12.1 Introduction
Imaging, in particular group analysis of structural MRI in homogenous patient populations, has provided further insight into disease pathophysiology and refined the cortico-thalamic network theory of genetic generalized epilepsy (GGE).1–4 The identification of key regions within networks provides putative biomarkers that can be targeted by clinical treatment strategies. Connectivity analysis provides a versatile tool to assess pathological interactions of different regions in GGE.
12.2 Thalamus
The thalamus as well as thalamocortical networks are critically important for the generation of generalized seizures. Simultaneous EEG-fMRI studies found that hemodynamic changes associated with generalized spike-wave discharges (GSWDs) could be consistently observed in the thalamus as well as within areas belonging to the default mode network (DMN).5, 6 Hence, thalamus and DMN have been the focus of investigations aiming to elucidate the pathophysiology of GGE syndromes.
There is compelling evidence that dysfunction in the cortico-thalamic circuitry contributes to the pathogenesis of generalized seizures.2, 3, 7–9 Cortico-thalamic interactions in GGE have been characterized by morphometric covariance,3 functional,7, 8 and anatomic connectivity.9, 10
In a recent study on GGE patients, Wang and colleagues utilized bilateral thalamic medial dorsal nuclei and pulvinar, identified in a structural analysis as seed regions for functional connectivity (FC) analysis, and observed reduced connectivity between thalamic nuclei and bilateral orbital frontal cortex, caudate nucleus, putamen, and amygdala,9 demonstrating the different involvement of the thalamocortical networks in GGE. Using similar methods, Kim et al. found decreased thalamocortical FC between anteromedial thalamus and medial prefrontal cortex and precuneus/PCC in a mixed cohort of GGE patients.7 Moreover, greater reduction in FC between anteromedial thalamus and medial prefrontal in relation to increasing disease duration in a mixed GGE group suggested a cumulative influence of the disease-underlying factors on thalamocortical connectivity.
A recent resting-state functional MRI study examined 97 GGE patients and found increased FC in thalamocortical networks.8 The thalamus was functionally divided into subregions analogous to histological parcellations,8 which is in accord with distinct cortical lobes relating to five parallel corticothalamic networks.11 Patients showed increased FC strength in four corticothalamic networks compared with controls, involving (1) the prefrontal-thalamic, (2) motor/premotor-thalamic, (3) parietal/occipital-thalamic, and (4) temporal-thalamic networks but no changes in sensory-thalamic network
Abnormalities in the motor-/premotor-thalamic system may be expected in view of the sustained muscle rigidity as well as rapid muscle contraction/relaxation experienced by patients during seizures. The premotor cortex and specific nuclei (pulvinar and ventrolateral nucleus) showed increased FC within the motor-thalamic but not the sensory-thalamic network. This may be attributed to the disruption of functional integrity in the sensorimotor network of the neocortex12 and may reflect the higher excitability of the motor system of patients with GGE compared with controls.13 Altered connectivity of the posterior aspect of the middle temporal gyrus may relate to the abnormal semantic processing observed in many patients with GGE.14 Similarly, altered prefrontal-thalamic connectivity in different subtypes suggests that these network changes may be a common feature of GGE.
Figure 12.1. Comparison of voxel-wise thalamocortical functional connectivity between patients with generalized epilepsy and healthy controls. The left column shows cortical lobes and corresponding thalamic subregions obtained by using the winner-take-all approach. The right column shows the between-group difference in functional connectivity strength. Abnormal voxels are labeled by warm colors and rendered on cortical surface models and thalamic sections (green). The color scale represents t values, as determined with 2-sample t tests (p < .05, corrected). The z plane coordinates refer to the Montreal Neurological Institute space.
