21 Acute Renal Injury in the Neurocritical Patient
Daniel Agustín Godoy 1, José Luis do Pico 2
1 Neurointensive Care Unit. Sanatorio Pasteur, Catamarca, Argentina
2 Head Intensive Care Unit. Hospital Municipal. Necochea. Buenos Aires, Argentina.
21.1 Introduction
Acute kidney injury (AKI), also known as acute renal failure, refers to a sudden decline in renal function, with disorders in fluid balance, electrolytes and acid base status due to loss of small solute clearance and decrease in the glomerular filtration rate (GFR). The term AKI reflects more accurately the spectrum of diseases from subclinical injury to complete organ failure.
21.2 Epidemiology of Acute Kidney Injury
The incidence of AKI has risen. Depending on how defined, between 35 and 66% of critically ill patients develop AKI, with a substantial increase in morbidity and mortality. On average, between 5 and 6% of critically ill patients with AKI in the critical care unit (CCU) require renal replacement therapy (RRT). The reported mortality due to AKI varies considerably, depending on the definition of AKI and the patient population evaluated (e.g., sepsis, trauma, cardiovascular surgery or contrast-induced nephropathy). Most studies found that mortality increases proportionally with increasing severity of AKI. In patients with severe AKI requiring RRT, mortality is about 50 to 70%. AKI requiring RRT is an independent factor of hospital mortality: even small changes in plasma creatinine (PCr) are associated with increased mortality.
The morbidity associated with AKI in the CCU is a less appreciated consequence, but it is associated with prolonged hospital stay, higher risk of chronic kidney disease (CKD) and end-stage renal disease (ESRD).
21.3 Definition of AKI in Critically Ill Patients
There are more than 35 definitions of AKI in the literature. In 2002, a group of experts formed the Acute Dialysis Quality Initiative (ADQI) and proposed the RIFLE classification scheme (R: risk, I: injury, F: failure, L: Loss, E: end-stage) specifically for AKI in critically ill or terminal patients (Table 21.1). Using the parameters of PCr and/or GFR and urine output, they defined three levels of severity and two prognostic classes.
Risk | One or more of the following:
|
Injury | One or more of the following:
|
Failure | One or more of the following:
|
Loss | Persistent AKI or complete loss of renal function: presence of AKI >4 weeks |
End | End-stage renal disease: requirement of renal replacement therapy >3 months |
Table 21.1. RIFLE criteria for AKI diagnosis and staging (2004).
Changes in serum creatinine as measured with the RIFLE method are applied in relation to a baseline serum creatinine value (BSCV). But since this is unknown in most patients, the modification of diet in renal disease (MDRD) formula (Table 21.2) was devised to estimate the BSCV, according to gender, race and age group, assuming a normal GFR (75 to 100 ml/min/1.73 m2).
GFR = 186 × SC-1.154 × Y-0.203 × 0.742° × 1.21*
186 × SC-1.154 = (Y-0.203 × 0.742° × 1.21*)/normal GFR
SC-1.154 = (Y-0.203 × 0.742° × 1.21* × 186)/normal GFR
where:
SC = serum creatinine; Y = years of age; ° in females; * in blacks
Age (years) | Black male (mg/dl) | White male (mg/dl) | Black female (mg/dl) | White female (mg/dl) |
20-24 | 1.5 | 1.3 | 1.2 | 1.0 |
25-29 | 1.5 | 1.2 | 1.1 | 1.0 |
30-39 | 1.4 | 1.2 | 1.1 | 0.9 |
40-54 | 1.3 | 1.1 | 1.0 | 0.9 |
55-65 | 1.3 | 1.1 | 1.0 | 0.8 |
≥65 | 1.2 | 1.0 | 0.9 | 0.8 |
Table 21.2. Estimated baseline creatinine value.
