Abstract
Traumatic brain injury (TBI) is a major health problem worldwide. Following the primary injury, there is no cure to rescue the damaged neural tissue. Current treatment options for TBI mostly focus on targeting the secondary injuries. The secondary injuries are a series biochemical processes that occur in response to the primary mechanical insult which often lead to further neural tissue loss in days and weeks following the primary injury. Finding a treatment that can prevent, reduce, and repair secondary damage is instrumental in the recovery of TBI patients. The flavonoid 7,8-dihydroxyflavone (7,8-DHF) is a tropomyosin-receptor-kinase B (TrkB) agonist that mimics the effects of brain-derived neurotrophic factor (BDNF). Upon binding to the TrkB receptor, signaling cascades are initiated that can promote neuronal survival and regeneration. The use of 7,8-DHF in the treatment of TBI is favorable due to its longer half-life and ability to pass the blood–brain barrier. In this review, we summarized functions of BDNF/TrkB signaling pathway and studies examining the potential of 7,8-DHF as a therapy for TBI.
Keywords
7,8-Dihydroxyflavone, BDNF, Neuronal degeneration, Neuroprotection, Traumatic brain injury, Tropomyosin-related kinase B (TrkB) receptor
Introduction
Approximately 350,000 individuals in the United States are affected annually by severe and moderate traumatic brain injuries (TBIs) that may result in long-term disability. This rate of injury has produced over 3 million disabled citizens in the United States alone. Despite generally improving rates of survival after TBI, approximately 80,000 individuals in the United States annually sustain TBIs that result in significant long-term disability. These impairments involve both sensory motor and cognitive functions and can result in a total vegetative state. Most of these 3 million TBI survivors depend upon others for daily care. Many clinical and animal model studies have now shown that severe and even moderate TBI is characterized by both neuronal and white matter loss with resultant brain atrophy and functional neurological impairment. Injury may be in the form of focal damage, as typically occurs after acute subdural hematoma, or it may be diffuse with widespread delayed neuronal loss as typically occurs after diffuse axonal injury.
TBI can be described as a loss of brain function due to an external mechanical force. The cells and tissue that are directly injured upon impact, including neurons, glia, blood vessels, and axons, are considered primary damage ( ). Following the primary injury, the subsequent secondary injury induces further tissue loss in the surrounding tissues of the initial impact site. Many biochemical processes, such as excess excitotoxin release, increased intracellular calcium concentration, lipid peroxidation, inflammatory mediators, and the production of free oxygen radicals, contribute to the secondary damage ( ). Secondary injury can occur over days to weeks after TBI ( ) and result in diffuse neurodegeneration that affects motor and cognitive function ( ). To date, there is no effective treatment for TBI. Current therapies are primarily focused on reducing the extent of secondary insult rather than repairing the damage from the primary injury.
To reduce secondary injury, strategies which have neuroprotective effect salvaging the injured brain tissue in the early stage postinjury and promote regeneration at the recovery stage are desirable. The brain-derived neurotrophic factor (BDNF) and its high affinity receptor tropomyosin receptor kinase B (TrkB) play a critical role in neuronal differentiation and survival, synapse plasticity, and memory. Targeting BDNF/TrkB signaling pathway has a remarkable therapeutic potential for many neurological disorders including TBI. This chapter will review recent understanding and progress in experimental TBI therapeutic development targeting this signaling pathway with focus on the potential of TrkB receptor agonist 7,8-dihydroxyflavone (7,8-DHF) for treating TBI ( Fig. 14.1 ).

Neurotrophins and Tropomyosin Receptor Kinase B Receptor
Neurotrophins are a group of polypeptides with great influence on neuronal differentiation, survival, axon growth, and synaptogenesis in the central nervous system (CNS) and peripheral nervous system (PNS) ( ). The neurotrophin family members include nerve growth factor (NGF), BDNF, neurotrophin-3 (NT-3), and neurotrophin-4 (NT-4). In the CNS, both neuronal and nonneuronal cells synthesize neurotrophins in a precursor form, or proneurotrophin form. The immature proteins are then either secreted or cleaved by plasmin or extracellular proteases into the mature form ( ).