12.3 Default Mode Network
The DMN is composed of precuneus/posterior cingulate cortex, medial prefrontal cortex, lateral parietal cortex, and inferior temporal cortex.15, 16 The nodes of this network were shown to exhibit coherent spontaneous fluctuations of their resting-state BOLD fMRI time courses,15 and constitute a system engaged during internally focused activities, including evaluating salience of internal and external cues, remembering the past, and planning the future.15 Of note, cortical DMN regions are known to be functionally and structurally connected to thalamus,11 and deactivation of the default mode areas along with thalamic activation during GSWDs was consistently found by EEG-fMRI studies.5, 6 Dynamic connectivity maps exploring thalamic and DMN interactions during the preictal, ictal, and postictal states of absence seizures show an evolving anticorrelation between the thalamus and the DMN, which is consistent with an inhibitory effect of seizures on the default mode of brain function, gradually fading out after seizure onset.17 Two recent resting-state studies found that DMN connectivity is lower in patients with GGE than in healthy controls. Impaired DMN connectivity found in studies probing the integrity of structural and functional studies might relate to impaired consciousness observed in GGE patients, frequently reported in other mental diseases.18 Another resting-state study on a large sample of patients with GGE showed that connectivity is further reduced in the subgroup with treatment-resistant seizures.19 Furthermore, in these studies, the strength of DMN connectivity was negatively correlated with disease duration.20, 21 It is notable that DMN connectivity declines with duration of epilepsy20, 21 and that this effect is significantly more pronounced in treatment-resistant patients.19 Although longitudinal studies would be needed to confirm that seizure duration correlates with changes in DMN FC over time, these findings might indicate a detrimental effect of epilepsy-related factors on the brain, supporting a clinical decision to treat aggressively.
Figure 12.2. Dynamic changes of the default-mode network during absence seizures. (A) Significant positive (warm color) and negative (cool color) correlations were derived from one-sample t tests (uncorrected heighted threshold p < .05, and extend threshold k = 5 voxels). The results are presented on inflated surface maps for preictal (upper), ictal (middle), and postictal (lower) periods from −22 to +32 s, relative to seizure onset. (B) Significant change of the default mode network functional connectivity map across the preictal, ictal, and postictal intervals (one-way within-subject ANOVA analysis, FDR-corrected height threshold p < .05, and extent threshold k = 5 voxels). Significant network changes (warm color) are presented on inflated surface maps (upper) and axial maps (lower). (C) Mean functional connectivity strength (z value) time courses in five anatomical clusters (thalamus, caudate, and inferior parietal lobule). Error bars refer to the standard error of the mean across all seizures. The mean seizure duration is shown in the red box.
12.4 Frontal Lobes
GGE is typically associated with normal intelligence, but many patients exhibit specific frontal-lobe cognitive deficits.22 The presence of specific hypo- and hyperconnectivity of frontal lobe areas indicated that GGE may not homogeneously affect the entire brain, and that the frontal lobe might be the epicenter of the effects of GGE.23–25
Vollmar et al. demonstrated aberrant patterns of FC between the supplementary motor area (SMA) and the motor cortex during a working memory task, which was paralleled by abnormal structural connectivity metrics.23, 24 Precipitation of myoclonic jerks by cognitive tasks is a known trait of some JME patients, known as praxis induction.26, 27 Therefore, the abnormal connectivity of SMA, prefrontal cognitive areas, and motor system was interpreted as a potential substrate underlying seizures triggered by cognitive effort. Regions of cortical hyperexcitability may overlap with areas physiologically activated during cognitive or motor activities. Hence, a complex task involving several functional cortical systems may summon a “critical mass of activated cortex,” which leads to seizure precipitation. The abnormal motor cortex coactivation during a working memory task may represent the functional correlate of this mechanism.
In an EEG-fMRI study, Szaflarski et al. found greater spike-related activation of paracingulate cortex in valproate-unresponsive GGE patients compared with valproate-responsive subjects.28 A further study detected a positive correlation between GSWD frequency and FC between paracingulate and frontal regions related to attention and execution functions as well as precentral gyrus. This might indicate an increased crosstalk between motor, default mode, and executive networks in patients with frequent GSWDs,29 suggesting that differences in paracingulate connectivity may be associated with frequent GSWDs and uncontrolled seizures in GGE. Recent investigations combining resting-state fMRI and DTI found enhanced interhemispheric functional synchronization as well as anatomic connectivity between bilateral orbitofrontal areas and anterior cingulate cortex (ACC) during interictal period.25, 30, 31 Ji and colleagues found increased functional and structural connectivity between bilateral ACC in chronic GGE patients who were drug-resistant. The presence of both anatomic and functional changes suggested that the bilateral ACC is relevant to the pathophysiology of GGE. Results of follow-up studies of intractable GGE patients who received corpus callosotomy demonstrated effectively reduced seizure frequency in them. To minimize cognitive impairment (such as cerebral disconnection syndrome) post-intervention, future investigations into the precision and actual feasibility of resective surgery are however paramount.
