1 Neuroembryology Knowledge of nervous system development can provide the foundation for understanding nervous system structure and function. This chapter describes early development of the nervous system and then discusses development of the spinal cord and brain. The main malformations due to abnormal nervous system development are also discussed. Table 1.1 summarizes the embryonic elements of the nervous system and their derivatives in the adult. See Fig. 1.1. Formation of the nervous system begins during the third week of gestation, when the neural plate develops from a thickening of the embryonic ectoderm. A longitudinal neural groove, bounded by two neural folds, forms along the midline of the plate. Fusion of the neural folds, which meet along the midline, proceeds in both cranial and caudal directions, gradually converting the grooved plate into the neural tube, which comes to lie below the surface ectoderm. The process of neural tube formation (neurulation) occurs simultaneously with the separation of the neu-roectoderm from the surface ectoderm, a process termed disjunction. Disjunction results in a separation of the future nervous system from the future skin. Failure to complete the process of disjunction (nondisjunction) and completion of disjunction prior to neural tube closure (premature disjunction) are two sources of spinal dysraphism. Before the neural tube closes completely during the fourth week of embryonic development, it remains in communication with the amniotic cavity through the anterior and posterior neuropores. The anterior neuropore closes between gestational days 23 and 25; the posterior neuropore closes between gestational days 25 and 27. Along the lateral margins on either side of the neu-roepithelial cells of the neural plate are two strips of cells that pinch off from the neural groove as it forms the neural tube. These neural crest cells eventually occupy a dorsolateral position between the surface ectoderm and the neural tube. Most of the peripheral nervous system is derived from the neural crest, including sensory ganglion cells of the cranial and spinal nerves, autonomic ganglia, and Schwann cells. Neural crest cells give origin to the adrenal medulla and melanocytes as well (Table 1.2). Thickening of the neural tube walls gives form to the brain and the spinal cord, whereas the lumen of the tube becomes the ventricular system and the central canal. The neuroepithelial cells that form the walls of the neural tube give origin to neurons and macroglia (i.e., astrocytes, oligodendrocytes, and ependymal cells). The microglia are derived from cells of mesodermal origin that enter the central nervous system (CNS) from the vasculature during development (Table 1.2). Fig. 1.1 Early neural development.
Early Neural Development
Neural Tube Derivatives | Neural Crest Derivatives |
Ventral horn cells | Cranial ganglia |
Preganglionic autonomic neurons | Dorsal root ganglia |
Astrocytes, oligodendrocytes, and ependymal cells | Autonomic ganglia |
Retina | Schwann cells |
Posterior pituitary | Adrenal medulla |
Cortical neurons | Melanocytes |
Gray nuclei of the brain and spinal cord |
Early Development of the Spinal Cord
See Fig. 1.2.
Three layers of cells are formed from the proliferation and differentiation of the thick, pseudostratified neuroepi-thelium that makes up the wall of the neural tube. Beginning from inner- to outermost, these are the neuroepithe-lial layer, the mantle layer, and the marginal layer.
On the innermost aspect of the neural tube, the neu-roepithelium forms a layer of ciliated columnar cells, the neuroepithelial (or ependymal) layer, that lines the future ventricles and central canal.
The neuroepithelium also gives rise to primitive neurons, called neuroblasts, that migrate peripherally to surround the neuroepithelial layer. This so-called mantle layer will later form the gray matter of the spinal cord.
As the neuroblasts in the mantle layer develop into mature neurons with cytoplasmic processes, these processes extend peripherally to form the outermost marginal layer that later becomes the white matter of the spinal cord.
Astrocytes and oligodendrocytes are also derived from precursor blast cells that originate in the neuroepithelial layer and migrate peripherally into the mantle and marginal layers.
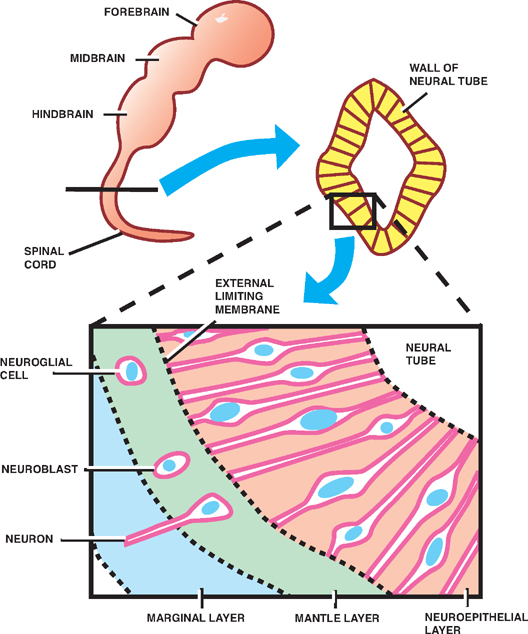
Fig. 1.2 Early development of the spinal cord.
