INTRODUCTION
Parkinson disease (PD) is a degenerative and progressive disorder characterized by the degeneration of the nigrostriatal pathway projecting to the striatum. All commonly employed therapies in PD have been focused on the replacement of brain dopamine levels by using dopaminergic drugs, which provide a significant clinical improvement. However, conventional therapies do not alter the progressive course of the disease, and many PD patients become severely disabled several years after the onset of the disease due to the appearance of nondopaminergic symptoms, as well as to drug-induced side effects. Over the last two decades, efforts have been oriented toward the development of new therapies that can provide symptomatic relief in advanced stages of the disease or delay its progressive course. Among these approaches, trophic factors and gene therapies are considered the most promising options.
GENE THERAPY
Gene therapy is the process of delivering nonspecific prosurvival genes to cells, in order to promote cell survival during pathologic conditions. The DNA of a therapeutic protein is inserted into a viral vector, which is administered and carried to a target area. Targeted cells then incorporate this DNA and produce the desired protein. These genetic modifications can modify the expression of specific genes by increasing or reducing them, or even restore the normal function of these genes product (1). Viral vectors are the most common carriers employed, since they are efficiently transported to the target structure. Virions are able to carry genetic information and can be designed according to the cells to be infected (2). The perfect viral vector for clinical use should fulfill several requirements (3). It should provide long-term expression of the transgene producing therapeutically relevant levels of protein, with minimal side effects. Moreover, when using a regulated vector, the expression needs to be dynamic, permanently controlled, and have a good safety profile. In these cases, the vector will be highly specific and efficient in transduction of target tissues during its “on” state, but during its “off” state, absence of protein expression is desirable.
The viral vectors used for gene transfer to target the central nervous system (CNS) are preferentially lentivirus (LV), adenovirus, adeno-associated virus (AAV), and herpes simplex virus (4). Adenoviruses have not been used in clinical trials, since they induce significant immunologic reactions (3). LV vectors are safer and very effective in transducing cells, but they insert the genetic material into the host genome. However, they have now been used for the first time in clinical studies in an open-label, phase I to II trial with no significant side effects (5). Recombinant AAV is a nonpathogenic neurotrophic virus, with low immunogenicity, which efficiently transduces postmitotic brain cells. As compared to LV, AAV vectors have demonstrated higher specificity at transducing certain brain areas, but one important limitation of these viruses is their capacity, which should not exceed 6 kb. When larger genes are required, adenovirus (8–30 kb) or herpes simplex virus (40–150 kb) is the best candidate. Twelve different serotypes of AAV have been described, and all are able to transduce cells in the brain. Serotype 2 was the first serotype to be discovered, but other serotypes have demonstrated higher efficiency and wider distribution when injected into the CNS.
In addition to viruses, nonviral gene therapy could be used to target specific cells by using receptor binding agents, ligands, peptides, or antibodies, avoiding immunogenic issues and insertions into the host genome. Nonviral gene therapy can be carried out using DNA/RNA complexes with carriers such as cationic agents, nanoparticles, cationic lipids and peptides, polymeric micelles, and receptor targeting peptides or proteins (2).
The fact that PD is due to the degeneration of a small, localized area of the brain makes this disorder a good candidate for gene therapy (6). Nevertheless, only a few vector-based gene therapy clinical trials have reached phase II (7). This might be related to the lack of animal models that reproduce the pathologic features of the disease, to the poor knowledge of the mechanism that underlies the neuropathologic abnormalities of PD and also because in most cases phase I clinical trials have failed to demonstrate relevant clinical benefit, probably because they include patients at advanced stages of the disease where the nondopaminergic features are prominent.
Gene therapy has been used in many ways to treat PD. Employing either recombinant AAV type 2 (rAAV2) or LV vectors, clinical trials in PD have been focused on three therapeutic approaches: augmentation of dopamine levels by increasing neurotransmitter production, modulation of the neuronal phenotype, and neuroprotection. To increase dopamine production, genes encoding the enzymes required for the synthesis of dopamine (amino acid decarboxylase [AADC], tyrosine hydroxylase [TH], and guanosine triphosphate [GTP] and cyclohydroxylase-1 [GCH1]) have been transferred to the striatal GABAergic neurons, providing a new source of dopamine in addition to increasing the efficiency of the remaining dopaminergic neurons. AADC is the enzyme responsible for the decarboxylation of levodopa to dopamine, and AADC levels are decreased in PD brains. Two phase I studies have assessed the efficacy and safety of AAV2-hAADC in PD patients. In both trials, AAV2-hAADC was unilaterally injected into the putamen of moderately affected PD patients. In these patients, the UPDRS (Unified Parkinson’s Disease Rating Scale) scores in “on” and “off” significantly improved; daily “off” time was reduced, and the positron emission tomography (PET) using the AADC tracer [18F] fluoro-L-m-tyrosine (FMT) showed increased AADC activity. Interestingly, motor benefit persisted up to 4 years, and no side effects including immunologic reactions were observed (9). These results support the long-term expression of the transgene as previously reported in MPTP-monkeys treated with AAV2-hAADC (8). However, it is not clear whether transduced GABAergic neurons can properly store, release, and metabolize dopamine. Hence, increased AADC activity in striatal nondopaminergic neurons would provoke unwanted side effects like dyskinesias, since dopamine would be released in a noncontrolled manner. In fact, abnormal movements have been described in preclinical studies with AAV2-hAADC in 1-methyl-4-phenyl-1,2,3,6 tetrahydropyridine (MPTP)-monkeys. Recently, a phase I trial (5) has reported a marked motor improvement in 15 PD patients after striatal delivery of LV containing three transgenes involved the synthesis of dopamine from tyrosine: TH, GCH1, and AADC (ProSavin®; Lenti-TH-AADC-GCH1). Although all these results are promising, it should be taken into account that they are phase I trials not specifically designed to prove efficacy, and therefore, placebo-controlled trials are mandatory before establishing the clinical benefit of these gene therapies in PD.
