Fig. 23.1
MRI in the axial view showing a small left vestibular schwannoma extending to the cerebellopontine angle cistern. On T1- (a) and T2-weighted (b) images, the tumor appears isointense. Note the cerebrospinal fluid (CSF) collection between the tumor and the fundus of the internal auditory canal (IAC) (b). After gadolinium injection (c), homogeneous enhancement is typically observed
MR imaging with CISS sequences has also been applied for direct identification of cranial nerves in healthy individuals [28] and VS patients as well [29]. Recently, diffusion tensor imaging-based (DTI) fiber tracking was validated as a reliable tool for preoperative estimation of the position of the facial nerve in relation to large VSs [29]. In 91 % of patients, the position of the facial nerve was correctly predicted preoperatively. On the other hand, DTI was not useful for estimation of facial nerve morphological shape (compact or flat) [29]. This is a valuable information for surgical planning and facial nerve preservation which should be investigated in larger studies.
Computed tomography (CT) is still helpful for identifying bone destruction or expansion of the IAC [5, 30]. Besides, preoperative CT is important for surgical planning wherein the position of the labyrinth structures, their relationship to fundus, as well as the position of the sigmoid sinus and the emissary vein should be evaluated [5, 30]. Differential diagnosis comprises meningiomas, hemangiomas, and glomus tumors [5].
Evaluating NF2 patients, VSs are encountered in 96 % of them being mostly bilateral, although unilateral VS is also reported [17]. Tumors that appear multilobulated may designate a lesion of multiple nerve tumors arising from different nerves (from the vestibular, cochlear, and facial nerves) [31] (Fig. 23.2). Spinal tumors are more common than previously reported comprising 90 % of NF2 patients [17]. Meningiomas in 58 % of NF2 patients and trigeminal schwannomas in 29 % are also part of the clinical spectrum of the disease [17].
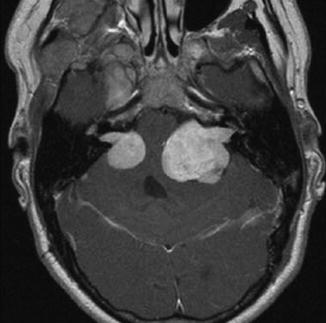
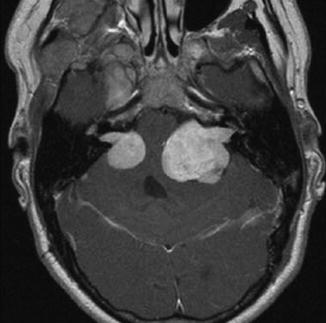
Fig. 23.2
Axial T1-weighted gadolinium-enhanced MRI of a NF2 patient revealing bilateral vestibular schwannomas, which is the hallmark of the disease. The tumor on the left side shows a rather lobulated aspect that may represent tumors arising from different cranial nerves
The patients are classified regarding the tumor extension as follows: class T1, purely intrameatal; T2, intra- and extrameatal; T3a, filling the cerebellopontine cistern; T3b, reaching the brainstem; T4a, compressing the brainstem; and T4b, severely dislocating the brainstem and compressing the fourth ventricle [3, 4, 8, 30, 32] (Figs. 23.3, 23.4, 23.5, 23.6 and 23.7) (Table 23.1). Patients affected by tumors larger than 4 cm in the maximum extrameatal diameter are considered to harbor giant lesions, which brings about a challenging situation for cranial nerve preservation due to severe nerve stretching [33].
