39 Acute Spinal Cord Injury: Pathophysiology and Intensive Care Management
David W. Cadotte 1, Doron Rabin 1, Michael G. Fehlings 1
1 Krembil Neuroscience Centre Spinal Program, Toronto Western Hospital; and Department of Surgery, Division of Neurosurgery, Toronto, Ontario, Canada
39.1 Introduction
In this chapter, we lay the scientific foundation for managing acute spinal cord injury by discussing the definitions of primary and secondary spinal cord injury (SCI). Understanding these concepts is important to implementing effective strategies aimed at reducing long-term neurological deficits. Afterwards, we review the important distinction between spinal and neurogenic shock and outline management strategies for patients suffering from diminished autonomic function. We describe the various spinal cord syndromes that will help clinicians correlate neurological findings to specific areas of pathology in the spinal cord. Lastly, we will cover specific patient management practices and clinical concepts essential to the care of patients during the acute stages of SCI and for optimizing neurological outcome.
39.2 Pathophysiology of Spinal Cord Injury
Acute SCI can be considered in terms of the primary injury, usually as a result of trauma or assault, and the biological mechanisms subsequent to this event, termed secondary injury. It is of critical importance to be aware of these secondary mechanisms because most of the intensive care and surgical strategies performed immediately after the injury aim to limit this damage in the attempt to improve the chances of functional neurological recovery.
Primary SCI refers to the damage inflicted on the neural elements as a result of an acute traumatic event. This can take the form of shear forces disrupting white mater tracts or hemodynamic compromise from blood vessel disruption. This damage occurs at the time of the injury and is, to date, irreversible. Secondary SCI is a sequence of hemodynamic and biochemical events that occur in response to the initial injury. While a detailed review of these mechanisms is beyond the scope of this chapter, we will highlight a few of the essential mechanisms to offer an introductory understanding. Secondary injury can take the form of systemic hypotension or hypoxia resulting in focal ischemia around the area of injury. Another form of secondary injury occurs at the cellular level and can take the form of ion-mediated cellular damage, excitotoxicity, apoptosis, oxidative cell damage, mitochondrial dysfunction, neuroinflamation or vasospasm. Each of these secondary mechanisms can theoretically be mitigated with various medical or surgical strategies. Such strategies will be outlined in the second and third part of this chapter. A summary of primary and secondary mechanisms of SCI are summarized in Table 39.1.
Primary mechanisms of injury |
|
Secondary mechanisms of injury | A complex cascade of events that invariably follows primary injury:
|
Table 39.1. Primary and secondary mechanisms of spinal cord injury.
In managing a patient with a SCI the treating team must adhere to Advanced Trauma Life-support (ATLS) Guidelines. The neurological examination should be done following stabilization of the airway, breathing, circulation and management of any other immediately life-threatening situation. Following this, a neurological examination should be carried out with the following two concepts in mind: spinal shock and neurogenic shock.
39.2.1 Spinal Shock
Spinal shock refers to depressed spinal reflexes caudal to the injury site following SCI. This is an important concept to understand as the initial neurological examination may not be an accurate reflection of disrupted neuronal circuits, including those that control motor and sensory pathways. Normally, these reflex pathways receive continuous input from the brain. When this tonic input is disrupted, the normal reflex pattern is disrupted and can vary from areflexic through to hyperreflexic depending on the time since the original injury.
Ditunno recently proposed a four-phase model to explain the clinical observations that occur caudal to the site of injury [1]. Briefly, these phases are as follows: Phase 1 (from the time of the injury to approximately 24 hours later) is characterized by hypo- or areflexia, which ensues from disrupted descending modulation. Phase 2 (between 24 and 72 hours following injury) is characterized by gradual return of the reflex. This is thought to result from neurotransmitter receptor up-regulation or denervation supersensitivity. Phase 3 (1-4 weeks following injury) is clinically characterized by hyperreflexia, which is the result of axon-supported synapse growth. Phase 4 (1-12 months after injury) is also characterized by hyperreflexia and results from cell-body-supported axon growth. The important point to gain from this discussion is that descending modulation can be suspended in the phase immediately following SCI. Clinically, this translates into the potential for a misleading representation of deficits if spinal reflexes are absent following injury. It is therefore recommended that patients not only be examined on presentation to the treating physician but also at the 72-hour mark following injury.