Loss of SN neurons elicits overactivity of the subthalamic nucleus neurons (SNT) and increased activity of the excitatory drive from the SNT to the globus pallidus medialis. The ablation and deep brain stimulation (DBS) of the subthalamic nucleus (STN) improve parkinsonism, indicating that phenotypic modulation of STN might be beneficial for PD patients. Accordingly, AAV2 virus has been used to delivery glutamic acid decarboxylase (GAD) to SNT neurons in order to convert excitatory glutamatergic neurons into inhibitory GABAergic cells. After several preclinical studies in rats and monkeys, a phase I trial and subsequently a double-blind study were undertaken in patients with advanced PD. In the phase II study, AAV2-GAD delivery into the SNT was compared to sham surgery. PD patients bilaterally injected with AAV2-GAD showed a marked and sustained motor improvement reflected by reduction in the UPDRS scores in “off-medication” situation. Motor fluctuations and freezing of gait also improved in some cases. No serious side effects were reported, with exception of those related to the surgery, thus confirming the safety profile of the virus (9).
Viral vectors can also provide neurotrophic factors (NTFs) to target areas to halt the progression of disease. Trials with gene therapy and NTFs are described in the next section.
NEUROTROPHIC FACTORS
Nowadays there is no cure for PD, and the disorder leads to severe disability several years after the diagnosis. For this reason, therapies with trophic factors arise as promising approaches to slow or prevent the progressive degeneration of nigral dopaminergic neurons.
NTFs are secreted polypeptides that activate specific cell receptors through binding and are grouped into families based on structural homology, receptors, and common signal transduction pathways (10). They have been described as having a role in neuronal differentiation, growth, and survival during development, and are essential in neuronal maintenance and protection in the CNS (11). NTFs increase in concentration against cell damage in an attempt to regenerate or rescue neuronal damage (12). Some of these NTFs have a broad effect on different cell populations, while others have a specific site of action and specific effects, such as neuroprotection or restoration for DA neurons in PD.
Table 50.1 summarizes the main results obtained in PD and animal models using NTFs.
GLIAL CELL LINE–DERIVED NEUROTROPHIC FACTOR
Glial cell line–derived neurotrophic factor (GDNF) is a member of the TGFb superfamily that signals via cell-surface tyrosine kinase receptors (RET) by binding to GFRα. GDNF was first purified from a rat glioma cell line medium (13) where it was shown that addition of GDNF to cultures of rat ventral mesencephalon increased the survival of DA neurons. Subsequent studies also demonstrated decreased apoptosis of TH-positive neurons in rat, monkeys, and ventral mesencephalon cultures (14,15) and also displayed a neuroprotective effect both in vitro and in vivo against dopaminergic toxins such as 6-hydroxydopamine (OHDA) and MPTP (16,17). There is strong evidence indicating that GDNF plays an important role in the survival and differentiation of dopaminergic neurons in culture and also in vivo. For instance, lentiviral delivery of GDNF and striatal carotid body grafts (18,19), which contain a large amount of GDNF, have been shown to induce an increase in the number of intrinsic dopaminergic neurons. Interestingly, GDNF knockout mice exhibited a progressive degeneration of the nigrostriatal pathway supporting the role of GDNF in maintaining the integrity of dopaminergic pathway (20). The neuroregenerative role of GDNF has been consistently demonstrated in animal models of the disease. Thus, lentiviral delivery of GNDF into the striatum of rats and MPTP-monkeys produced, in addition to motor recovery, markedly increased dopaminergic innervation in the denervated area (21).