Figure 12.3. Alterations of structural connectivity in juvenile myoclonic epilepsy (JME). (A) Summary of findings in JME patients. The anterior presupplementary motor area (SMA) cluster showed reduced connectivity to anterior frontopolar regions (B) and increased connectivity to the medial central region (C) as well as to descending motor pathways (D). The posterior SMA cluster showed reduced connectivity to the central region (E) but increased connectivity to the temporal neocortex (F) and occipital cortex (G). (H) Functional connectivity between the prefrontal cortex and SMA, as found with fMRI, correlated significantly with the structural connectivity shown with diffusion tensor imaging (DTI). (I) Connectivity of the SMA also correlated with disease activity.
12.5 Temporal Lobes
While the majority of connectivity analyses in GGE have focused on frontothalamic networks, there is limited understanding as to whether temporal lobe structures may also contribute to epileptogenesis and neurobehavioral comorbidities. Recent imaging analyses provided evidence for reduced hippocampal volumes,32, 33 aberrant cortical features of lateral temporal areas,34 and altered developmental trajectories of fronto-temporoparietal cortices in JME.35 In absence epilepsy cohorts, reduced gray matter volumes of lateral temporal cortices as well as mesiotemporal structures were also detected.36–38 Fewer studies, however, have assessed connectional properties of mesiotemporal and lateral temporal regions.
Diffusion MRI studies on mixed GGE cohorts documented aberrant white matter properties of several fiber tracts connecting temporal lobe structures, including inferior longitudinal fasciculus, cingulum, and the temporal branch of the superior longitudinal fasciculus.39, 40 In one of the former reports, a subgroup analysis comparing patients with JME against the remainder GGE group failed to detect syndrome-specific effects.40 Another study detected reduced fractional anisotropy of fornix and uncinate fasciculi in patients with JME compared with controls, while similar findings could not be substantiated for the GGE subgroup with GTCS only.41 In JME, Vollmar and colleagues identified enhanced diffusion-based connectivity between SMA and lateral temporal cortex,41 while a graph-theoretical analysis based on a diffusion MRI dataset reported hyperconnectivity within a subnetwork encompassing motor areas, parietal cortices, cerebellum, as well as the right hippocampus.42 Abnormal connectional properties of temporal structures were also detected by a graph-theoretical diffusion MRI study on childhood absence epilepsy (CAE). Xue and collaborators reported altered nodal properties of the inferior temporal gyri, impaired white matter connections of lateral temporal cortical nodes, as well as disconnection within subnetworks linking hippocampi and basal ganglia with parietal default-mode areas.43 In a cohort of GGE with GTCS only, an analysis of thalamocortical network integrity relying on the covariance of gray matter MRI markers found evidence of increased correlations between thalamus and lateral temporal areas, along with decreased coupling between thalamus and temporopolar cortices.44
With regard to functional MRI studies, EEG-fMRI analyses investigating the imaging correlates of GSWD in several GGE cohorts pointed to the involvement of additional cortical areas beyond the classically identified DMN, such as lateral temporal5, 45, 46 or temporopolar cortices.47 In a mixed GGE cohort, enhanced resting-state FC between pulvinar and bilateral middle temporal gyrus was identified, and interpreted as a putative imaging correlate for dysfunctional semantic processing in GGE.8
On balance, imaging studies addressing the organization of functional and structural networks in GGE documented abnormalities also encompassing temporal lobe structures, which may be less prominent than those affecting frontal regions.