39.2.2 Neurogenic Shock
Neurogenic shock is a potentially life-threatening condition and must be managed as such. Without a clear understanding of this condition, inappropriate management of a trauma patient, who often suffers concomitant hemodynamic instability, could be fatal. Neurogenic shock is defined as a disruption of the sympathetic nervous system with preserved parasympathetic activity. This typically occurs in patients suffering a severe SCI at the level of T6 or higher. A comprehensive, open-access review was recently published in 2008 [2] and the reader is referred to this for a detailed understanding. The salient clinical aspects are reviewed here. Disruption of the sympathetic division of the autonomic nervous system has three effects on the cardiovascular system: coronary blood flow, cardiac contractility and heart rate [3]. With preserved parasympathetic activity this translates clinically into bradycardia (and possibly other cardiac arrhythmias) in a setting of profound hypotension [4]. A prudent clinician must look for these characteristics in combination, as many trauma patients are hypotensive as a result of blood loss or intravascular hypovolemia but will mount an appropriate tachycardia response.
The treatment of neurogenic shock is therefore quite difficult. While it is theoretically possible to distinguish between hypovolemic and neurogenic shock, this is not so clinically. In fact, acute trauma patients sustaining a high cervical SCI may suffer from both conditions. It has therefore been recommended by the Consortium for Spinal Cord Medicine to rule out other causes of shock before assuming a diagnosis of neurogenic shock [5]. The practical treatment of these patients rests on initially restoring intravascular volume and if symptoms of neurogenic shock persist, vasopressors (such as dopamine) should be used. The goal of treatment in the first week after sustaining a SCI is to maintain a mean arterial blood pressure of 85 mmHg [6,7].
39.2.3 Spinal Cord Syndromes
A detailed and consistent neurological exam is essential to the management of the patient with acute SCI. We recommend the use of the American Spinal Injury Association and International Spinal Cord Injury Society (ASIA-ISCoS) scale for characterizing the severity of injury [8]. In brief, an ASIA A grade refers to a complete injury with no sensory or motor function present from the level of injury to the sacrum. An ASIA B grade is assigned to a patient with some preserved sensation but no motor function from the level of injury to the sacrum. ASIA C and D grades are differentiated by the absence or presence of grade 3 power (full motion against gravity), respectively. ASIA E is reserved for acute SCI patients who are neurologically intact. A form illustrating how the neurological examination is recorded according to the ASIA-ISCoS standards can be obtained for free from the ASIA website (http://www.asia-spinalinjury.org).
Neurological examination of the patient should be performed immediately following the injury and ideally within 72 hours, especially if spinal shock is suspected. One should be aware of the terminology used to describe deficits and different SCI syndromes. Paresis refers to weakness or partial paralysis. For example, hemiparesis describes weakness on one side of the body. In contrast, both paralysis and the suffix plegia refer to no movement. For example, hemiplegia refers to no movement on one side of the body. Keeping these terms in mind, we turn our attention to spinal cord syndromes that occur when a select region of the spinal cord is damaged, resulting in a predictable pattern of neurological deficit. These syndromes are outlined in Table 39.1 and expanded on in the paragraphs that follow.
Transverse spinal cord lesions disrupt all motor and sensory pathways at and below the level of the lesion. There is a sensory level that corresponds to the level of the lesion. Common causes of this syndrome include trauma or more rarely spinal cord tumours.
Hemi-section of the spinal cord, commonly referred to as the Brown-Sequard syndrome, is characterized by damage to one half of the spinal cord and all motor and sensory pathways at that level and neurological deficits at and below that level. This results in ipsilateral upper-motor neuron weakness and ipsilateral loss of vibration and position sense at and below the level of lesion. There is contralateral loss of pain and temperature sensation below the level of the lesion. There may also be ipsilateral loss of pain and temperature at the level of the lesion for one or two spinal segments if the lesion has damaged posterior horn cells before the fibres have crossed to the other side. Common causes of this type of injury include penetrating trauma or a tumour compressing only one side of the spinal cord.