The diverse effects that occur at the presence of neurotrophins are a result of the ligand-receptor binding and the specific signal cascades that follow. This includes two transmembrane-receptor signaling systems that consist of the p75 neurotrophin receptor (p75 NTR ) and the tyrosine kinase receptors: TrkA, TrkB, and TrkC. The p75 NTR belongs to the tumor necrosis factor (TNF) receptor super family while the tyrosine kinase receptors belong to the tropomyosin receptor kinase (Trk) family ( ). The Trk family of receptors is instrumental in carrying out the cellular effects of neurotrophins. Among Trk family receptors, TrkB acts as a receptor for BDNF and NT-4 ligands. NT-3 binds to TrkC and can also bind TrkB with a reduced affinity. NGF has a higher affinity for TrkA ( ). It is through high-affinity binding for TrkB that neurotrophin is able to provide neuronal survival, neuronal plasticity, and neurogenesis ( ), whereas binding with the p75 NTR receptor is more associated with apoptosis. Both TrkB and p75 NTR receptors can be found in the same cell, coordinating and modulating neuronal responses. Furthermore, the signals generated by each receptor can augment each other or go against each other, fluctuating between an enhancing and suppressing relationship ( ).
TrkB Signaling Pathways and Its Function
TrkB is a single pass type 1 membrane protein expressed in both the CNS and PNS. In the CNS, TrkB is expressed at high levels in the cerebral cortex, hippocampus, thalamus, choroid plexus, cerebellum granular layer, brain stem, retina, and the spinal cord ( ). In the PNS, TrkB is found in the cranial ganglia, vestibular system, submaxillary glands, and the dorsal root ganglia ( ).
Binding of neurotrophins and TrkB induces activation of signaling pathways that include mitogen-activated protein kinase (MAPK)/Ras pathway, phosphatidylinositol 3-kinase (PI3k)/serine threonine kinase (Akt) pathway, and/or phospholipase Cγ (PLCγ) pathway. These signal cascades play important roles in plasticity and survival of neurons as well as neurogenesis ( ).
The MAPK pathway stimulates antiapoptotic proteins, such as B-cell lymphoma-2 (Bcl-2) and cAMP-response-element binding protein (CREB). In particular, CREB is required by neurotrophins for mediating neuronal survival and memory function ( ). MAPK can also activate extracellular signal-related kinase (ERK) ( ). MAPK pathway leads to further phosphorylation of synapsin I, a protein involved in synaptic vesicle clustering and release ( ). Interfering with the MAPK pathway has shown to decrease BDNF-induced synapsin I phosphorylation; thus inhibiting the release of neurotransmitters ( ). Ras, a small guanosine 5′ triphosphate-binding protein, is often responsible for neurotrophin-dependent survival, but it does not have a direct effect on survival. Ras turns neurotrophin-initiated signals into other multiple signaling pathways, including PI3k/Akt and MEK/MAPK ( ). Overall, MAPK activation leads to neuronal survival and differentiation ( ).
TrkB activates PI3k/Akt pathway promoting neuronal survival through regulating proteins that influence cell survival and transcription factors that influence apoptosis ( ). TrkB activation also regulates synaptic plasticity by promoting the PLCγ-mediated pathways through downstream protein kinase C signaling and calcium/calmodulin kinase pathway ( ).