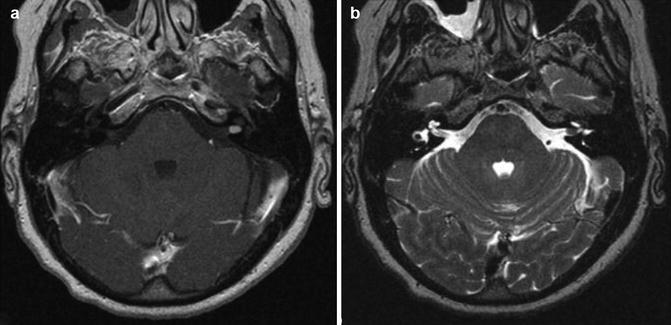
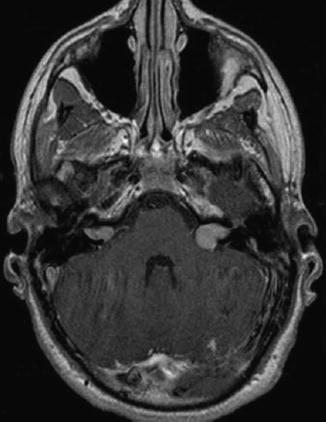
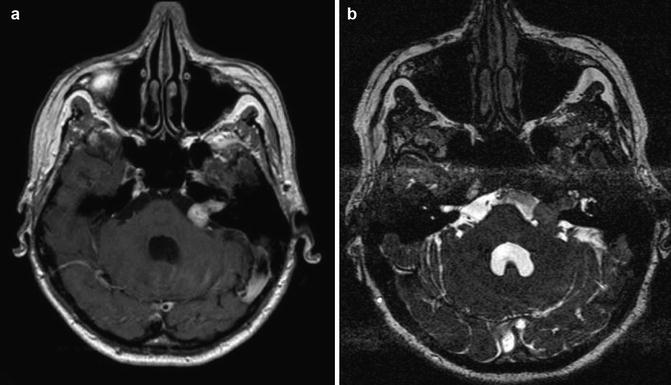
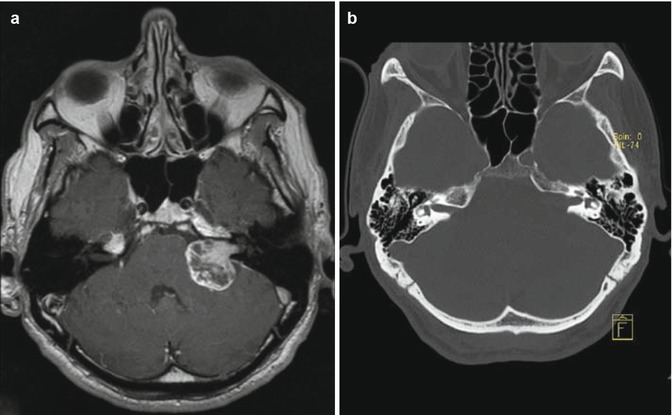
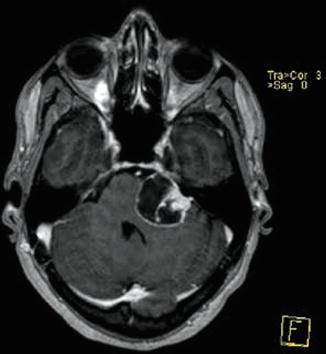
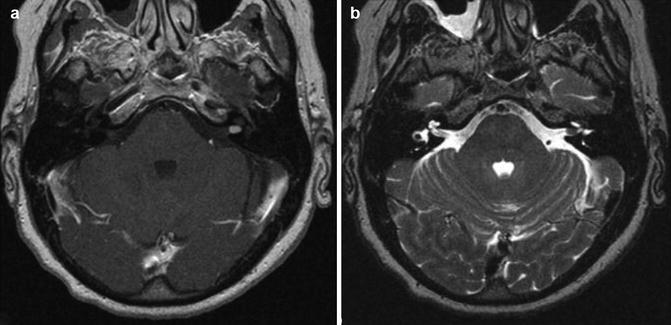
Fig. 23.3
Vestibular schwannoma Hannover class T1. Axial T1-weighted gadolinium-enhanced (a) and T2-weighted MRI (b) demonstrating a purely intracanalicular vestibular schwannoma on the left side. Note the rim of CSF between the tumor and the end of the IAC (b)
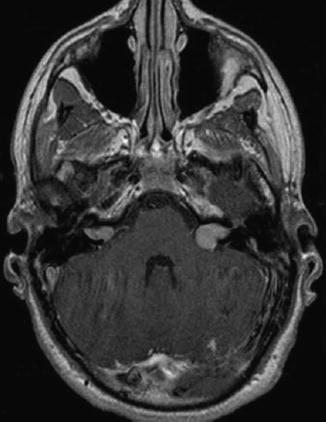
Fig. 23.4
Vestibular schwannoma Hannover classes T2 and T3a. Axial T1-weighted gadolinium-enhanced MRI in a patient affected of NF2 revealing an intra- and extrameatal tumor on the right side and a tumor that fills the cerebellopontine cistern on the left side
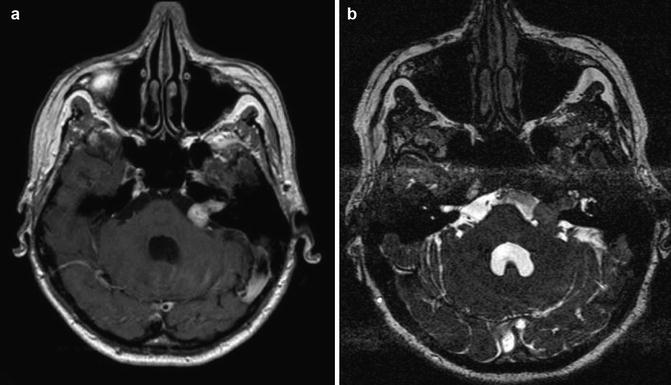
Fig. 23.5
Vestibular schwannoma Hannover class T3b. Axial T1-weighted gadolinium-enhanced (a) and T2-weighted (b) MRI showing a vestibular schwannoma on the left side that reaches the brainstem
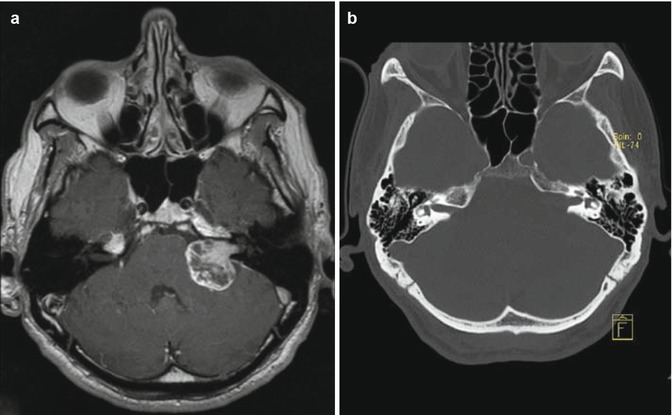
Fig. 23.6
Vestibular schwannoma Hannover class T4a. Axial T1-weighted gadolinium-enhanced MRI (a) demonstrating a large tumor that compresses the brainstem. Thin-slice bone window computed tomography (b) showing the typical bony expansion of the IAC
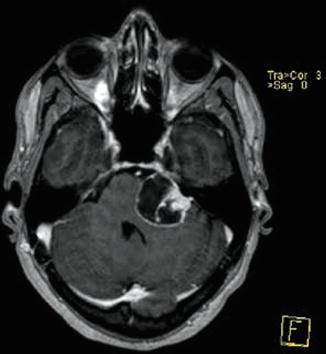
Fig. 23.7