The RIFLE method’s validity and predictive ability for mortality have been evaluated in critically ill patients in several trials. In their review of studies which used this system between 2004 and 2007, Ricci et al. examined 24 studies involving a total of 71,000 patients. They noted that the studies had several weaknesses: small cohorts; selected subpopulations of critically ill patients; lack of “urine output” criterion; and single-centre design. What the studies did show, however, was the first mortality rates of AKI (31.2%) diagnosed according to a uniform definition. A striking finding was that the relative risk of death was higher when renal dysfunction was more severe (18.9%, 36.1% and 46.5% for mortality as risk, injury and failure, respectively).
In 2008, Bagshaw et al. published a multicentre evaluation of AKI diagnosed according to the RIFLE classification within the first 24 hours after admission (120,123 patients in 57 Australian CCUs from 2000 to 2005). They reported an AKI incidence of 36.1% (Risk = 16.2%, Injury = 13.6%, Failure = 6.3%), being more common in patients over 65 years of age, female gender, with comorbidities and admitted for medical causes, mainly cardiac, septic or liver diseases. Worsening in RIFLE category was correlated with an increased length of stay in hospital and the CCU and, as Ricci et al. had found, with higher mortality.
Recently, the ADQI group and representatives of the American Society of Nephrology (ASN), the International Society of Nephrology (ISN), the National Kidney Foundation (NKF) and the European Society of Intensive Care Medicine Acute Kidney Injury Network (AKIN) proposed a new diagnosis and classification system (Table 21.3) modified from the RIFLE scheme:
- The Risk, Injury and Failure categories were replaced with three numerical stages of severity (1, 2 and 3), while retaining similar cut-off points for PCr and urine output.
- The method’s sensitivity was enhanced by including in Stage 1 increases in PCr ≥0.3 mg/dl, based on studies showing that this small change was associated with higher risk of mortality.
- Implementation of RRT was eliminated as a severity criterion because patients classified in the Failure category who received RRT could be included in Stage 3, given that the starting time of RRT varies across different populations and countries due to variability of resources and criteria.
- An “acute” change in serum creatinine was specified as occuring within 48 hours, based on evidence that the worst prognosis observed with these small increases occurs during this period. Unlike RIFLE, the AKIN system considers increases in PCr in relation to the value recorded at admission rather than in relation to a theoretical baseline creatinine.
- It was noted that these criteria should be applied only after having achieved an optimal state of hydration because the blood volume status can modify PCr values.
AKIN stage 1 | One or more of the following:
|
AKIN stage 2 | One or more of the following:
|
AKIN stage 3** | One or more of the following:
|
Table 21.3. AKIN criteria for AKI diagnosis and staging (2007).
* “Acute” changes in serum creatinine refer to those which occur within 48 hours of the last recorded value
** Includes patients receiving RRT
In 2008, Bagshaw et al. carried out a retrospective comparison between the RIFLE and AKIN schemes on the same 120,123 patients evaluated the same year, and reported similar sensitivity, robustness and predictive ability of both methods. The study revealed several weaknesses, however: assessment of AKI was performed only within 24 hours of the patient’s admission to the CCU; detailed urine output and weight of patients were unknown; and there was no record of the proportion of cases undergoing RRT.
Except for the use of diuretics or the presence of urinary obstruction, diuresis is still considered a more sensitive parameter of intrarenal hemodynamic changes than biochemical markers or solute clearance. It is also more likely to be used in CCUs and general care wards owing to its easy identification.
The RIFLE system assumes that the use of this parameter enhances the method’s sensitivity. The AKIN scheme also recognizes that this marker of renal dysfunction becomes impaired before PCr values increase, thus offering a sensitive and easy tool to identify patients with AKI. Recently, Bagshaw et al. demonstrated that the use of urine output in the RIFLE scheme has prognostic and predictive value for length of CCU and hospital stay and for mortality.