Spinal Gray Matter
See Fig. 1.3.
Thickening of the dorsal and ventral aspects of the neural tube produces the alar and basal plates, respectively. Together, these plates represent the future gray matter of the spinal cord. These dorsal and ventral bulges are separated by a longitudinal groove, the sulcus limi-tans, that develops along the sides of the central cavity.
The alar plate forms the dorsal gray columns, which contain sensory afferent neurons. In cross section, these columns are referred to as the dorsal horns. The basal plate contains somatic and autonomic motor neurons that constitute the ventral and lateral gray columns, respectively. In cross section, these columns are known as the ventral and lateral horns.
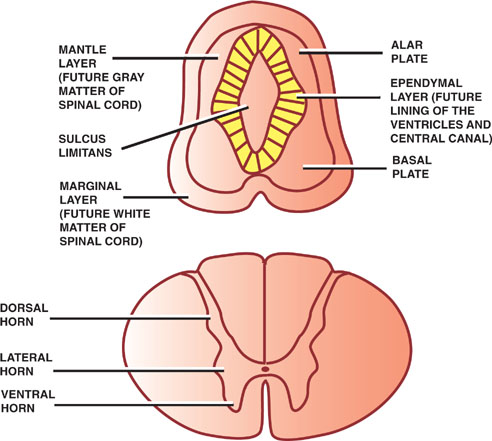
Fig. 1.3 Spinal gray matter.
Ventral and Dorsal Roots
See Fig. 1.4.
Sensory neurons in the dorsal root ganglia are derived from the neural crest. These pseudounipolar neurons project both central and peripheral branches (axons).
The central branches of the dorsal root ganglia enter the spinal cord through the dorsal sensory roots. They either synapse in the dorsal gray column (spinothalamic tract) or ascend in the dorsal white column to terminate in the dorsal column nuclei (dorsal column-medial lem-niscus tract). Neurons in the dorsal gray column and the dorsal column nuclei are derived from the alar plate.
The peripheral branches of the dorsal root ganglia enter the spinal nerves, course peripherally, and terminate as sensory endings in somatic or visceral structures.
Motor neurons in the ventral gray columns are derived from the basal plate. They project axons peripherally into the ventral motor roots.
Somatic motor neurons in the ventral motor roots join peripheral branches of the dorsal root ganglia in the region of the intervertebral foramina to form the spinal nerves. Sympathetic motor neurons in the ventral motor roots also join the spinal nerves but exit soon after in the white communicating ramus to reach the paravertebral and prevertebral ganglia.
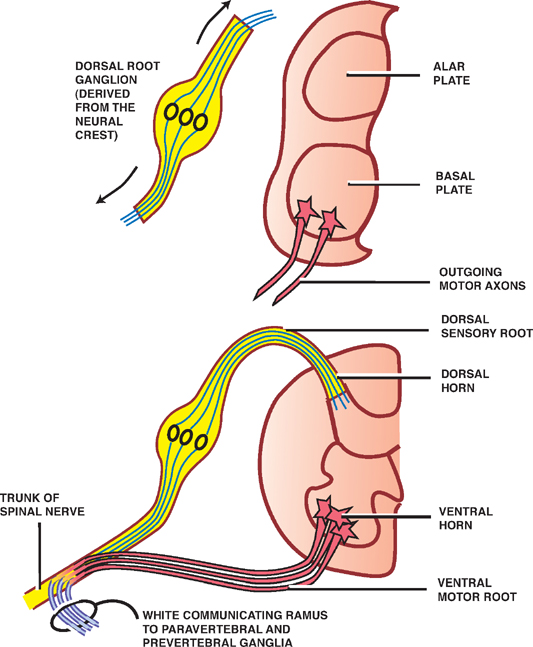
Fig. 1.4 Ventral and dorsal roots.
Ascent of the Conus Medullaris
See Fig. 1.5.
During the early stages of development, the rate of growth of the spinal cord keeps pace with that of the vertebral column; thus, the spinal nerves pass through the intervertebral foramina at their respective level of origin in the spinal cord.