In order to use NTFs in clinical practice, it should be taken into account that they are large proteins that do not cross the blood–brain barrier (BBB), so they must be infused directly or targeted by a carrier. GDNF has been directly administered into the brain of animals and PD patients using intracerebral infusions of recombinant proteins. Nutt et al. (22) performed the first randomized, double-blind study of GDNF in PD. In this study, GDNF was administered into the lateral ventricle. Patients receiving GDNF did not exhibit any motor improvement either in the “on” or in the “off” UPDRS scores after 8 months of treatment. In addition, severe side effects like nausea, vomiting, and anorexia occurred in many patients, and the postmortem analysis showed that GDNF did not reach the brain parenchyma. Subsequently, Gill et al. (23) performed phase I and II studies, administering GDNF through an intraputaminal cannula. The promising results of the phase I study in 5 patients encouraged the development of a double-blind study in 34 PD patients. Unfortunately, the marked improvement observed in the open study was not replicated in the double-blind study in spite the increased striatal uptake of 18F-dopa observed in patients receiving GDNF. The failure of the double-blind study relative to the open ones might be due to technical concerns, including the use of different-sized catheters to administer GNDF. It is also important to point that the double-blind study was prematurely halted due to the presence of antibodies against GDNF in 10% of the patients, thus precluding any subsequent motor benefit.
< div class='tao-gold-member'>
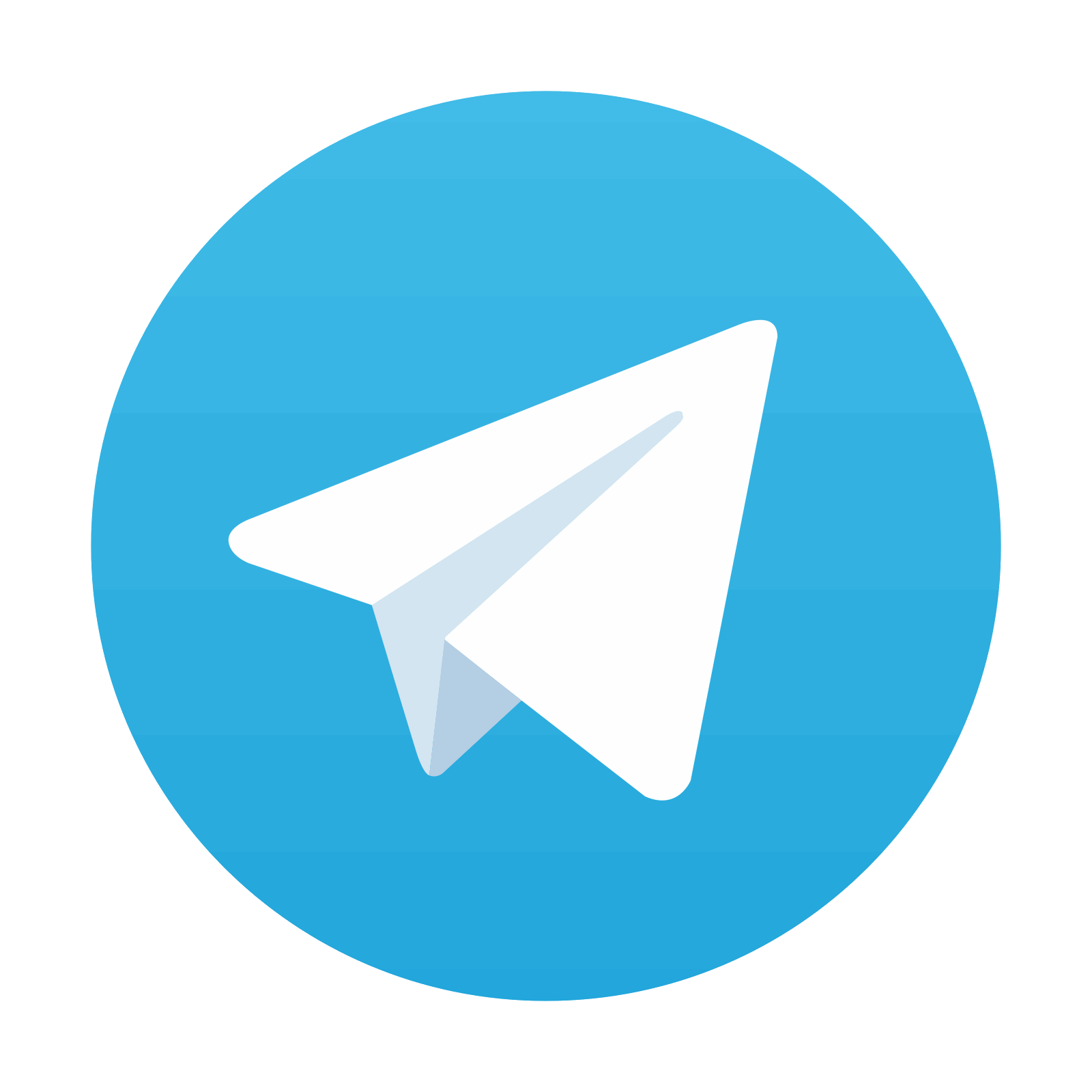