Both the RIFLE and AKIN schemes set an arbitrary urine output value derived from the initial definitions of Kellum, which state that a patient with a urine output <800 ml in 24 hours can be defined as an AKI patient, or the equivalent of 30 ml/h, i.e., 0.5 ml/kg/h for a 70-kg patient. Although these figures are theoretical, neither the RIFLE nor the AKIN scheme specifies how often measurement should be taken, and it is unknown whether they are applicable to catheterized patients in whom measurements are taken every 2, 4 or even 6 hours, or in those with spontaneous urination.
21.4 Diagnosis of AKI in Critically Ill Patients
Procedures and parameters for diagnosing AKI (PCr) and determining its etiology (clinical history, physical examination, renal ultrasound, fractional excretion of sodium [FENa], fractional excretion of urea [FEU], plasma urea and urine sediment) are available in the CCU. However, no single marker may be accurate enough to estimate GFR and to indicate injury. A simple analogy of this problem is the use of cardiac enzymes (troponin specific to cardiac muscle) as a “surrogate marker” of cardiac muscle injury in acute myocardial infarction. This marker of cardiac injury does not provide information on changes in global cardiac function and myocardial performance. To evaluate cardiac performance, more examinations are needed. Extending this analogy to critical care nephrology, the surrogate markers for GFR (e.g., urea and creatinine), however, are not sufficiently specific for detecting acute kidney injury. What we urgently need are markers that incorporate function and injury in critical care nephrology.
The diagnosis and etiological classification of ARI depend largely on the detection of changes in endogenous conventional markers of renal function: serum levels of plasma creatinine and urea, and, less frequently, other urine tests. Although conventionally used, these markers have their limitations as none reflect dynamic changes in GFR nor do they reflect kidney injury. Moreover, these endogenous markers require time to accumulate before they can be detected as abnormal levels in serum, thus potentially delaying the diagnosis of AKI.
Serum creatinine is an amino acid compound derived from the non-enzymatic conversion of creatine in skeletal muscle and subsequent hepatic metabolism of creatine through the methylation of amino acetic acid to form creatinine. Creatinine clearance is the most common tool to estimate GFR, and serum creatinine levels typically show an inverse relationship to GFR (in stable situations). Like urea, there is no evidence of toxicity caused by creatinine accumulation in the blood. However, there are limitations in the use of serum creatinine as a marker of renal function:
- The production and release of creatinine in serum may be highly variable. Differences in age, gender, food intake (e.g., vegetarian or creatine supplements) and muscle mass (e.g., neuromuscular disease, malnutrition) can cause baseline serum creatinine values to vary significantly. Similarly, certain diseases can predispose to a variable release of muscle creatinine. For example, serum creatinine may rise more rapidly during rhabdomyolysis due to the release of preformed creatinine from damaged muscle or peripheral metabolism of creatine phosphate to creatinine in extracellular tissue. Critically ill patients may present abnormal liver function and a dramatic reduction in muscle mass, both of which can significantly alter creatinine metabolism. Other factors contributing to increased creatinine production include muscle catabolism in trauma patients, fever, and immobilization. Its production can be reduced in liver disease, in patients with reduced muscle mass and in the elderly. The volume of creatinine distribution (total body water) influences plasma creatinine and can increase dramatically in critically ill patients. However, like creatinine clearance, plasma creatinine does not accurately reflect GFR in non-equilibrium conditions such as AKI. Creatinine clearance overestimates GFR in patients with AKI because, as GFR decreases, tubular secretion of creatinine increases; therefore, urinary excretion is much greater than the filtered load, resulting in a potentially gross overestimation of GFR. During the evolution of AKI, plasma creatinine levels may underestimate the degree of dysfunction, while the opposite is true as far as kidney function will recover.
- About 10 to 40% of serum creatinine is cleared by tubular secretion into the urine. This effect has the potential to “hide” a substantial initial decline in GFR.
- Many drugs (e.g., trimethoprim, cimetidine) may alter creatinine secretion, leading to transient and reversible increases in serum creatinine levels.