After the third month of embryonic development, however, the rate of growth of the vertebral column exceeds that of the spinal cord so that the end of the spinal cord assumes an increasingly higher position in relation to the vertebral column. In the adult, the caudal end of the spinal cord, called the conus medullaris, is positioned at the level of the first lumbar vertebra. The conus medullaris is attached to the periosteum of the coccygeal vertebrae by a long thread of pia mater known as the flum terminale. Because of the differential rate of growth of the spinal column and spinal cord, the spinal cord segment does not correlate with the respective vertebral column levels. In the cervical spine, each vertebral level corresponds to the level of the succeeding cord segment (i.e., the sixth cervical spine corresponds to the level of the seventh spinal cord segment). In the upper thoracic spine, the difference is two segments, and in the lower thoracic and upper lumber spine, the difference is three segments.
Because all spinal nerves pass through their corresponding intervertebral foramina, the lumbar and sacral roots are considerably stretched. These lengthy fibers constitute the cauda equina (L. horse’s tail).
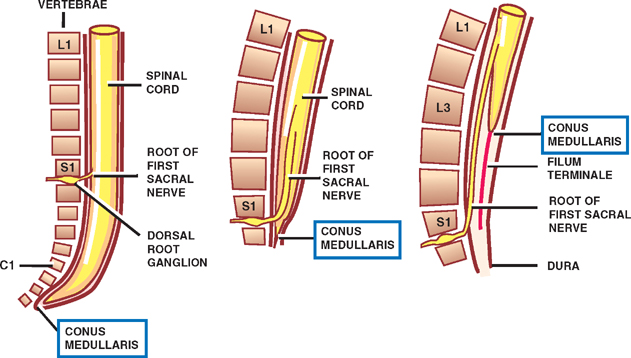
Fig. 1.5 Ascent of the conus medullaris.
The Brain Vesicles
See Fig. 1.6.
During the fourth week of gestation, the rostral neural tube takes the form of three primary brain vesicles: the forebrain or prosencephalon, the midbrain or mes-encephalon, and the hindbrain or rhombencephalon. During the fifth week, the forebrain divides into the tel-encephalon and diencephalon, the midbrain becomes the mesencephalon, and the hindbrain divides into the met-encephalon and the myelencephalon, resulting in the formation of fve secondary brain vesicles.
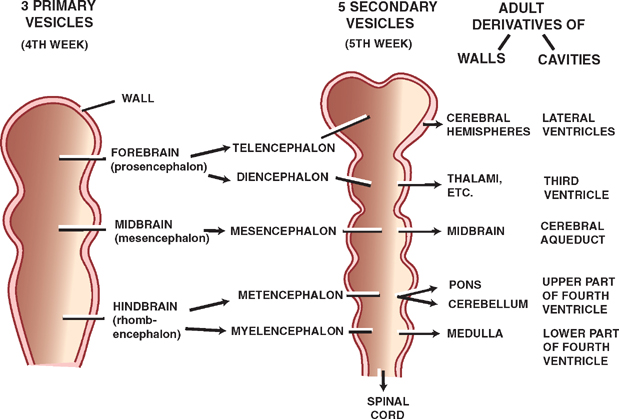
Fig. 1.6 Brain vesicles.
The Brain Flexures
See Fig. 1.7.
As the primary brain vesicles develop, the brain flexes, or bends, to form the cephalic flexure in the midbrain region, and the cervical flexure at the junction of the hind-brain and the spinal cord. A compensatory pontine flexure later forms between the cephalic and cervical flexures.
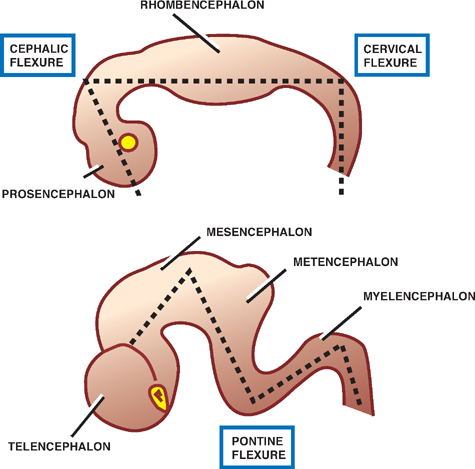
Fig. 1.7 Brain flexures.
The Rhombencephalon (Hindbrain)
The cervical flexure marks the junction between the spinal cord and the hindbrain. The hindbrain is divided by the pontine flexure into the myelencephalon (the future medulla) and the metencephalon (the future pons and cerebellum).