Vestibular schwannoma Hannover class T4b. Axial T1-enhanced MRI revealing a large tumor that compresses and displaces the brainstem together with compression of the fourth ventricle. Note hypointense areas of cystic degeneration
Table 23.1
Hannover classification of tumor extension
Class | Extension |
---|---|
T1 | Purely intrameatal |
T2 | Intra- and extrameatal |
T3a | Filling the cerebellopontine cistern |
T3b | Reaching the brainstem |
T4a | Compressing the brainstem |
T4b | Dislocating the brainstem and compressing the fourth ventricle |
A complete audiological evaluation is performed based on patient’s pure tone audiogram (PTA), speech discrimination scores (SDS), and brainstem auditory evoked potentials (BAEP). VS may cause hearing loss of any frequency; however hearing loss affecting high frequencies is the most common audiometric finding [15, 23, 34]. Flat loss, trough-shaped loss, and low tone loss have been also reported in evaluating PTA of patients suffering from VS [15, 23, 34]. High-frequency hearing loss together with a marked reduction in SDS is a landmark sign of an eighth nerve lesion, such as VS [34]. The auditory function is analyzed by using the new Hannover classification, as follows: H1 (normal hearing), 0–20 dB and 95–100 % SDS; H2 (useful hearing), 21–40 dB and 70–94 % SDS; H3 (moderate hearing), 41–60 dB and 40–69 % SDS; H4 (poor hearing), 61–80 dB and 10–39 % SDS; and H5 (no functional hearing), >80 dB and 0–9 % SDS [8] (Table 23.2).