Central cord syndrome is commonly caused by a traumatic spinal cord contusion, posttraumatic syringomyelia or a medullary spinal tumour. This lesion tends to affect pathways that are in the immediate vicinity of the central portion of the spinal cord and the symptoms differ depending on the size of the lesion. Small lesions affect the spinothalamic fibres that cross in the ventral commissure and cause bilateral regions of suspended sensory loss to pain and temperature. If the lesion is larger, anterior horn cells, corticospinal tracts and posterior columns may be affected. Loss of these pathways respectively results in lower-motor neuron deficits at the level of the lesion, upper-motor neuron signs below the level of the lesion, and loss of vibration and position sense below the level of the lesion. In addition to suspended pain and temperature loss with small lesions, larger lesions can result in complete loss of pain and temperature below the level of the lesion if the anterolateral pathways are compressed from a medial aspect. Given the spinal cord laminations, sacral sparring is observed.
Posterior cord syndrome involves bilateral loss of vibration and position sense below the level of the lesion as a result of disruption of the posterior columns. If the lesion is large enough, one may observe upper-motor neuron signs below the level of the lesion indicating involvement of the lateral corticospinal tracts. Common causes include trauma or a posterior located tumour. In addition, vitamin B12 deficiency or tertiary syphilis can cause isolated involvement of the posterior columns.
Anterior cord syndrome results in loss of pain and temperature sensation below the level of the lesion (spinothalamic pathways), lower-motor neuron signs at the level of the lesion (anterior horn cell damage) and upper-motor neuron signs below the level of the lesion (lateral corticospinal tracts). In addition, urinary incontinence is common because of the ventral location of the descending pathways controlling sphincter function. Common causes of this syndrome include trauma and anterior spinal artery infarct.
39.3 Acute Management of the Patient With Spinal Cord Injury
The ATLS guidelines emphasize the need to establish and stabilize airway, breathing and circulation in trauma patients. These important steps in resuscitation precede neurological examination or imaging of the neuraxis. Spinal precautions should be implemented throughout resuscitation, with special care to avoid manipulation of the cervical spine during intubation. Major life-threatening thoracic, abdominal or extremity injuries may be masked by SCI. Continuous cycling through primary and secondary trauma surveys should minimize the risk of missing such injuries or changes in neurological function.
39.3.1 Hemodynamic Support and Oxygenation
Serial neurological examination is paramount to detecting any deterioration in function quickly and preventing permanent deficit. Accurate neurological examination may not be possible in a patient who is sedated or under general anesthetic. Furthermore, the pharmacological agents administered may produce hypotension and small vessel vasoconstriction, which can induce further ischemic damage to the injured spinal cord. The period of induction during administration of a general anesthetic may be a period of relative hypoperfusion secondary to hypotension. Under these circumstances, all members of the health care team must be vigilant in maintaining the hemodynamic parameters outlined in the next paragraph.
As stated in the first part of this chapter, a mean arterial blood pressure of 85 mmHg should be maintained during the first week following SCI [6,7]. Vale et al. employed arterial lines and Swan-Ganz central catheters to monitor patient hemodynamics. Volume resuscitation with crystalloid and blood products were initially used to maintain a mean arterial blood pressure of 85 mmHg. If required, a dopamine (2.5-5 µg/kg/min) infusion was started to meet this target. Mean arterial pressure was further supplemented with levophed (0.01-0.2 µg/kg/min) when needed. When administering vasopressors to the patient with SCI, it is important to avoid agents that would produce vasoconstriction of the small vessels supplying the spinal cord. Aggressive management of mean arterial pressure and oxygenation are one the most important aspects of acute care in the patient with SCI. Similarly, intraoperative blood loss should be closely monitored. Vale et al. administered blood products to keep hematocrit >32%. We often administer blood products early, as opposed to waiting for hemoglobin/hematocrit to reach critical levels [7].
39.3.2 Neuroprotection
Inducing regional or systemic hypothermia for acute SCI aims to minimize secondary injury by lowering enzymatic activity and energy requirements of the injured spinal cord. Many of the studies examining the impact of hypothermia on acute SCI reported the experience in small groups of patients with heterogeneous clinical characteristics. The results were further confounded by the administration of multiple neuroprotective measures simultaneously. Kwon et al. [9] reviewed the relevant literature in 2008 and concluded that there is no compelling evidence that hypothermia (systemic or regional) improves neurological outcome in acute SCI. Furthermore, systemic hypothermia carries an increased risk of bacteremia/infection, coagulopathy, raised intracranial pressure and cardiac arrhythmia. While a randomized control trial to examine the utility of hypothermia in SCI is under development, we do not recommend inducing hypothermia for neuroprotection in acute SCI.