The central cavity of the hindbrain becomes the fourth ventricle, the dorsal surface of which is bounded by an ependymal roof plate. The roof plate is formed as the fourth ventricle expands, spreading its lateral walls open like the pages of a book.
As a result of this change, the roof plate of the fourth ventricle, which is covered by vascular pia mater, is stretched and greatly thinned. Together, the roof plate and the vascular pia mater constitute the tela choroidea. Invagination of the tela choroidea into the cavity of the fourth ventricle forms the choroid plexus, which is responsible for the secretion of cerebrospinal fluid (CSF). Similar plexuses develop in the third and lateral ventricles.
CSF flows out of the fourth ventricle through two lateral apertures (the foramina of Luschka) and one median aperture (the foramen of Magendie) that are formed as local resorptions of the roof of the fourth ventricle.
Another change produced by the spread of the lateral walls of the fourth ventricle is that the alar plates assume a lateral position in relation to the basal plates. This explains why sensory neurons (derivatives of the alar plates) lie lateral to motor neurons (derivatives of the basal plates) in the pons and medulla, in contrast to their dorsal-ventral relation in the spinal cord.
The Myelencephalon
See Fig. 1.8.
The myelencephalon develops into the medulla ob-longata. Many of the structural similarities between the medulla and the spinal cord, with which it is continuous, disappear during development as the fourth ventricle expands (see earlier discussion).
Neuroblasts of the alar plate develop into sensory nuclei; neuroblasts of the basal plate develop into motor nuclei. Some neuroblasts of the alar plate migrate ventrally to form isolated areas of gray matter, including the inferior olivary nuclei, which are associated with the cerebellum, and the gracile and cuneate nuclei, which are associated with the dorsal column-medial lemniscus tracts. On the ventralmost aspect of the caudal medulla are the medullary pyramids, which contain the cortico-spinal tracts.
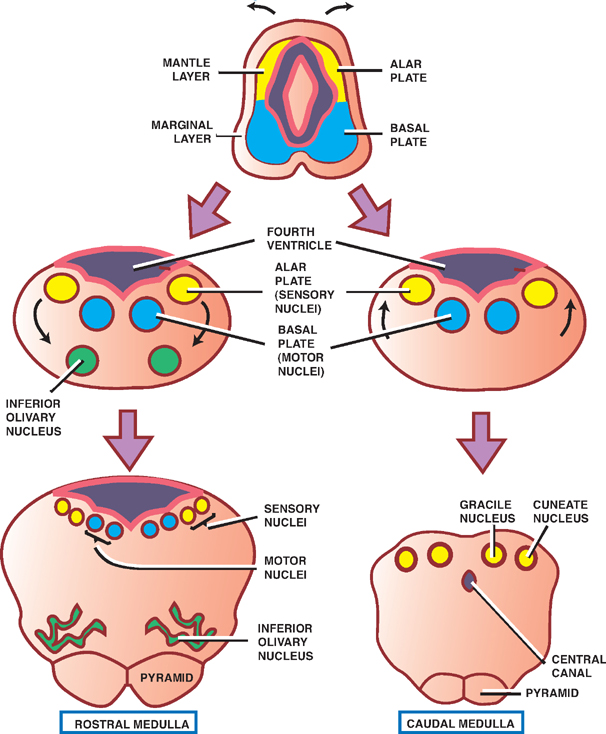
Fig. 1.8 The myelencephalon.
The Metencephalon
See Fig. 1.9.
The dorsal part of the metencephalon develops into the cerebellum, and the ventral part develops into the pons.
The cerebellum is formed from the fusion of dorsolat-eral thickenings of the metencephalon that overgrow the roof of the fourth ventricle. These thickenings, or rhombic lips, fuse in the midline to form the cerebel-lar vermis, which is fanked on either side by enlarging cerebellar hemispheres. Peripherally migrating neuroblasts contribute to the cerebellar cortex, whereas those situated centrally differentiate into the deep intracerebel-lar nuclei.
Development of the pons occurs ventral to the cerebellum in the ventral aspect of the metencephalon. The pontine nuclei, whose axons project to contralateral cere-bellar cortices, come to lie in the ventral pons; the dorsal pons contains the cranial nerve nuclei. As in the myelen-cephalon, motor cranial nerve nuclei are derived from the basal plate; sensory cranial nerve nuclei are derived from the alar plate.