TrkB pathway plays an important role in neural development and maintenance through regulating neuronal differentiation, survival, neurogenesis, and plasticity. Disruption of TrkB pathway leads to abnormal Purkinje cell dendrite morphology, atypical cerebellum development, and diminutive geniculate and nodose ganglia ( ). BDNF and TrkB are upregulated in areas where there is neuronal plasticity occurring. In the hippocampus, BDNF/TrkB signaling can affect the maturation of dendritic trees and synaptic terminals. BDNF/TrkB signaling in the hippocampus modulates synaptogenesis of the mossy fibers extending from the dentate granule cell layer to the hilus and the CA3 region ( ) and has a positive effect on learning and memory by regulating both short-term synaptic function and hippocampal long-term potentiation ( ). Animal studies have shown that absence of BDNF or TrkB has a great impact on the formation of long-term potentiation in hippocampal synapses, leading to poorer results in certain learning tasks ( ). Disruption of the pathway can result in the clinical symptoms of deteriorating memory and cognitive dysfunction ( ). Many studies have also shown a relationship between lower levels of BDNF and cognitive decline in aging, Alzheimer’s disease, schizophrenia, and Rett’s syndrome ( ). BDNF/TrkB signaling activation also mediates neuronal survival particularly the newly generated neurons in the neurogenic regions in the adult brain ( ).
The Molecule 7,8-Dihydroxyflavone
The importance of BDNF/TrkB signaling pathway in regulation CNS function has inspired much research exploring the therapeutic potential of BDNF for varying neurological diseases including TBI with exciting outcomes in animal studies over the past decade. Although BDNF plays crucial role in neuronal survival, differentiation, and synaptic plasticity, the therapeutic potential of BDNF is restricted due to its limited ability to diffuse in the CNS parenchyma, short half-life, and inability of permeating the blood–brain barrier (BBB) ( ). Thus small compounds such as TrkB agonists that mimic BDNF’s neurotrophic signaling without its pharmacokinetic barriers may have greater therapeutic potential.
Flavonoids are groups of compounds which have the potential to provide neuronal protection, enhance existing neuronal function, and stimulate neurogenesis ( ). The screening of chemical libraries has resulted in the discovery of a flavone derivative, 7,8-dihydroxyflavone (7,8-DHF), a polyphenolic compound family found in fruits and vegetables which mimics BDNF functions due to its ability to bind to TrkB ( ). The molecule 7,8-DHF can bind to the TrkB receptor with high affinity ( K d = ∼10 nM) and is able to pass the BBB, making it a viable alternative to BDNF in therapeutic treatment ( ). The binding of 7,8-DHF to the TrkB extracellular domain activates signal cascades that can last for hours without causing degradation of the internalized receptor ( ). In one study, the bioavailability of 7,8-DHF was about 5% with a half-life of 134 min given orally at 50 mg/kg ( ). 7,8-DHF is a selective TrkB agonist which is able to activate TrkB receptor without binding to p75 receptors, initiating signaling pathway that only influences neuroprotection and neurogenesis without activation of the apoptotic processes ( ). The binding of 7,8-DHF to the TrkB extracellular domain activates signal cascades that induce autophosphorylation of TrkB leading to activation of MAPK, PI3k/Akt, and ERK1/2 signal pathways in a time frame that was comparable to BDNF and in a dose-dependent manner ( ).