The administration of pharmacological agents to mitigate the effects of primary and secondary mechanisms of acute SCI remains controversial. Methylprednisolone, GM-1 ganglioside, thyrotropin-releasing hormone, nimodipine and gacyclidine have been tested in prospective randomized control trials of acute SCI in humans [10,11]. Only methylprednisolone and GM-1 ganglioside have shown modest benefits in neurological outcome [10]. For the purposes of this text, we will focus on the protocol for administering methylprednisolone in acute SCI.
The National Acute Spinal Cord Injury study (NASCIS I) randomized SCI patients into two cohorts; a 100 mg loading dose of methylprednisolone followed by 25 mg every 6 hours for 10 days in one group, and a 1000 mg loading dose of methylprednisolone followed by 250 mg every 6 hours for 10 days in a second group [12]. There were no differences in neurological outcome between the two groups at 1 year after injury [12]. NASCIS II investigated a 30 mg/kg bolus of methylprednisolone over 1 hour followed by 5.4 mg/kg/h over the following 23 hours and included a placebo group and naloxone administration group [13]. The results from the overall group showed no significant differences in neurological outcome at 6 months, though subgroup analysis demonstrated improved motor and sensory outcomes in the patients receiving methyprednisolone within 8 hours of injury [13]. NASCIS III examined outcomes in acute SCI patients receiving 30 mg/kg bolus of methypredniosolone followed by 5.4 mg/kg/h for either 23 hours or 47 hours [14]. Patients could be randomized into a third group receiving tirilazad mesylate [14]. The patients treated with methylprednisolone for 48 hours had better neurological outcomes in comparison to the other treatment groups if therapy was initiated within 3-8 hours of injury [14]. The 48-hour regimen was associated with an increased risk of sepsis and pneumonia [14]. Bracken reviewed five studies (including NASCIS I, II and III) investigating methyprednisolone in acute SCI and concluded that high-dose therapy was safe and modestly effective in improving neurological outcome [15].
There is still considerable debate as to whether or not high-dose methylprednisolone should be administered in the context of acute SCI. This has led to variability in the decision to administer methylprednisolone and specific protocols [16]. The senior author of this chapter has published his protocol for administering methylprednisolone following acute SCI based on the timing of administration [10]. Patients with acute non-penetrating SCI should receive methyprednisolone as per the NASCIS II protocol if started less than 3 hours after injury. If started between 3 and 8 hours after injury, then the NASCIS III 48-hour protocol should be applied. If therapy cannot be administered within 8 hours of injury, or there is a penetrating SCI, then methylprednisolone should not be administered for neuroprotection. The decision to administer methyprednisolone must account for medical comorbidities. For example, the risks of administration may outweigh the benefits in a patient with diabetes mellitus and a complete thoracic SCI injury. Blood glucose levels must be carefully monitored during the course of methylprednisolone therapy, and hyperglycemia aggressively managed with an insulin infusion.
39.3.3 Surgical Management of Acute Spinal Cord Injury
Prior to discussing the surgical management of SCI, it is necessary to outline appropriate imaging used to identify the extent and type of bony and soft tissue injuries. Selecting the anatomic spinal area to be imaged depends on the neurological examination. A quadraplegic patient requires imaging of cervical spine (skull base to top of T1), while a paraplegic patient with a thoracic sensory level requires a radiograph of the entire thoracic spine. While plain radiographs may be an appropriate first step, computed tomography (CT) scan is essential for characterizing bony pathology, while magnetic resonance imaging (MRI) is used to identify soft tissue injury, including injury to the spinal cord. The clinician should be aware that a significant proportion of SCI patients have more than one anatomic level of spinal injury, which may or may not be contiguous. Appropriate imaging is essential to planning further surgical management.
Closed reduction aims to relieve compression of the spinal cord by restoring normal bony alignment. The technique involves applying traction force to the skull through rigid fixation with Gardner-Wells tongs or Halo, and reducing a cervical dislocation. Increasing weight is added over time while neurological status and radiographic monitoring of the cervical fracture dislocation are assessed between traction increments. Traction may be an appropriate first step in a conscious patient who can undergo reliable serial neurological assessment, especially in the setting of bilateral locked facets [17]. This technique does not address compression secondary to hematoma or soft tissues. It may be impossible to obtain and/or maintain bony reduction with this technique. Surgical decompression and reduction offer the advantage of addressing all compressive etiologies immediately and providing the opportunity for internal fixation. Attempts at closed reduction should not delay definitive surgical decompression, open reduction and internal fixation of the spine.