12.6 Basal Ganglia
The basal ganglia, a set of brain structures related to motor control, consist of four main nuclei: the striatum, globus pallidus, subthalamic nucleus, and substantia nigra (SN). The basal ganglia is proposed to be implicated in the modulation of the generalization of epileptiform discharges in patients with GGE.48 Anatomical and functional connections between basal ganglia and cerebral cortex are characterized by parallel, predominantly segregated, closed-loop projections.48 In EEG-fMRI findings, basal ganglia activity is related to interictal slow wave discharges and to generalized seizure in patients with secondarily generalized epilepsy or GGE.49, 50
One resting-state fMRI study, using independent component analysis (ICA), identified a resting-state functional network predominantly encompassing basal ganglia in both healthy controls and patients with GGE. Basal ganglia network in patients, compared with controls, showed significantly more integration, either during interictal epileptic discharges or without epileptic discharge.51 Specifically, functional connections among the main nuclei in basal ganglia, amygdalae, and hippocampus were strengthened in GGE patients compared with controls. In addition, a greater FC increase was found in bilateral caudate nucleus and the putamen in patients with epileptic discharges when comparing with patients without interictal discharges. Functional connectivity in bilateral caudate nuclei positively correlated to the discharge number in the patients with epileptic discharges; meanwhile, epilepsy duration was positively related to the FC in bilateral putamen in the patients without epileptic discharges. The modulation enhanced the integration in basal ganglia networks of patients, and modulation in patients was stronger with than without GSWDs. These findings confirmed that the basal ganglia networks are related to the GSWDs. Enhanced integration suggests that the basal ganglia are implicated in the modulation of epileptic discharge generalization in GGE.
Further evidence for the involvement of the basal ganglia in the modulation of epileptic discharges has been also suggested by DTI studies examining structural connectivity in absence epilepsy.52 In a recent EEG-fMRI study, spike-correlated activation changes in the basal ganglia were also found in JME patients.47 Of particular relevance to JME is the role of basal ganglia in executive and attentional function. The disruption of functional and structural connectivity between basal ganglia and superior frontal and SMA regions was found in JME patients, which may be associated with changes in executive behavior and implicated as anatomical correlates of myoclonus in JME patients.10 These alterations of basal ganglia–thalamo–cortical loops implicate specific basal ganglia network dysfunction in GGE patients.
12.7 Cerebellum
The cerebellum shares reciprocal connections with thalamus, cortex, and basal ganglia through which it could, theoretically, modulate ictogenic activity throughout the brain.48 The role of the cerebellum in GGE, however, is still unclear, with cerebellar atrophy often found in epilepsy patients.48 Although the cerebellum is not thought to be a primary source of ictogenesis in GGE, both structural and functional neuroimaging studies showed loss of cerebellar connectivity with thalamus, basal ganglia, and cortex.29, 51, 53 Reduced cerebellar FC was also shown to be associated with frequent discharges51 and often related to resistance to treatment.29 These findings suggest that cerebellum could represent a modulator of ictogenic circuits.
12.8 Influence of Genetic Factors on Functional MRI Abnormalities
In order to increase the yield of genetic studies and understand gene variants and complex phenotype interactions, a strategy originating from psychiatry research consists of characterizing intermediate biological phenotypes, or “endophenotypes.”54
Juvenile myoclonic epilepsy, the most common GGE syndrome, is a presumed neurodevelopmental condition where complex polygenetic inheritance is suspected in most cases, though Mendelian inheritance also exists.55, 56
The heritability in JME is high (0.7),57 and particularly high among first-degree relatives, i.e., siblings and offspring, with 30% of affected first degree relatives also suffering from JME.58, 59 Twin studies show very high monozygous concordances with nearly always identical syndromes within concordant pairs.60, 61 However, so far, identifying disease-culprit genes has proven difficult.