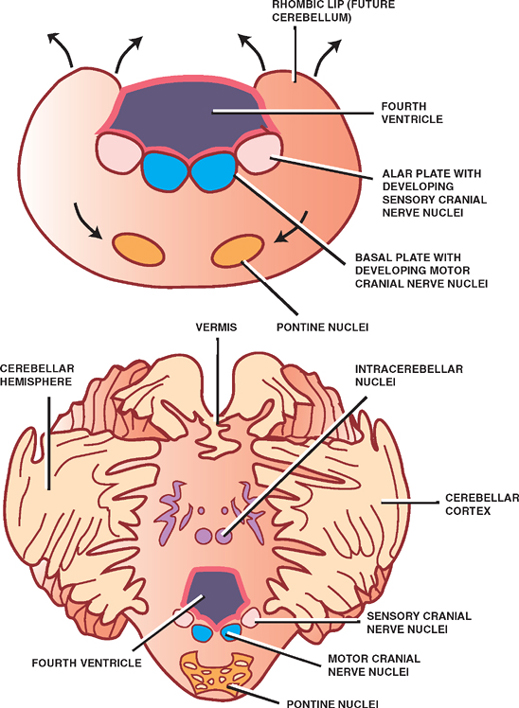
Fig. 1.9 The metencephalon.
The Mesencephalon
See Fig. 1.10.
Of all the parts of the brain, except the caudal hind-brain, the midbrain undergoes the least dramatic change during development. The central cavity of the midbrain forms the cerebral aqueduct of Sylvius, which connects the more expansive third and fourth ventricles.
Neuroblasts from the alar plates migrate into the roof, or tectum, of the midbrain to form the inferior colliculi, which are concerned with audition, and the superior col-liculi, which are concerned with visual reflexes. These collections of cells produce four bulges on the dorsal surface of the midbrain, known as the quadrigeminal plate. The central gray surrounding the aqueduct is also derived from neuroblasts of the alar plates.
Neuroblasts from the basal plates give rise to several groups of neurons in the tegmentum of the midbrain, comprising the oculomotor (III) and trochlear (IV) cranial nerve nuclei, the reticular nuclei, the red nuclei, and the substantia nigra.
Two cerebral peduncles in the ventral midbrain contain cortical fibers descending to the brainstem and spinal cord.
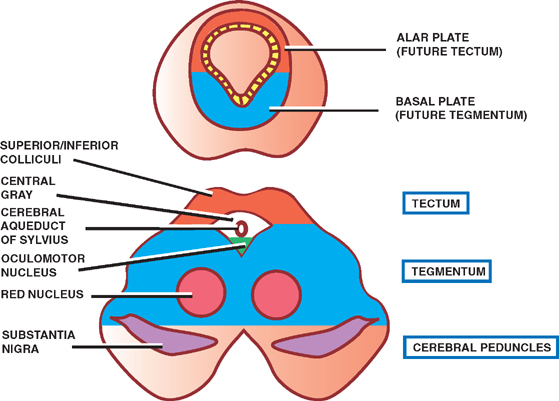
Fig. 1.10 The mesencephalon.
The Prosencephalon (Forebrain)
Early in development, a lateral outgrowth called the optic vesicle appears on each side of the forebrain. These vesicles, which give origin to the retinas and optic nerves, divide the forebrain into rostral and caudal parts, referred to as the telencephalon and diencephalon, respectively. (The optic vesicles themselves are of diencephalic origin.)
The Diencephalon
See Fig. 1.11.
The central cavity of the diencephalon becomes the third ventricle. As the third ventricle extends into the medial portion of the telencephalon, it is fanked by two larger lateral ventricles.
Three swellings develop in the lateral walls of the third ventricle that give rise to the epithalamus, the paired thalami, and the hypothalamus. The thalami are situated between the dorsally located epithalamus and the ven-trally located hypothalamus. They are separated from the epithalamus by the epithalamic sulcus, and from the hypothalamus by the hypothalamic sulcus.
The epithalamus gives origin to the habenular nuclei and the pineal gland.
The thalami, which expand greatly in the lateral walls of the third ventricle, reduce the ventricle to a thin slit. In many brains, the thalami meet and fuse in the midline to form a gray matter structure called the massa intermedia.
The hypothalamus contains several cell groups related to autonomic and endocrine functions, and a paired group of neurons called the mammillary bodies that are visible as rounded swellings on the diencephalon’s ventral surface.
More rostrally, two other swellings appear on the ventral surface of the diencephalon. These are the optic chiasm, in which the fibers from the medial halves of the retinas cross the midline, and the infundibulum, which is the stem of the pituitary gland. The retinas and the pituitary gland are both derived from a combination of surface and neural ectoderm, the latter of which is derived from a downward evagination of the diencephalon.
