (A1, A2) and inhibits brain activity through blockage of excitatory neurotransmitters including dopamine, glutamate, and acetylcholine (ACh) ( ). The resulting decline in cortical excitability leads to an increased feeling of sleepiness and negatively impacts the brain’s ability to carry out many cognitive functions. For example, ACh is a major excitatory catecholamine believed to facilitate wakefulness and attention ( ). Further, ACh levels have been associated with visual working memory, which is important in visual search, planning and decision-making ( ). Dopamine is another catecholamine that impacts working memory and sustained attention (i.e., vigilance) through regulation of activity in the prefrontal cortex ( ). Hence, reduced ACh release contributes to many of the cognitive deficits listed previously. In fact, one of the mechanisms by which caffeine is known to exert its effects is through blocking adenosine A2 receptors ( ). This prohibits adenosine from blocking the release of dopamine thereby reducing the cortical excitability inhibition effects. Likewise, caffeine stimulates the noradrenergic centers (i.e., locus coeruleus) causing increased release of norepinephrine (NE). This increases wakefulness, attention, and arousal, while simultaneously increasing blood pressure, heart rate, and blood flow ( ). Not surprisingly, caffeine has become a ubiquitous and readily available substance in modern society for combating fatigue. It is even included in many military rations ( ). However, there are several limiting factors with caffeine consumption.
While temporarily effective at reducing cognitive effects caused by CF (e.g., ), caffeine’s effects are short-lived ( ), the effects are diminished when fatigue levels are high ( ), and its effectiveness declines with chronic use ( ). While it functions well in increasing wakefulness, it does not appear to improve decision-making and response accuracy ( ) and the effects on mood are mixed. Some researchers have found negative consequences of caffeine intake such as increased depression and confusion ( ). Others have found positive effects on mood with similar doses ( ). Furthermore, high doses (i.e., above 400 mg) can cause a condition known as “caffeine intoxication” that produces declines in mood, insomnia, nervousness, hallucinations, and intestinal complaints ( ). Because of caffeine’s side effects and limitations, researchers have begun examining more direct neuromodulation techniques to reduce fatigue through noninvasive brain stimulation.
Transcranial Direct Current Stimulation as a Countermeasure for Fatigue
A noninvasive brain stimulation technique known as transcranial direct current stimulation (tDCS) has emerged as a potential treatment for reducing fatigue symptoms. The technology passes a weak electrical current (typically <4 mA) though electrodes (anode and cathode) placed on the scalp/body. Fig. 139.1 provides an image of the set-up. The current has been shown to modify neural excitability in a polarity dependent manner (i.e., increased excitability under the anode and decreased excitability under the cathode) when applied to the motor cortex, and this excitability change augments the resulting behavior.

When examining cognitive effects, the two polarities often do not cause opposite results. In a recent meta-analysis, it was noted that both polarities cause similar behavioral outcomes in cognitive tests ( ). In other cases, cathodal tDCS may produce no effect at all (when compared to sham). The authors theorized that this phenomena may be a result of the fact that cognitive processes use a complex network of brain regions and that other network nodes may help compensate for the areas exhibiting reduced excitability. Regardless, there have been recent developments providing evidence that tDCS applied to the left prefrontal cortex or primary motor cortex (DLPFC) can assuage CF symptoms.
In the clinical realm, studies have shown that MS and fibromyalgia patients tolerated tDCS well without adverse events ( ). found that tDCS over the primary motor cortex (M1) reduces fatigue ratings in 65% of MS patients by 30% and those benefits last at least 3 weeks poststimulation. Likewise, found that anodal tDCS over the right parietal cortex may counteract the increase in reaction time that is associated with the vigilance decrement in MS patients by enhancing processing in the parietal cortex. There is also converging evidence that tDCS over left DLPFC has stimulant-like effects. In a study by , MS patients with higher lesion load (numbers of lesions on MRI) responded positively to anodal stimulation over the left prefrontal cortex. However, when grouped with patients with lower lesion load, they found that fatigue ratings were not altered by tDCS ( ). Because fatigue has been associated with higher lesion load, the low lesion load patients may not have benefited simply because their fatigue levels were already low. investigated sleep efficiency and arousal levels for patients with fibromyalgia. They found that stimulation over M1 increased sleep efficiency and decreased arousal while stimulation of the DLPFC decreased sleep efficiency and increased arousal. Because of these trade-offs, it is unclear whether DLPFC or M1 is the ideal cortical target for fatigue reduction. In another study, fibromyalgia patients under the active tDCS condition reported a small but significant improvement in pain and improved daily functioning ( ). Nevertheless, these initial studies have provided evidence that anodal tDCS over left DLPFC or left primary motor cortex may reduce subjective ratings of fatigue, particularly in patients suffering from high lesion load MS and fibromyalgia. It is possible that tDCS may counteract abnormal hypoactivation associated with MS in the motor and prefrontal regions and restore activity to more normal levels. Because tDCS also have been shown to elicit long-lasting effects that are likely a result of long-term potentiation (LTP) ( ), tDCS may also help to mitigate communication dysfunction of the frontal and motor areas by strengthening existing connections or helping form new synaptic connections in the remaining healthy tissue. In doing so, it would restore the normal volitional drive of the descending motor pathways and reduce added cognitive or physical effort caused by abnormal communication and activity.