Given the strong link between genetic variations, neurodevelopment, and function of brain regions,62 advanced functional and structural imaging techniques can be used to identify such endophenotypes. Ideally, linking these MRI endophenotypes to candidate genes has the potential to characterize genotype effects on neural networks and, vice versa, may increase the power of genetic analyses by using MRI phenotypes as a quantitative trait.63, 64
Cognitive fMRI activation networks are assumed to be highly heritable, as, e.g., demonstrated in a large cognitive fMRI study in healthy fraternal and identical twins.65 So far, MRI endophenotype research has been mainly employed in schizophrenia and autism and most studies investigate unaffected siblings of patients to control for possible confounders of disease activity and medication and ensuring at the same time comparability of age and environmental factors.66, 67
There are only few studies investigating MRI endophenotypes in epilepsy and only one in GGE.64 Wandschneider and colleagues were the first to employ this concept in JME by using an fMRI n-back working memory task. In a previous study employing the same paradigm in JME patients and controls,23 patients showed comparable working memory performance to controls. Thus, with increasing task demand, patients demonstrated an abnormal coactivation and increased FC of the motor cortex and cognitive networks. In a follow-up study24 investigating DTI-tractography and FC in the same patients, structural and functional connectivity was increased between primary motor and prefrontal cognitive networks, whereas within prefrontal cognitive networks, connectivity was decreased. Findings provide possible explanations for cognitively triggered myoclonic jerks, commonly termed as “praxis induction,” a phenomenon highly associated with JME,27 and also subtle frontal lobe cognitive deficits as reported by several neuropsychological studies in JME.22
Figure 12.4. Group fMRI activation from working memory and group differences between juvenile myoclonic epilepsy (JME) siblings and healthy controls. Group fMRI activation maps from JME siblings and healthy controls (A–C) show cortical activation for the three different task conditions: motor cortex and supplementary motor area for “0 Back” (A), bilateral frontal, and parietal activation for “1 minus 0 Back” and “2 minus 0 Back” (B, C). Lower row (D–F), activation patterns in siblings compared to controls (inclusively masked for task-dependent deactivation maps of healthy controls (p < .001 uncorrected with 20 voxel threshold extent): no significant difference for the “0 back” condition (D), but attenuated deactivation in the motor cortex (E) and the supplementary motor area (F) with increasing task demand in the working memory contrasts was seen.
Similarly to JME patients, motor cortex coactivation and increased FC between motor and working memory networks were also demonstrated in seizure-unaffected siblings,68 hence suggesting that these imaging traits may constitute an endophenotype of JME. In a recent twin study65 mentioned above, fMRI activation patterns during the same n-back working memory task have been shown to be significantly heritable and regions of interest identified by Wandschneider et al.,68 i.e., the precentral gyrus and SMA, have been among the regions with the highest heritability estimates. Thus, not only seizures but also neurobehavioral comorbidities are likely part of the genetically determined neurodevelopmental dysfunction, and, as similar imaging and behavioral abnormalities have been shown in unaffected siblings,69–71 are not explained by antiepileptic drug side effects or disease severity.
GGE and its comorbidities may hence reflect different degrees of disease with a shared underlying pathological condition, as further corroborated by behavioral studies in genetic generalized epilepsies that identified cognitive impairment even prior to disease onset.72
12.9 Conclusion
Connectivity analysis techniques represent sensitive methods to elucidate disease pathomechanisms in GGE. The thalamus and DMN are the two main networks involved in the generation of GSWD and, arguably, in the pathogenesis of GGE. Altered thalamic FC with different cortical areas may also relate the semiology and clinical manifestations in GGE patients. More pronounced reduction of connectivity in the DMN in drug-resistant patients may provide a biomarker for treatment resistance. Abnormal connectivity of frontal areas may relate to the specific profile of cognitive dysfunction observed in GGE. Increased FC between prefrontal cognitive areas and the motor system in JME suggests that alterations of SMA connectivity might be the “missing link” between seizure‐facilitating mechanisms and seizure type. Increased functional and structural connectivity between bihemispheric ACC in chronic GGE patients suggests that interhemispheric communications may represent a target for future treatment exploration in patients with GGE and intractable seizures. Increased functional and structural connectivity integration found in basal ganglia provided evidence that this could be an important modulator in GGE. The findings of connectivity analyses addressing the cerebellum are still preliminary, though decreased structural and functional connectivity of the cerebellum may also play a role in ictogenesis. Prospective studies are needed to establish the causality and rule out the confounding effects of AED treatment.
Though imaging genetic studies in GGE patients are rare, the cognitive task fMRI studies of unaffected, cognitively intact first-degree relatives of JME suggested that quantitative imaging trait in combination with candidate gene studies could be used in the future for exploring genetic findings in epilepsy.