Table 23.2
New Hannover classification of hearing function
Hearing class | Audiological evaluation |
---|---|
H1 (normal hearing) | 0–20 dB and 95–100 % SDS |
H2 (useful hearing) | 21–40 dB and 70–94 % SDS |
H3 (moderate hearing) | 41–60 dB and 40–69 % SDS |
H4 (poor hearing) | 61–80 dB and 10–39 % SDS |
H5 (no functional hearing) | >80 dB and 0–9 % SDS |
BAEP is considered to be the most sensitive audiological test for diagnosis of VS [15, 32]. Nevertheless, a lower sensitivity is expected in patients with normal audiological findings [21] showing additionally a significant variation with tumor size [20]. In tumors smaller than 1 cm, the BAEP’s wave morphology was abnormal solely in 76.5 % of patients, whereas in tumors larger than 2 cm, it was abnormal in 100 % [20]. Given the relevance of early diagnosis of VS along with the aforementioned lower sensitivity, MRI is recommended whenever there is clinical suspicion of VS [20].
The patients are further classified according to Nordstadt’s BAEP typing system, consisting of types B1 to B5 [32] (Table 23.3). Preoperative prediction of hearing preservation may be more accurately evaluated when combining clinical data from the patients’ audiometry, the radiological data of tumor extension, and neurophysiological parameters [32]. These data are currently used for patient counseling regarding timing of surgery [32]. Patients suffering from small tumors, together with good to fair hearing and BAEP types B1 and B2, attain the best hearing preservation rates [32]. The House-Brackmann scale is used to assess facial function [35].
Table 23.3
Nordstadt BAEP classification system
BAEP types | BAEP waves |
---|---|
B1 | Waves I, III, and V are present |
Latency I–III is normal or slightly increased (within two standard deviations, 2.1 ± 0.28 ms) | |
B2 | Waves I, III, and V are present |
Latency I–III is severely increased (>2.66 ms) | |
B3 | Wave III is lost |
B4 | Solely wave I or wave V is present |
B5 | All waves are lost |
23.5 Treatment Options
The treatment comprises clinical and radiological observation, surgery, and radiation therapy. The patients are advised of all treatment modalities and the decision is taken together with the medical team.
The conservative management is a viable treatment alternative for VS being advocated by some authors in selected cases such as patients with advanced age, poor general health, small tumors with minimal or no symptoms (especially intracanalicular), tumors in the only or better-hearing ear, NF2, patient preference, and any combination of these [36–42]. Nevertheless, the conservative management carries a significant risk of hearing loss [36–42] even in nongrowing tumors [43] but particularly in those tumors with a growth rate greater than 2.5 mm/year at any point during follow-up [44, 45]. Therefore, when hearing preservation is considered, earlier intervention should be taken into account [36, 37]. Besides, the chances of hearing preservation are better in cases of short symptom duration [8, 31]. In addition to hearing deterioration, loss of patient’s compliance should be considered in choosing the conservative management [36, 39, 42].
In a systematic review, Sughrue et al. [44] analyzed 982 untreated patients and described a mean growth rate of 2.9 ± 1.2 mm/year in a 26–52-month period. A recent prospective study of 178 untreated small VS compared three different growth models, namely, a mm/year-based model, a cm3/year-based model, and a volume doubling time (VDT)-based model [46]. Varughese et al. [46] observed that the mean growth rate was of 0.66 mm/year, 0.19 cm3/year, and a VDT of 4.4 years, which was found as the most realistic VS growth model indicating a threshold of 5.22 years as the cutoff point to distinguish growing from nongrowing tumors [46].