Evolving evidence suggests that early decompression may enhance recovery after cervical SCI and has acceptable safety, though this remains somewhat controversial. The guidelines for the management of acute cervical spine injuries published in 2002 include early open or closed reduction of cervical fractures as a management option for SCI [18]. However, in actual practice, there appears to be considerable heterogeneity in the timing of surgery for acute SCI [19]. This variability in surgical timing may be due, in part, to a lack of a clear definition of “early surgery” or other factors, including logistical issues such as delays in medical transfers and the availability of key imaging or surgical resources.
Recent systematic reviews of the literature suggest that there is a biological basis for surgical decompression of the spinal cord within 24 hours of injury [10,19-21]. Preliminary results from the Surgical Treatment of Acute Spinal Cord Injuries Trial (STASCIS) indicate that decompression within 24 hours of injury may actually improve outcome in patients with isolated SCI [22]. Early decompression (within 24 hours) should be considered as part of the therapeutic management of any patient with SCI, particularly those with cervical SCI. Very early decompression (within 12 hours) should be considered for a patient with an incomplete cervical SCI (with the possible exception of the central cord syndrome). An international survey [23] indicates that the majority of spine surgeons prefer to decompress the acutely injured spinal cord within 24 hours. The majority of spine surgeons prefer to decompress the cervical spine for patients with complete or incomplete cervical spinal cord injury within 24 hours.
39.3.4 Pressure Ulcers
Preventing pressure ulcers is a life-long concern in SCI patients. The risk of developing a pressure ulcer is stable over the first 10 years after injury, with a reported prevalence of 11.5% within the first year of injury and 14.3% within 10 years of injury [24]. Preventing pressure ulcer following acute SCI may initially be overshadowed by issues of transportation, resuscitation, decompression and stabilization.
A recent systematic review of the literature identified a number of possible risk factors for the development of pressure ulcer in the acute SCI period (defined as prior to admission to a rehabilitation facility) [25]. Mobility level, activity level, lung disease, decreased mental status and nutritional status were identified as risk factors for the development of pressure ulcer, though the evidence was considered insufficient based on conflicting evidence between studies included in the review [25]. Low blood pressure was considered a possible risk factor with moderate evidence, as were transport time to hospital and time on a spine board [25]. Length of stay in hospital, tracheostomy and vertebral osteomyelitis were reported as possible risk factors with insufficient evidence[25]. Based on these results, both patient factors and medical management factors seem to play a role in the development of pressure ulcer.
There are no recognized guidelines for pressure ulcer prophylaxis in the acute phase of SCI. We would recommend removing the patient from the spine board upon arrival to hospital and optimizing oxygenation, hemodynamics and nutrition. These measures are supported by other authors [26]. A high level of clinical suspicion and vigilance, with frequent inspection of anatomical pressure points are required to provide adequate protection and early detection of pressure ulcer.
Results of a recent multicenter, international, prospective cohort study (STASCIS) indicate that not only is early surgery safe and feasible but results in better neurological outcomes at 6-months follow up relative to SCI patients who undergo surgical decompression beyond 24 hours. This trial defined early surgery as <24 hours and the mean time to surgery of the early group was 14.2 +/- 5.4 hrs. Late surgery was defined as occurring beyond 24 hours and in this study had a mean time 48.3 hr [27].
39.3.5 Venous Thromboembolism
Venous thromboembolism (VTE) is common in the SCI population. A recent review indicated that clinically symptomatic deep venous thrombosis (DVT) and pulmonary embolism (PE) occur in 12 to 64% of SCI patients not treated with thromboprophylaxis, while asymptomatic VTE may be more common [28]. The Guidelines for Management of Acute Cervical Spinal Injuries recommend prophylactic VTE treatment for patients with severe motor deficits [18]. In 2004, guidelines for the prevention of VTE recommended starting all acute SCI patients on thromboprophylaxis once primary hemostasis is evident [29]. Low-molecular-weight heparin was recommended over single therapy with unfractionated heparin, compression stockings or an inferior vena cava filter [29]. A combination of these latter modalities was recommended in instances where there was a contraindication to low-molecular-weight heparin during acute phase SCI [29]. The recommendations included in both sets of guidelines were based on strong clinical evidence in the scientific literature [18,29].