Due to the successes in treating fibromyalgia and MS with tDCS, it is possible that ME/CFS may also benefit from similar treatments. TDCS has potential as both a clinical application for symptomatic management and as a treatment strategy targeting the HPA axis and hypersensitivity of the CNS. It is simple, inexpensive, noninvasive, and can induce sustained excitability changes in relatively restricted human brain areas.
Efforts have begun to examine the effects of anodal tDCS applied to the DLPFC on hypersomnia. In an initial case study, found that tDCS applied bilaterally to the DLPFC improved both objective (evaluated via the psychomotor vigilance test (PVT)) and subjective vigilance, while simultaneously reducing sleepiness scores in a patient with organic hypersomnia. Following two blocks of tDCS applied over three consecutive days, the authors reported that the patient exhibited less daytime sleep and attained an overall improvement in QOL. Although this was not a controlled study, it provided important initial evidence that tDCS may have therapeutic effects for hypersomnia patients for which there are current few treatment options. examined the effects of tDCS on hypersomnia over 4 weeks of treatment (three treatments per week). Similar to Frase’s single case study, the results showed that patients who received the tDCS treatment had significantly less subjective daytime sleepiness and faster response times in an attention test when compared to the sham condition. Hence, the authors concluded that tDCS may be a treatment option for managing hypersomnia symptoms. Frase has also discovered that the anodal, bifrontal tDCS paradigm utilized in the hypersomnia patient reduces total sleep time in healthy individuals ( ). The authors posit that stimulation of the prefrontal cortex induces a “top-down” regulation of sleep-wake regulation via the corticothalamic pathway. While cathodal, bifrontal tDCS did not increase total sleep time, they did find that anodal tDCS-induced increased cortical arousal while cathodal tDCS-induced decreased cortical arousal, as evidenced by resting-state EEG recordings. Further, recently examined the efficacy of repeated tDCS on fatigue symptoms in patients suffering from Parkinson’s disease (PD). Again, the authors found that anodal tDCS applied to the DLPFC reduced fatigue symptoms in these patients but were unable to shown any effects on daytime sleepiness. In another study, researchers applied tDCS to the left DLPFC of TBI patients ( ). They discovered that anodal tDCS improved attention scores immediately following stimulation, but the effect was not present 3 h after stimulation.
Investigations of tDCS as a fatigue mitigation tool have expanded to healthy, normal populations. In a seminal work, found that tDCS can remediate the deleterious cognitive and mood deficits caused by sleep deprivation for at least 6 h poststimulation in healthy normal participants. Specifically, the authors found that tDCS improved vigilance performance, improved arousal, and reduced declines in mood when compared to participants that received either sham tDCS or caffeine. Participants reported feeling less fatigued, less drowsy, more energetic, and their composite mood scores were elevated over their sham and caffeine counterparts. The authors concluded that tDCS was twice as effective as caffeine and the effects persisted three times as long ( ). One interesting finding was that tDCS tended to preserve performance and mood rather and enhance them over baseline levels. This is consistent with previous work in the attention domain (i.e., ). Of note, the targeted brain region (i.e., left DLPFC) was the same brain target that showed promise for reducing fatigue in MS and PD patients ( ). Another study shows that the effects of tDCS are even longer lasting than originally reported ( ). When applied earlier in the sleep deprivation period, the effects on attention were analogous to those described by . However, the effects on mood and arousal were more pronounced and these effects persisted at least 24 h. Reaction times were significantly faster in the tDCS participants when compared to either the sham or caffeine groups, starting 12 h post-tDCS and lasting 24 h post-tDCS. In addition, working memory performance was significantly higher in the tDCS group. Subjectively, participants reported feeling more able to do the tasks, more focused, more optimistic, less angry, and less distressed. The authors concluded that early intervention tDCS applied to the left DLPFC stabilizes both mood and performance to offset the negative effects of fatigue encountered in sleep deprivation.