23.5.1 Surgery
The surgical indications comprise, as follows:
Large tumors (brainstem compression)
Cystic tumors
Young patients (<60 years)
Vestibular-related main symptoms
Bilateral tumors in NF2 patients
Patient’s individual decision
Concerning NF2 patients, the first surgery is recommended as soon as possible. If hearing preservation is not a goal, the surgeon may adopt a waiting attitude for the second tumor while the patients are scheduled for lip-reading learning. On the other hand, if hearing preservation is a goal, the second surgery is planned for the next 2 or 3 months at which total or deliberate subtotal resection may be performed with the assumption of function preservation, particularly in the last hearing ear.
VSs may be operated on by the retrosigmoid approach, the middle fossa approach, and the translabyrinthine approach.
The risk implied for patients is minimized if the surgeon and the team use the approach with which they are most familiar. Samii and Matthies [8]
We perform solely the retrosigmoid approach at our department for VS because it provides a wide exposure of the cerebellopontine angle (CPA) allowing superior and inferior extensions of the approach for treating multiple tumors within a single procedure (as occur in NF2 patients), as well as preservation or reconstruction of the cranial nerves [8].
The operative nuances of the retrosigmoid approach for VS resection and auditory brainstem implant (ABI), as well as the preoperative and postoperative precautions, were detailed in Chap. 9.
23.5.1.1 Intraoperative Monitoring (IOM)
Cochlear Nerve Monitoring
The introduction of intraoperative neurophysiological monitoring technique for the auditory function emerged in the 1970s and at the beginning of the 1980s together with the first attempts of hearing preservation in CPA surgery [32, 47].
The goal of intraoperative auditory function monitoring is to provide information about the auditory pathway status during surgery in order to prevent damage to the auditory function [48] and consequently hearing loss. Early detection of the damage through a near-real-time recording technique is required for the electrophysiological method to alert the surgical team, resulting in immediate interruption or change of the microsurgical maneuvers with the assumption for reversal of the damaging process. This may enable wave recovery sparing hearing loss [48, 49].
The brainstem auditory evoked potential (BAEP) is the most widely used intraoperative electrophysiological method for auditory function monitoring, because it has a high sensitivity [48] and reliability to detect cochlear nerve damage [50]. However, BAEP requires 30 s up to 4 min to deliver reliable response of wave changes [34, 49], which is a disadvantage of the method. In this interim, a permanent damage to the cochlear nerve could happen, preventing the surgical team from detecting and avoiding it [48].
Direct auditory nerve manipulation [49, 51, 52], cerebellar retraction [49, 51, 52], and drilling of the internal auditory canal [49] are identified events that lead to intraoperative BAEP changes and, ultimately, could intervene with the postoperative hearing outcome.
During intraoperative BAEP monitoring, three changes can happen, as follows: latency increase, amplitude decrease, and wave loss [49]. Usually, latency increase and amplitude decrease are observed in the way to wave loss [49].
“When to warn the surgeon?” still is a matter of debate between authors. Empirical and divergent warning criteria have been used for different electrophysiological teams to warn the surgeon about deteriorating BAEP: (a) latency increase of 0.3 ms or amplitude decrease of 50 % [49], (b) latency increase of wave V of 0.5 ms [48], (c) latency increase of wave V of 0.07 ms/min or more than 1.5 ms (absolute value) [51], or (d) latency increase of wave V of 1.0 ms and amplitude decrease of 50 % [48, 53, 54].
Latency increase of wave V of 1.0 ms and amplitude decrease of 50 % are the most widely used criteria [48, 53, 54]. However, the observance of hearing impairment in CPA tumor surgeries following increased latency of less than 1 ms together or not with amplitude decrease smaller than 50 % of wave V showed that these criteria may be not sensitive enough as “warning sign” for hearing preservation in this group of patients [54]. Conversely, in patients affected of nonneoplastic CPA diseases, such as neurovascular compression (trigeminal neuralgia, facial hemispasm, etc.), these criteria seemed to be too sensitive in the form that latency increase of wave V of 1.0 ms and amplitude decrease of 50 % or even wave V loss did not predict hearing loss in this group of patients [54].
So, the reasonable answer for that question would be: “It depends on the disease that you are dealing with” [53, 54]. For CPA tumor a more strict criteria should be used and, therefore, we use latency increase of more than 0.3 ms or amplitude decrease greater than 50 % as our reference in such surgeries [49].