There are currently no recommendations for a particular low-molecular-weight heparin. Most guidelines are based on studies employing enoxaparin for VTE prophylaxis [18,29,30]. A retrospective study comparing the rate of VTE in SCI patients on prophylactic dalteparin or enoxaparin reported clinically symptomatic DVT or PE in 1.6% of the enoxaparin group and 9.7% in the dalteparin group [30]. The difference was not statistically significant due to insufficient power [30]. The balance of evidence favours the use of enoxaparin (40 mg subcutaneous once daily) for VTE prophylaxis in the acute SCI population.
39.3.6 Nutritional Support
The early period of acute SCI is characterized by disturbed metabolic activity and increased nitrogen excretion [26,31]. While the benefits of enteral nutrition in critically ill patients are accepted, there is little information supporting early feeding in acute SCI. A pilot, randomized control trial demonstrated no differences in septic complications between patients fed within 72 hours of SCI and those fed after 120 hours of the injury [31]. Only 17 patients were included in the analysis, making it difficult to draw any conclusions from the results. A retrospective review of 27 SCI patients started on enteral feeds a median of 2 days after injury reported no major complications in the group, though two patients had feeds interrupted due to high gastric aspirates [32]. Early enteral feeding should be considered in the acute SCI population, with consideration of their hypercatabolic state, with enough nutritional support to accommodate 1.5 times the normal basal metabolic rate.
39.4 Conclusion
Understanding the pathophysiology of SCI is essential to optimizing patient management. Maintaining a mean arterial pressure ≥85 mmHg, appropriate administration of methylprednisolone, and early surgical decompression are essential to optimizing the neurological outcome. Recognition of the common medical issues encountered by SCI patients (thromboembolism, pressure ulcer, altered nutritional requirements) and their avoidance are essential to improving overall outcome. We recommend that the reader be familiar with the following key references.
References
1. Ditunno JF, Little JW, Tessler A, et al. Spinal shock revisited: a four-phase model. Spinal Cord 2004; 42: 383-95
2. Furlan JC, Fehlings MG. Cardiovascular complications after acute spinal cord injury: pathophysiology, diagnosis, and management. Neurosurg Focus 2008; 25: E13
3. Garstang SV, Miller-Smith SA. Autonomic nervous system dysfunction after spinal cord injury. Phys Med Rehabil Clin N Am 2007; 18: 275-96
4. Atkinson PP, Atkinson JL. Spinal shock. Mayo Clin Proc 1996; 71: 384-9
5. Medicine CfSC. Early acute management in adults with spinal cord njury: a clinical practice guideline for health-care providers. Washington, DC: Paralyzed Veterans of America; 2008
6. Levi L, Wolf A, Belzberg H. Hemodynamic parameters in patients with acute cervical cord trauma: description, intervention, and prediction of outcome. Neurosurgery 1993; 33: 1007-16; discussion 16-7
7. Vale FL, Burns J, Jackson AB, et al. Combined medical and surgical treatment after acute spinal cord injury: results of a prospective pilot study to assess the merits of aggressive medical resuscitation and blood pressure management. J Neurosurg 1997; 87: 239-46
8. http://www.asia-spinalinjury.org [latest accessed April 2012]