- Although less frequent, the accuracy of serum creatinine assays may be reduced, leading to artificial increases in serum creatinine levels in diabetic ketoacidosis; increased serum concentration of acetoacetate may interfere with selected tests (e.g., with the alkaline picrate method) and cause false elevations in serum creatinine regarding Jaffe’s reaction. Similarly, some drugs may have similar effects (e.g., cefoxitin).
- Various equations to evaluate kidney function can be applied to predict creatinine clearance or GFR. They are based on demographics and biochemical indices (e.g., gender, age, race, weight, blood creatinine and albumin) but they have not been validated in patients with AKI. All equations use serum creatinine and, therefore, have the same limitations as serum creatinine for the assessment of GFR in patients with AKI. There are few data on the use of equations to estimate GFR in patients with AKI.
Changes in serum creatinine are aspecific, do not discriminate between the nature and the type of renal insult (ischemic, nephrotoxic) or the site and extent of tubular or glomerular injury, and serum creatinine levels are relatively insensitive to small changes in GFR. Moreover, changes in serum creatinine “may fall behind” the changes in GFR, both its increase and its decline, for several days. Finally, because serum creatinine is influenced by potential interventions in AKI (creatinine removed by RRT), its specificity to assess renal recovery is even more problematic.
Plasma urea, a by-product derived from the metabolism of water soluble low-molecular-weight proteins is used as serum marker of retention and removal of nitrogenous solutes. The accumulation of urea per se is thought to predispose to metabolic biochemical and physiological side effects such as increased oxidative stress and impaired function of Na-K-Cl co-transporters, which are critical in regulating intracellular water and potassium, and alterations in immune function. In addition, retention of uremic toxins may contribute to such secondary organ dysfunctions as acute lung injury.
Similarly to serum creatinine, urea levels exhibit a non-linear and inverse relationship to GFR. However, measuring urea levels to estimate GFR is a problem due to extra-renal factors which influence its endogenous production and renal clearance, regardless of the GFR.
The urea production rate is not constant. Urea values can be modified by high protein intake, critical illness (sepsis, burns, trauma), gastrointestinal bleeding, or drugs such as steroids or tetracycline. The use of steroids in the CCU is a fairly common practice and may increase protein catabolism. Critically ill patients with chronic liver disease may have almost normal urea levels due to reduced protein production and restriction and, at the same time, normal serum creatinine levels due to either decreased hepatic production of creatinine or loss of muscle mass, despite significant reductions in GFR and impaired renal function.
The rate of renal clearance of urea is not constant. Approximately 40-50% of filtered urea is passively reabsorbed by the proximal and distal tubule cells. In states of volume depletion with low cardiac output, there is an increased reabsorption of sodium and water in the proximal tubule cells, with a corresponding increase in urea reabsorption. The urea concentration can increase far beyond the rate of change in serum creatinine and doesn’t represent a reduction in GFR.
Overall, urea concentration is a poor marker of GFR. It doesn’t represent real-time changes in GFR and requires time to accumulate. In addition, urea doesn’t really reflect “acute” kidney injury. Relying on serum levels of urea leads to delays in the diagnosis of AKI.
Urine output can be used as a “crude” dynamic measurement of renal function. It may be a more sensitive marker of changes in renal hemodynamics than biochemical markers for the clearance of solutes. The importance of dynamic changes in urine output has been recognized because such changes are integrated into the RIFLE and AKIN classifications. Generally, however, urine output lacks sensitivity and specificity as a marker of renal function or AKI in critically ill patients in whom the excretion of free water and solutes is altered. At times, critically ill patients with severe AKI, characterized by elevated serum creatinine or retention of nitrogenous solutes, can maintain normal or even high urine output.