As previously reported by Matthies and Samii [49], each I, III, or V temporary or permanent wave loss carries a risk of deafness, as follows: for wave I loss is 65 %, for wave III loss is 65 %, and for wave V loss is 78 %. Whether these losses are concomitant, that is, permanent waves I, III, and V loss or type B5, it is associated with 96 % of deafness postoperatively [49]. The loss of wave III is the most precocious and sensitive sign for predicting postoperative deafness, whereas loss of wave V is the most definitive sign of postoperative deafness [49]. Other authors also emphasize the relevance of waves I and V loss for hearing preservation, alone or in combination [51, 52].
BAEP stability during CPA tumor surgeries indicates high hearing preservation rate [52, 55]. Patients with preoperative types B1 and B2 have a higher rate of hearing preservation (up to 80 %), whereas those with types B4 and B5 have reduced chances of hearing preservation (12–17 %, respectively) [32].
BAEP is performed throughout the procedure at our department in all patients that have types B1 to B4 BAEPs being the microsurgical maneuvers modified if BAEP changes happen that are known to have impact on postoperative hearing.
Facial Nerve Monitoring
Even though there is an increasing rate of reported facial nerve preservation following VS surgery [56], facial nerve paresis or paralysis remains a significant risk to the patients [57], being frequent postoperative sequelae of major concern [56]. Indeed, the increasing rates of facial nerve preservation are supported by the introduction of routine intraoperative facial nerve monitoring [56–59]. Conversely, some authors believe that monitoring serves only to speed up the dissection thereby reducing the operating time substantially [5].
Various monitoring techniques have become routine in dealing with complex cranial base lesions [57]. Direct electrical stimulation, continuous electromyography (EMG), and facial motor evoked potential (fMEP) are currently useful adjuncts to CPA surgery [57–60].
Direct electrical stimulation is performed by using a bipolar stimulation probe and the responses are recorded in the subdermally placed needle electrodes into the ipsilateral orbicularis oculi and orbicularis oris. The stimuli have the purpose to directly identify the facial nerve fibers over the tumor surface [58]. Hence, dissection can be performed without risking the flattened nerve bundle [58]. Besides, the stimuli may be intermittently applied assuring the surgeon that the nerve is preserved distal to stimulation [58]. Compound muscle action potentials comparing the response from stimulation at the brainstem to the response at the internal auditory canal may be predictive of functional integrity of the facial nerve [57]. Minimal stimulus intensity of 0.05 mA or less together with a response amplitude of 240 μV or greater is able to predict House-Brackmann grades I and II outcome in 85 % of patients at 1-year follow-up [59]. Disadvantages of the method include its intermittent use and difficulty to locate the facial nerve at the brainstem in large tumors [57, 58].
Continuous EMG is better than direct electrical stimulation alone, improving the rate of facial nerve preservation during cranial base surgery [57, 58]. The spontaneous facial activity registered by EMG shows five typical waveforms that could be classified into spikes, bursts, and A, B, and C trains [58]. A-trains are sustained periodic EMG activities presenting a sinusoidal pattern that produce a high-frequency sound from the loudspeaker [58]. They occur following tumor dissection in the vicinities of the facial nerve, as well as dissection nearby the brainstem and intrameatal decompression [58]. A-trains appearance has a sensitivity of 86 % and a specificity of 89 % in demonstrating postoperative facial function deterioration [58]. In that study, however, a correlation between the number of A-trains and postoperative facial function could not be demonstrated [58]. Thereafter, Prell et al. [61] showed that a quantitative EMG parameter, namely, the train time, is a reliable indicator of postoperative facial palsy. Interestingly, 2 time thresholds are described [61]. For patients with normal preoperative function, a train time of <0.5 s is strongly correlated with a good
postoperative outcome, whereas the 10-s time threshold is associated with facial deterioration in patients with normal preoperative function and those affected by preoperative facial palsy [61].