9. Kwon BK, Mann C, Sohn HM, et al. Hypothermia for spinal cord injury. Spine J 2008;8:859-74.
10. Fehlings MG, Baptiste DC. Current status of clinical trials for acute spinal cord injury. Injury 2005; 36(Suppl 2): B113-22
11. Kwon BK, Fisher CG, Dvorak MF, et al. Strategies to promote neural repair and regeneration after spinal cord injury. Spine 2005; 30: S3-13
12. Bracken MB, Shepard MJ, Hellenbrand KG, et al. Methylprednisolone and neurological function 1 year after spinal cord injury. Results of the National Acute Spinal Cord Injury Study. J Neurosurg 1985; 63: 704-13
13. Bracken MB, Shepard MJ, Collins WF, et al. A randomized, controlled trial of methylprednisolone or naloxone in the treatment of acute spinal-cord injury. Results of the Second National Acute Spinal Cord Injury Study. N Engl J Med 1990; 322: 1405-11
14. Bracken MB, Shepard MJ, Holford TR, et al. Administration of methylprednisolone for 24 or 48 hours or tirilazad mesylate for 48 hours in the treatment of acute spinal cord injury. Results of the Third National Acute Spinal Cord Injury Randomized Controlled Trial. National Acute Spinal Cord Injury Study. JAMA 1997; 277: 1597-604
15. Bracken MB. Methylprednisolone and acute spinal cord injury: an update of the randomized evidence. Spine 2001; 26: S47-54
16. Frampton AE. High dose methylprednisolone in the immediate management of acute, blunt spinal cord injury: what is the current practice in emergency departments, spinal units, and neurosurgical units in the UK? Emerg Med J 2006; 23: 550-3
17. Lee AS, MacLean JC, Newton DA. Rapid traction for reduction of cervical spine dislocations. J Bone Joint Surg Br 1994; 76: 352-6
18. [No authors listed]. Guidelines for management of acute cervical spinal injuries. Introduction. Neurosurgery 2002; 50: S1
19. Fehlings MG, Tator CH. An evidence-based review of decompressive surgery in acute spinal cord injury: rationale, indications, and timing based on experimental and clinical studies. J Neurosurg 1999; 91: 1-11
20. Gunnarsson T, Fehlings MG. Acute neurosurgical management of traumatic brain injury and spinal cord injury. Curr Opin Neurol 2003; 16: 717-23