Because of the effects of tDCS on both sleep and arousal, researchers have begun to discuss whether it is impacting “top-down” or “bottom-up” control. The top-down pathway suggests that regulation of arousal and sleep stems from brain activity originating in the frontal cortex that is then communicated to the thalamus ( ). Information from the thalamus is then fed back to the frontal cortex in a constant feedback loop known as thalamocortical oscillations ( ). According to , these oscillations form the “pacemaker” for brain oscillations during non-REM sleep. Because tDCS applied to frontal regions of the cortex have been shown to modulate arousal and impact sleep, it is possible that the stimulation is producing such effects through the top-down pathway. In fact, functional magnetic resonance imaging (fMRI) data reveals that tDCS does in fact change functional connectivity in thalamocortical circuits ( ). Fig. 139.2 provides functional magnetic resonance imaging data from tDCS applied over the left DLPFC. The images display the difference between the sham and active tDCS groups. The areas in red indicate higher activity in the active tDCS group when compared to sham. Both cortical and subcortical regions exhibit greater excitation with active tDCS. Given the excitatory neuromodulatory effects of anodal tDCS, the current likely induces frontal activity that induces a more wakeful, attentive state. This is then communicated downstream to subcortical areas via the thalamus.

Others have postulated that tDCS may modulate arousal and sleep through a “bottom-up” mechanism. Because of the large distance between the anode and cathode described by (i.e., one on the scalp and the other on the shoulder), it is possible that the current followed a deeper pathway through the brain, exciting subcortical regions along the way. In particular, it is possible that the LC was excited due to its involvement in regulating attention and maintaining vigilance and wakefulness ( ). LC interactions with cortical and subcortical (e.g., thalamus) sensory and attention networks modulate activity states that are conducive to collecting salient, sensory information ( ). Known as “waking,” this increase in arousal comes with an increased ability to detect critical or salient information in the environment, process that information, and make the appropriate response ( ). Noradrenergic activity from the LC contributes to increased forebrain activation inducing the waking state and enhanced arousal. Further, NE released by the LC modulates long-term changes in synaptic strength (plasticity) indicating a critical role in memory formation. The effects of LC activation on arousal, vigilance, attention, and plasticity allow humans to adapt to the changing environment and augment what information is important to learn in order to assess threats and survive. Given that there appears to be a robust effect of tDCS on arousal, attention (vigilance), and subjective wakefulness, the evidence suggests LC is modulated by the prefrontal cortex tDCS paradigm ( ). Hence, the observed effects of tDCS on arousal, vigilance, and wakefulness are likely related, in part, to lasting changes in synaptic plasticity in the LC and basal forebrain that may result in stronger and/or prolonged increase release of excitatory neurotransmitters such as ACh and noradrenaline.
Another different possible pathway that induces a bottom-up regulation of arousal and sleep has been proposed by . The authors state that because the anode is located proximal to projections of the trigeminal nerve when targeting the DLPFC, the current may be traveling down that nerve and modulating activity in the LC. Interestingly enough, the trigeminal nerve is merely one synapse away from the LC and demonstrated that exciting this nerve does significantly influence LC activity. When evaluating changes in mood via the Profile of Mood States (POMS) questionnaire, both stimulation of the trigeminal nerve ( ) and tDCS of the DLPFC ( ) produced significant improvements in mood. suggest that the tDCS’s effects may be, in part, due to peripheral effects such as trigeminal nerve stimulation.
Regardless of the mechanism, tDCS is very promising in reducing fatigue symptoms in a variety of neurological disorders. In particular, there is new evidence that tDCS may provide therapeutic benefits to those suffering from MS, PD, hypersomnia, and TBI. Further, evidence suggests that it can remediate the cognitive effects of fatigue and help the users feel less drowsy and more energized. While these data are promising, more research is needed to assess the utility of tDCS as a fatigue countermeasure outside of the laboratory environment. Due to the long-lasting nature of the stimulant-like effects, it is possible that tDCS could negatively impact sleep quality. This is further supported by the evidence that tDCS can reduce daytime sleep in hypersomnia patients. Such possible side effects should be further evaluated.