The Telencephalon
The telencephalon is subject to the most extensive developmental changes in the nervous system. It gives rise to the cerebral hemispheres, the cerebral commissures, the corpus striatum, and the internal capsule.
Early in development, the telencephalon consists of a median portion and two lateral diverticula, the tel-encephalic vesicles that will develop into the cerebral hemispheres. As mentioned earlier, the median portion of the telencephalon is filled by the rostral extension of the third ventricle. Filling the telencephalic vesicles are the lateral ventricles, which communicate with the third ventricle through the interventricular foramina (Monro).
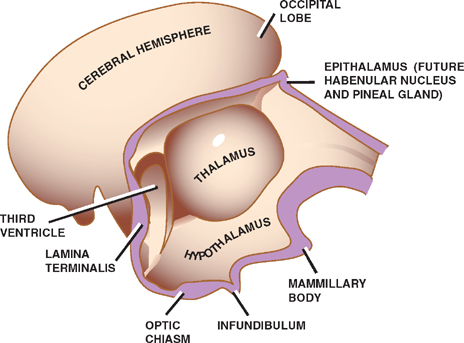
Fig. 1.11 The diencephalon.
Cerebral Hemispheres
See Fig. 1.12.
During the fifth gestational week, the developing cerebral hemispheres expand in several directions, overgrowing the diencephalon, the midbrain, and the hindbrain. Embryonic mesenchyme that is trapped in the longitudinal fissure between the two hemispheres gives rise to the falx cerebri.
The multiple directions through which the cerebral hemispheres expand account for its mature, C-shaped, confguration. Thus the frontal lobe is formed from anterior growth of the hemispheres; the parietal lobe from lateral-superior growth; and the occipital and temporal lobes from posterior-inferior growth. The slowly growing insula or insular cortex overlying the outer surface of the corpus striatum is overgrown by the frontal, parietal, and temporal lobes and thus comes to lie deep in the lateral cerebral sulcus (sylvian fissure).
A complex pattern of sulci and gyri develops in the external surface of the cerebral hemispheres, creating an increase in brain surface without a proportionate increase in the whole brain volume. Internally, the development of the lateral ventricles roughly parallels the development of the hemispheres. The anterior horn thus develops in the frontal lobe, the posterior horn forms into the occipital lobe, and the inferior horn projects to the temporal lobe.
The C-shaped pattern of growth assumed by the cerebral hemisphere and the lateral ventricle produces parallel C-shaped structures, including the fornix and the caudate nucleus (see later discussion).
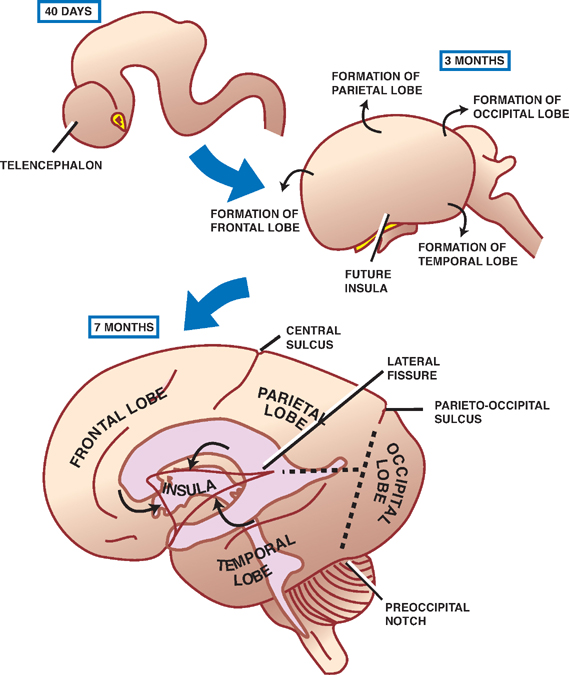
Fig. 1.12 Cerebral hemispheres.
Cerebral Commissures
See Fig. 1.13.
These are groups of fibers that interconnect corresponding regions of the two cerebral hemispheres. This function is originally served by the cephalic end of the neural tube, the lamina terminalis, which later forms the anterior wall of the third ventricle. Three major commissures develop within (or from) the lamina terminalis.
The anterior commissure is the first commissure to form. It connects the olfactory bulbs and temporal lobes of both sides. Forming a C-shaped arch that overlies the thalamus, the fornix, which develops next, consists of longitudinally oriented fibers that project from the hippocampus to the mammillary bodies of the hypothalamus. Commissural fibers of the fornix connect the hippocam-pal formations of both sides, forming the hippocampal commissure. Finally, the corpus callosum, the largest of the cerebral commissures, takes the form of an arch over the third ventricle. It connects the neocortices of both sides.