The term AKI spans the whole spectrum from prerenal azotemia to acute tubular necrosis (ATN), and from functional impairment to structural injury. Numerous tests have been described as a “replacement” for tubule cell function and have been and still are used for the detection and classification of AKI, particularly in the diagnosis of prerenal azotemia and ATN. Although easy to perform, they lack sensitivity and specificity for the early characterization of AKI, particularly in critically ill patients. In addition, the causes of prerenal azotemia and structural injury commonly coexist in critically ill patients. Until we have other diagnostic tools, different from the current ones, and in the absence of validated new biomarkers, prerenal azotemia is often a retrospective diagnosis made only after fluids challenge.
Fractional excretion of sodium (FENa) is based on the fact that filtered sodium is avidly reabsorbed in the renal tubules of the glomerular filtrate in prerenal azotemia, in which tubular function remains intact, resulting in FENa <1%, while FENa is >1% in tubular injury, as in ATN. Although physiologically sensitive, in clinical practice the diagnostic accuracy of FENa is poor and its value has been questioned.
FENa is often >1% in patients receiving diuretics and has been found to be <1% in various settings, including sepsis, rhabdomyolysis, and exposure to contrast media. Carvounis et al. suggested that the fractional excretion of urea (FEU) is more sensitive and specific for discriminating between prerenal azotemia and ATN, especially if diuretics have been administrated. However, this study had important methodological errors.
Not all tests have been tested and questions remain whether they are useful for the diagnosis and classification of AKI in critically ill patients. Also, they don’t quantitatively measure kidney injury nor do they provide any information about prognosis. We must remember that the classification of AKI in prerenal azotemia and ATN is arbitrary and that both probably exist as a continuum of injury and that their separation in terms of diagnosis also has its clinical and prognostic limitations.
Proteinuria is frequently detected in critically ill patients. Microalbuminuria is defined as <300 mg/g of creatinine or proteinuria >300 mg/g of creatinine. Proteinuria is more commonly encountered in the elderly, patients with diabetes, chronic kidney disease, or shock. A high albumin/creatinine ratio (>100 mg/g) is associated with increased mortality.
The detection of microalbuminuria is suggestive of increased capillary permeability to proteins, with a predictive and prognostic value of disease severity and mortality. However, no studies have assessed the value of microalbuminuria in predicting the course of AKI in patients admitted to the CCU. The detection of low-molecular-weight proteins of tubular origin has been described in critically ill patients with AKI and sepsis. No study has evaluated a priori urinary protein excretion as a marker of AKI or as a predictor of decline in renal function or recovery in critically ill patients.
The classic urinary profile of ATN is represented by tubular epithelial cells with large casts, brown casts or casts with mixed cells, whereas fine granular casts can sometimes be detected in prerenal azotemia.
Few studies have assessed urinary sediment in AKI in critically ill patients. Moreover, the value of urine sediment to classify AKI pathogenesis and severity is imperfect and often fails to correlate with traditional urinary biochemical markers or derived indices. There are no clinical studies to date that have evaluated a priori the value of urine sediment and microscopy as a marker of renal function or AKI. Urine microscopy should be performed when systemic vasculitis, rapidly progressive glomerulonephritis or a pulmonary-renal syndrome is suspected.
In conclusion, these conventional markers are familiar to the CCU team though they are not ideal, have limitations, and none reflect real-time changes in GFR or genuine injurious processes in the kidney. Moreover, they may lead to delayed recognition of AKI and delay proper support and therapeutic interventions.
Fortunately, the application of innovative technologies such as functional genomics and proteomics in humans and animal models of AKI has discovered new genes and gene products that have emerged as biomarkers. Biomarkers are required to:
- Identify the primary site of injury (proximal tubule, distal tubule, interstitium, or vasculature).
- Indicate the duration of renal failure (AKI, chronic renal disease or re-exacerbation of chronic renal failure).
- Identify the pathogenesis of AKI (ischemia, toxins, sepsis, or a combination thereof).
- Stage risk and prognosis (AKI duration and severity, need for renal replacement, hospital stay, mortality).