21. Fehlings MG, Perrin RG. The timing of surgical intervention in the treatment of spinal cord injury: a systematic review of recent clinical evidence. Spine (Phila Pa 1976) 2006; 31: S28-35; discussion S6
22. Fehlings MG, Arvin B. The timing of surgery in patients with central spinal cord injury. J Neurosurg Spine 2009; 10: 1-2
23. Fehlings MG, Rabin D, Sears W, et al. Current practice in the timing of surgical intervention in spinal cord injury. Spine (Phila Pa 1976) 2010; 35(21 Suppl): S166-73
24. Chen Y, Devivo M, Jackson A. Pressure ulcer prevalence in people with spinal cord injury: age-period-duration effects. Arch Phys Med Rehabil 2005; 86: 1208-13
25. Gélis A, Dupeyron A, Legros P, et al. Pressure ulcer risk factors in persons with SCI: part I: acute and rehabilitation stages. Spinal Cord 2009; 47: 99-107
26. Nockels RP. Nonoperative management of acute spinal cord injury. Spine (Phila Pa 1976) 2001; 26: S31-7
27. Fehlings MG, Vaccaro A, Wilson JR, et al. Early versus Delayed Decompression for Traumatic Cervical Spinal Cord Injury: Results of the Surgical Timing in Acute Spinal Cord Injury Study (STASCIS). PLoS One 2012; 7(2): e32037
28. Furlan JC, Fehlings MG. Role of screening tests for deep venous thrombosis in asymptomatic adults with acute spinal cord injury: an evidence-based analysis. Spine (Phila Pa 1976) 2007; 32: 1908-16
29. Geerts WH, Pineo GF, Heit JA, et al. Prevention of venous thromboembolism: the Seventh ACCP Conference on Antithrombotic and Thrombolytic Therapy. Chest 2004; 126: 338S-400S
30. Slavik R, Chan E, Gorman S, et al. Dalteparin versus enoxaparin for venous thromboembolism prophylaxis in acute spinal cord injury and major orthopedic trauma patients: ‘DETECT’ trial. J Trauma 2007; 62: 1075-81
31. Dvorak M, Noonan VK, Bélanger L, et al. Early versus late enteral feeding in patients with acute cervical spinal cord injury: a pilot study. Spine (Phila Pa 1976) 2004; 29: E175-80
32. Rowan C. Is early enteral feeding safe in patients who have suffered spinal cord injury? Injury 2004; 35: 238-42
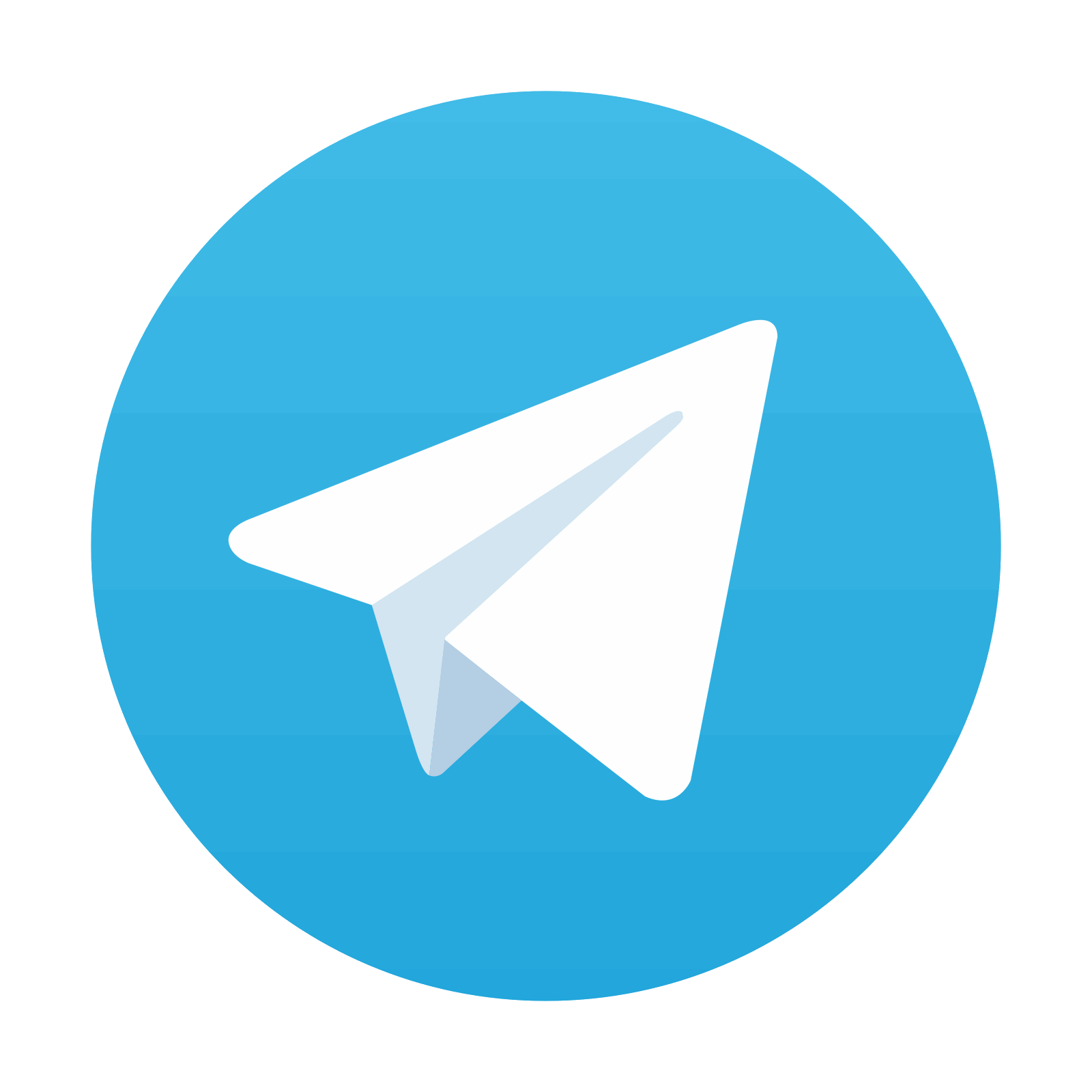
Stay updated, free articles. Join our Telegram channel

Full access? Get Clinical Tree
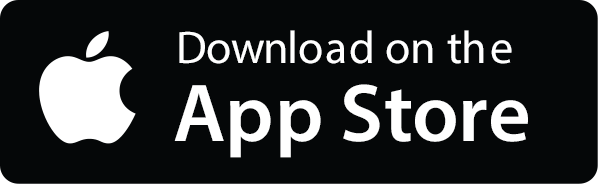
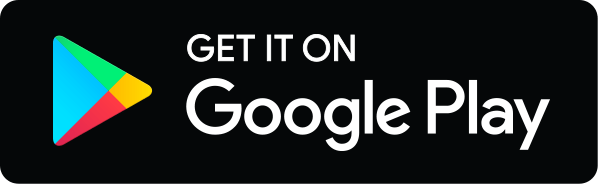