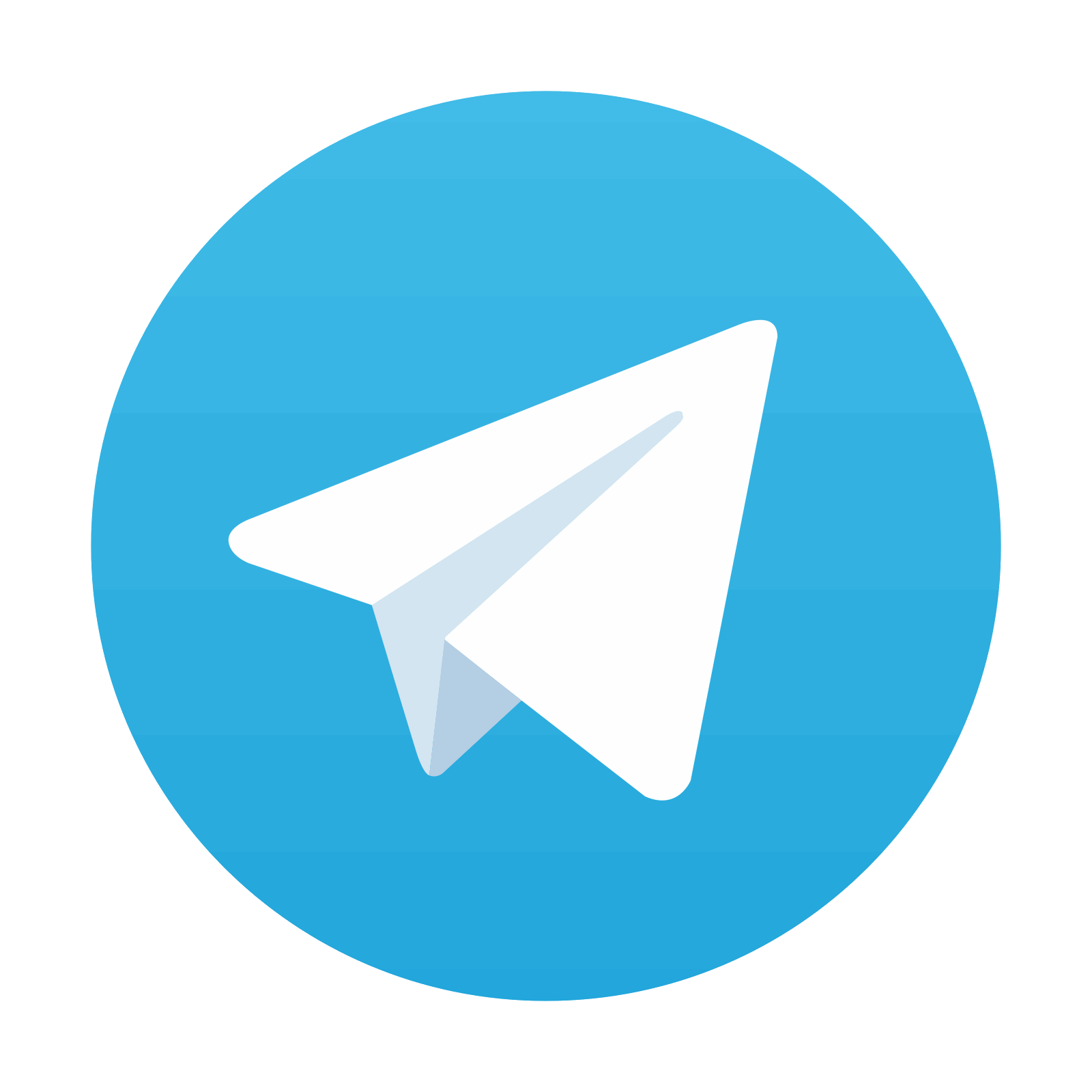
Stay updated, free articles. Join our Telegram channel

Full access? Get Clinical Tree
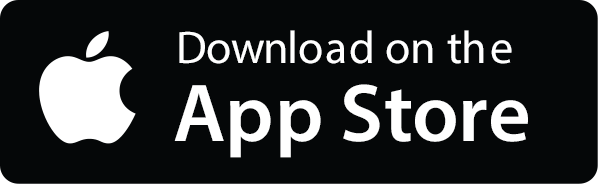
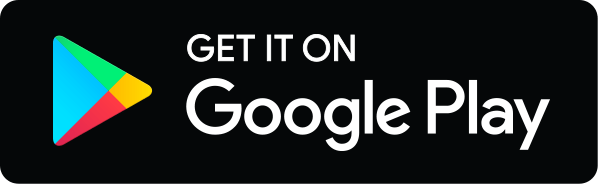