What is left of the lamina terminalis after the development of these commissures is a thin wall called the septum pellucidum, which separates the anterior horns of the lateral ventricles. The corpus callosum and the fornix bound the anterior horns of the lateral ventricles from above and below, respectively.
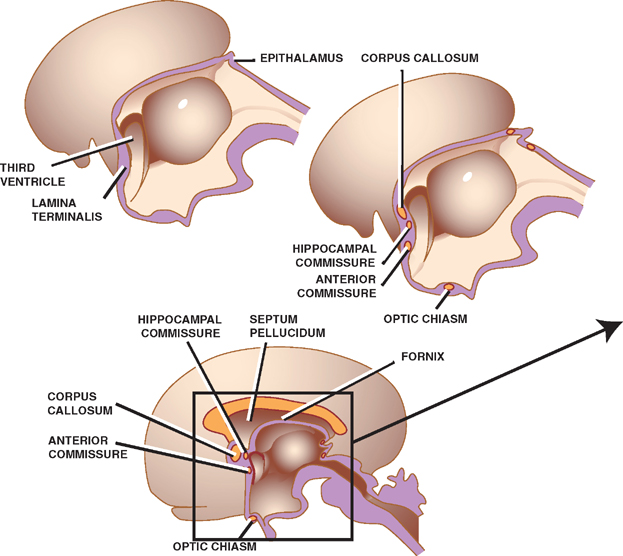
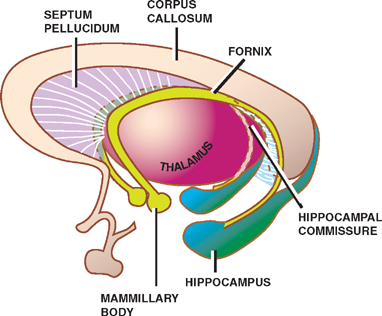
Fig. 1.13 Cerebral commissures.
Corpus Callosum
See Fig. 1.14.
Because of its clinical importance, the development of the corpus callosum is worthy of a more detailed discussion. Anatomically, the corpus callosum is divided into four sections: the rostrum, genu, body, and splenium. Development of the corpus callosum begins at about the seventh week of gestation, when the dorsal aspect of the lamina terminalis thickens into what is known as the commissural plate. Once formed, a groove develops in the commissural plate, which becomes filled with cellular material. This cellular material forms a glial bridge superiorly across the groove, the cellular components of which express surface molecules and secrete chemical messengers that attract and help guide axons across the midline to form the three cerebral commissures.
Development of the entire corpus callosum, however, does not occur simultaneously; rather, it follows a rostral to caudal sequence. This means that arrest of the corpus callosum development prior to its completion results in a normally formed anterior portion but an absent or only partially formed posterior portion. Exceptions to this rostral-caudal sequence of development include the ros-tralmost portions of the corpus callosum—the rostrum and the anterior part of the genu. Violations of the “front to back” rule may also occur as a result of secondary destructive processes that damage the corpus callosum after it has already fully formed. These processes may lead to an absent or small genu or body and an intact sple-nium and rostrum.