References











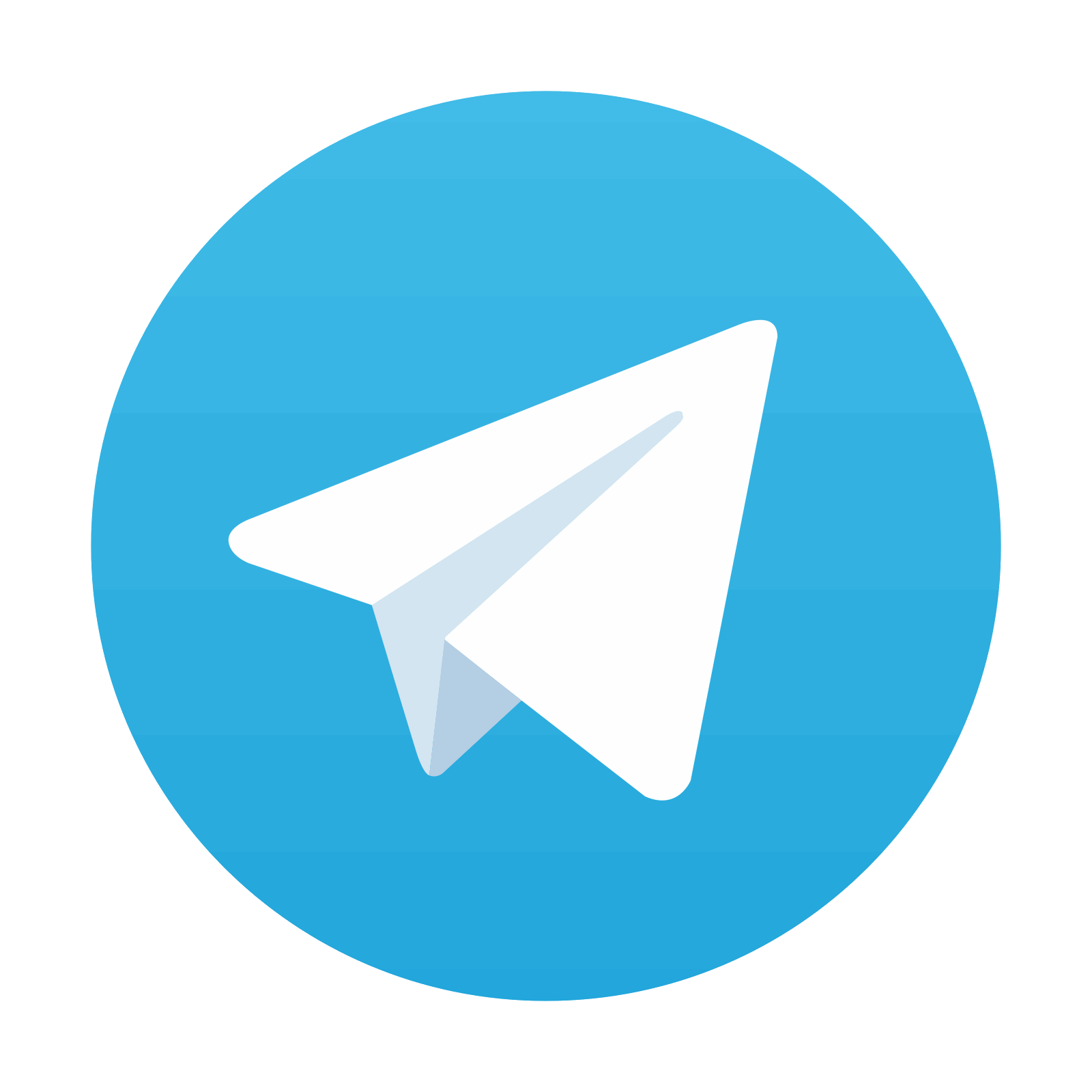
Stay updated, free articles. Join our Telegram channel

Full access? Get Clinical Tree
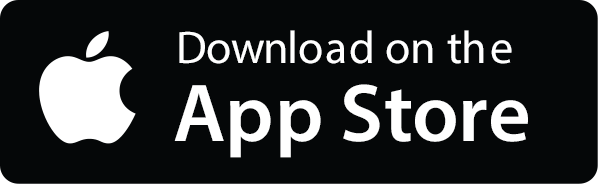
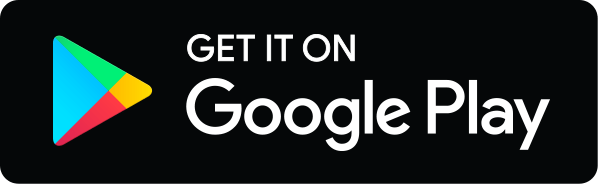