- Define the course of AKI.
- Monitor the response of AKI to various interventions.
New and promising markers of AKI have been proposed and are currently under evaluation. These include a plasma panel (neutrophil gelatinase-associated lipocalin [NGAL] and cystatin C) and a urine panel (NGAL, IL-18 and KIM-1). Because they are sequential biomarkers, it is likely that panels for the diagnosis of AKI may be useful at the time of initial insult and to assess the duration of AKI (analogous to the diagnostic panel in chest pain) and to predict global prognosis regarding RRT and mortality. What is likely is that diagnostic panels will allow us to distinguish between different types and pathogenesis of AKI.
21.5 Common Causes of AKI in Critically Ill Patients
The causes of AKI in critically ill patients are multifactorial and often result from a combination of hypovolemia, sepsis, pharmacological therapy, and hemodynamic alterations. Often, a single cause cannot be isolated, further complicating the search for effective therapeutic interventions in this complex disease.
Sepsis is the most common cause of AKI in critically ill patients, accounting for up to 50% of cases. AKI is common after cardiac surgery, occurring in up to 40% of patients without pre-existing renal disease and associated with increased morbidity and mortality with an elevated PCr of only 0.3 mg%. AKI in trauma patients is multifactorial (e.g., hemorrhagic shock, abdominal compartment syndrome, rhabdomyolysis) and occurs in up to 31% of adult trauma patients.
The kidneys are very sensitive to intra-abdominal hypertension and intra-abdominal pressure over 12 mmHg associated with AKI. A sustained intra-abdominal pressure of 20 mmHg in association with a new organ dysfunction may be associated with AKI in more than 30% of cases. Rhabdomyolysis occurs in 28% of trauma patients with AKI requiring RRT.
Pharmacological therapy is a common cause of AKI, accounting for about 20% of all causes of AKI in critically ill patients. The mechanisms inducing AKI are multiple, including acute interstitial nephritis (AIN), direct tubular toxicity (e.g., aminoglycosides) and hemodynamic abnormalities (e.g., nonsteroidal anti-inflammatory drugs (NSAIDs), angiotensin-converting enzyme (ACE) inhibitors). AIN is an underrecognized cause of AKI in the CCU and is associated with pharmacological therapy. Because of the relative paucity of clinical findings, however, a high index of suspicionis needed for diagnosis.
21.6 Prevention and Management of AKI in Critically Ill Patients
The primary prevention of AKI in the CCU is limited to those conditions in which the time of injury is foreseeable, such as exposure to contrast media, cardiac surgery, and chemotherapy. Unlike most cases of community-acquired AKI, almost all cases of AKI in critically ill patients originate from more than one insult (Tables 21.4 and 21.5). In critically ill patients the first insult is often unpredictable. Therefore, prevention of AKI in the CCU relies on the prevention of secondary injury in a patient at high risk of AKI, as the patient is critical. In a retrospective study of over 5000 critically ill patients (6), AKI developed in 67% and occurred after CCU admission in 45%.