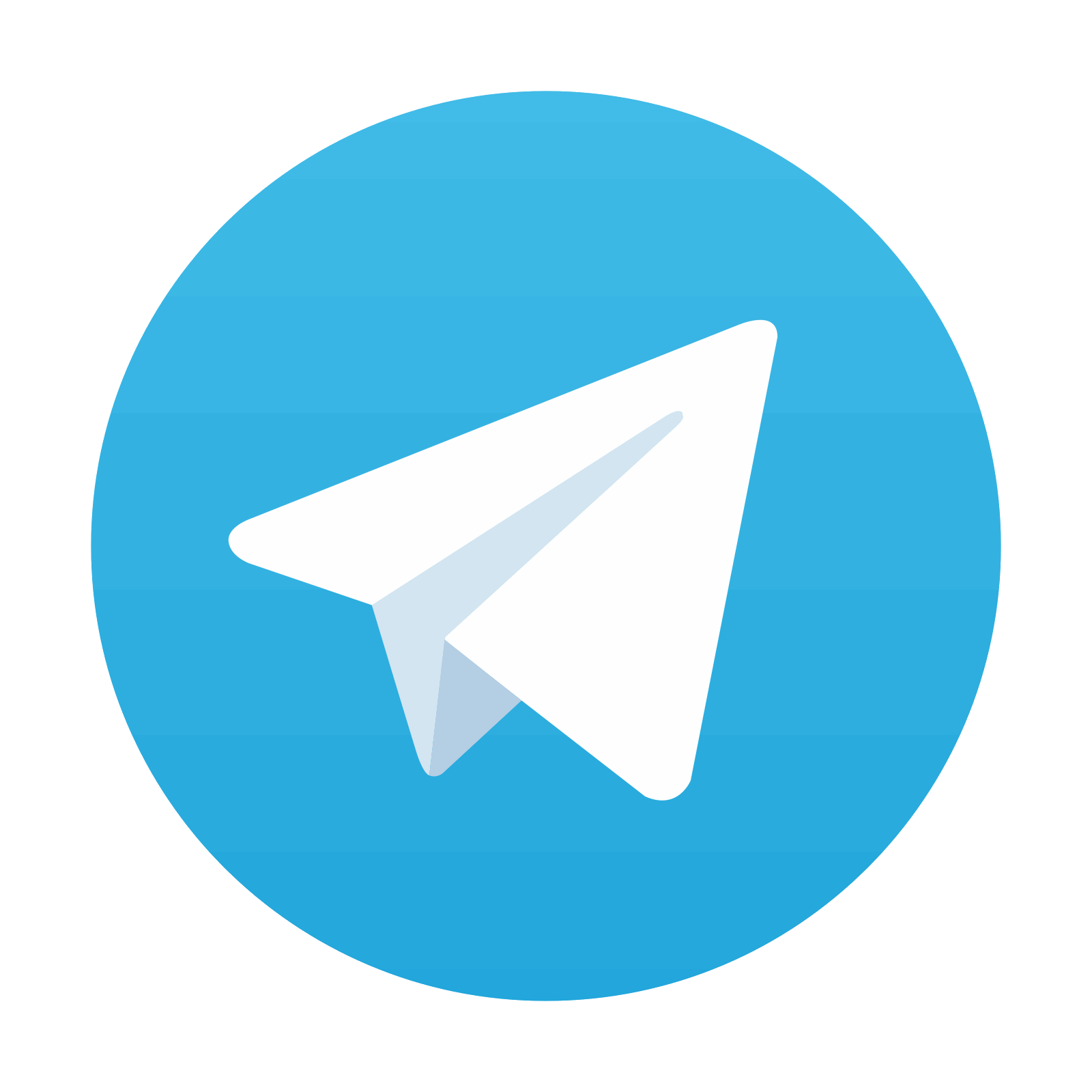
Stay updated, free articles. Join our Telegram channel

Full access? Get Clinical Tree
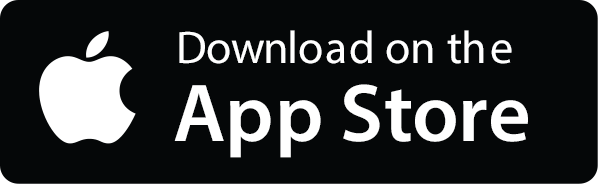
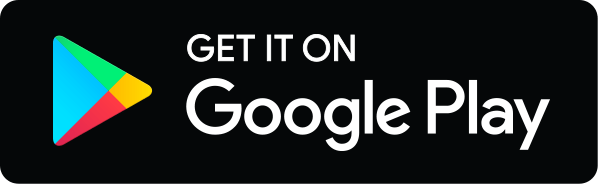