It is worth mentioning that both studies provided an offline analysis of the intraoperative findings so that waveforms are evaluated retrospectively after the end of the surgical procedure. Recently, the study was continued by developing software capable of recognizing and quantifying A-trains in real time [62]. The software shows train time activity continuously through a monitor inlet in the surgeon’s view depicted as a traffic light display (green, < 0.125 s; yellow, 0.125–2.5 s; red, 2.5–10 s; and black, > 10 s) [62]. Such threshold levels were compared with those obtained in the offline analysis, rendering lower amounts of time by a factor of 4, in which 0.5 s of offline analysis corresponded to 0.125 s of real-time analysis. For patients with normal preoperative facial function, train time activity lower than 0.125 s was strongly correlated with good postoperative outcome, whereas 2.5-s and 10-s thresholds mostly indicated good postoperative function and marked dysfunction, respectively [62]. Conversely, for patients with preoperative facial dysfunction, only the 2.5-s threshold was defined indicating favorable or unfavorable facial function. The surgical strategy was modulated according to intraoperative findings in a way that subtotal or near-total resection was considered when the red and black thresholds were achieved. This situation occurred in 4 of 30 studied patients, whereas in the other 4 patients, threshold levels were exceeded, but the surgical procedure was continued because of the younger age [62]. That study is unique due to first-time demonstration of real-time FN monitoring with interpretation independent from the electrophysiological team. It may contribute in the near future to increasing reliability of facial function prediction during surgical procedures. Nonetheless, a major limitation is that special software is necessary for either offline or real-time analysis.
As aforementioned, both methods have limitations which have stimulated the development of alternative techniques for facial nerve monitoring. Facial motor evoked potential (FMEP), obtained by transcranial electrical stimulation (TES), has recently been introduced and may be considered the most promising method for facial nerve monitoring [57, 63–66] because it surpasses most of the disadvantages of standard techniques, namely, direct electrical stimulation and free-running EMG [65]. FMEP interpretation is independent from the surgeon’s ability to locate the proximal facial nerve at the brainstem and can facilitate recognition of waveform recordings obtained by free-running EMG [63, 64]. FMEP can be performed throughout the procedure at the demand of the surgeon, as well as following neurotonic discharges recorded by EMG or after extensive dissection on the facial nerve [57].
FMEP amplitude ratio reduction of 50 % at the end of the surgery has been identified as a good predictor for postoperative facial nerve outcome following CPA and skull-base surgeries [57, 63–65]. However, by calculating final-to-baseline amplitude ratios, only initial and final FMEP amplitude values are considered for function prediction. We hypothesized that intraoperative variation of FMEP amplitude could correlate with postoperative facial function, even if final-to-baseline amplitude ratios remain above the 50 % cutoff level. With this assumption, we have demonstrated the correlation of intraoperative FMEP deterioration with immediate and late postoperative outcome justifying changes in surgical strategy in order to avoid definitive facial nerve injury. Therefore, event-to-baseline FMEP monitoring is a better predictor of facial function than final-to-baseline isolated measurements [67].
In addition, amplitude has been the only quantitative criterion used to date for interpretation of FMEP [67]. Thus, we also investigated the intraoperative changes of the FMEP waveform morphology and their correlation to postoperative facial function [67]. We found that waveform complexity seems to represent an additional, independent quantitative parameter that can be used for FMEP monitoring, providing excellent results for prediction of postoperative facial function [67].
Just as for cochlear nerve monitoring, the surgical steps are modified or even interrupted if facial nerve changes happen that are known to be significant in postoperative deterioration of facial function. Table 23.4 summarizes prediction criteria for facial nerve monitoring which are related to a satisfactory functional outcome.
Table 23.4
Summary of current standard facial nerve intraoperative monitoring and their criteria to indicate satisfactory functional outcome postoperatively
Facial nerve intraoperative monitoring modality | Prediction criteria |
---|---|
Direct electrical stimulation | 1. CMAP absolute amplitude (brainstem stim ≤0.05–0.6 mA and amplitude ≥100–500 μV) |
2. Minimal stimulation threshold (brainstem stimulation ≤0.05–0.1 mA) | |
3. Proximal-to-distal amplitude ratio (brainstem/meatus ≥30 %) | |
Free-running EMG | 1. A-trains occurrence |
2. A-trains time (<0.5 s) | |
3. A-trains real time (<0.125 s and <2.5 s)
![]() Stay updated, free articles. Join our Telegram channel![]() Full access? Get Clinical Tree![]() ![]() ![]() |