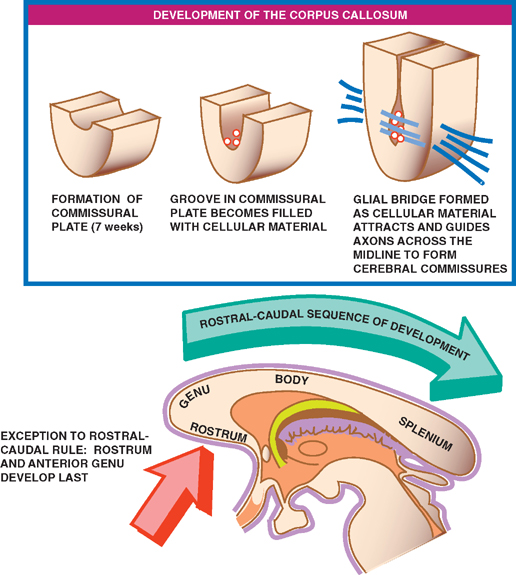
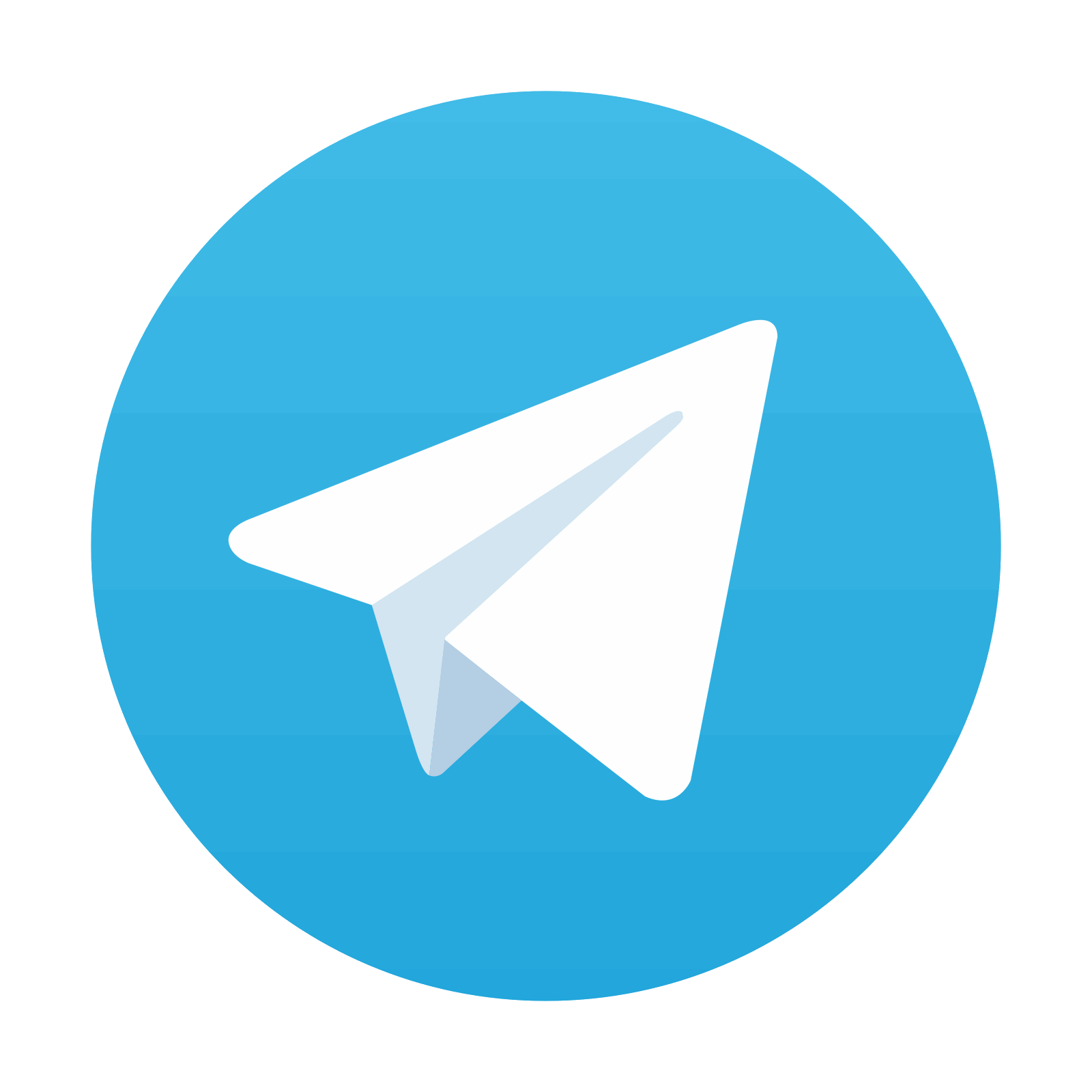
Stay updated, free articles. Join our Telegram channel

Full access? Get Clinical Tree
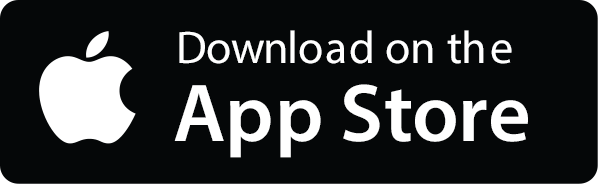
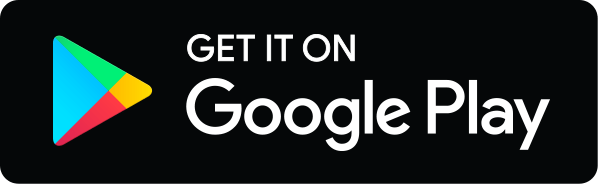