The general principles of secondary prevention of AKI include:
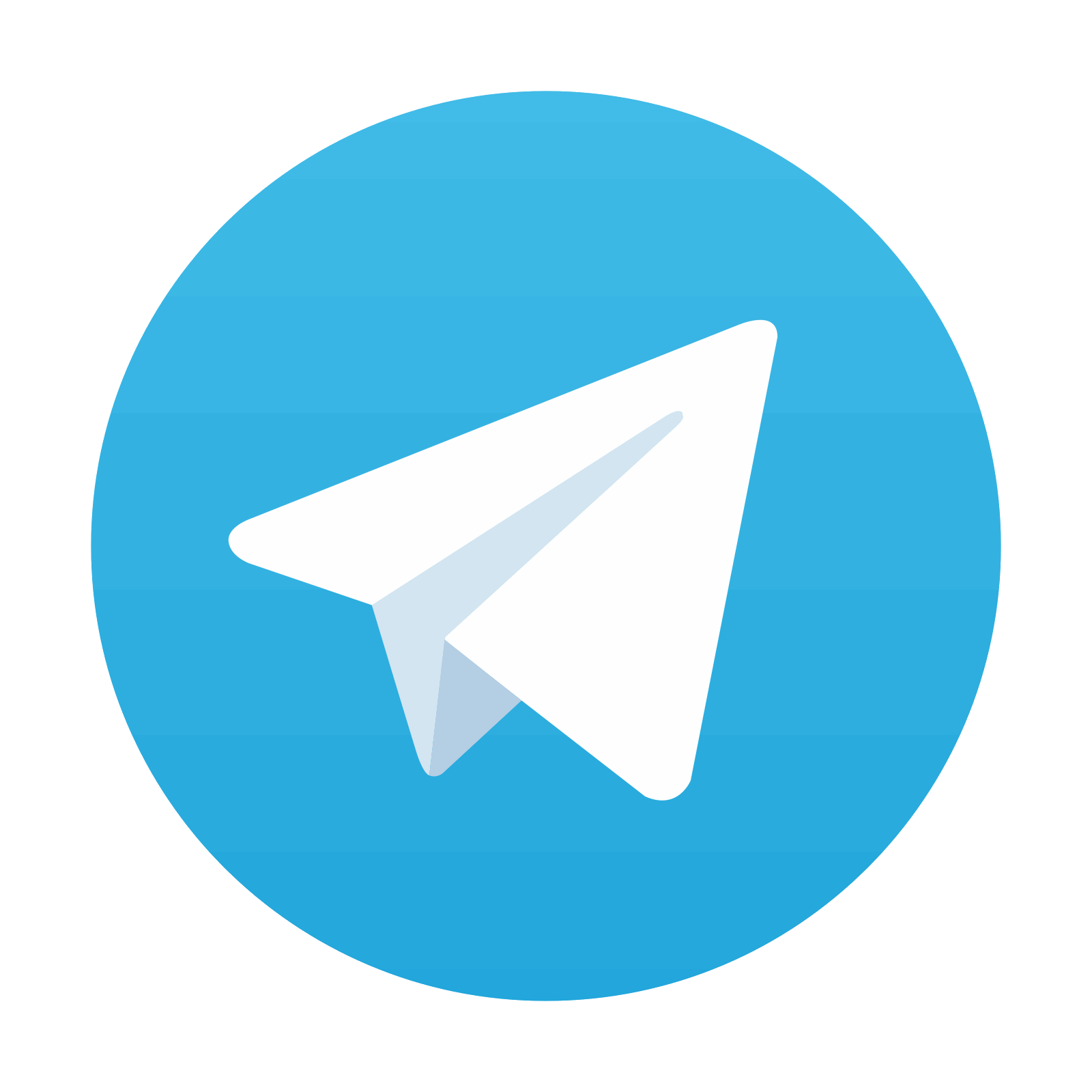
Stay updated, free articles. Join our Telegram channel

Full access? Get Clinical Tree
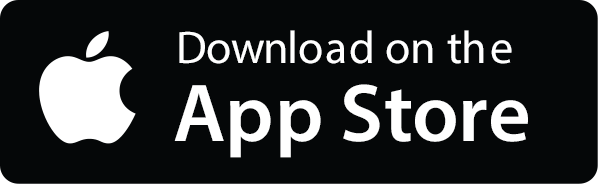
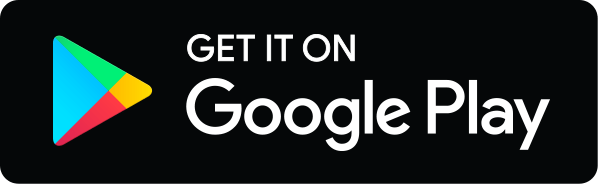