15.1 Introduction
Despite advances in antiepileptic drug (AED) therapy, about one third of patients with epilepsy are resistant to several, if not all, AEDs, even though these drugs act by diverse mechanisms.1 Pharmacoresistant epilepsy is defined as failure of adequate response to two tolerated and appropriately prescribed AED schedules (whether as monotherapies or in combination) to achieve sustained seizure freedom.2 Drug response in epilepsy applies not only to seizure control, albeit the defining symptom of the disease, but also to affective, behavioral, and cognitive symptoms, related to the disease and/or antiepileptic treatment. Many patients rank cognitive impairment as the most relevant complaint and, vice versa, consider the side-effect profile of specific AEDs as more important than achieved seizure control by these drugs.3, 4 Currently, we have no means to predict how a patient will respond to a drug, in terms of both efficacy and side effects. Rather than waiting for the next seizure or side effects to occur in order to assess efficacy and tolerability through trial and error, we ultimately need clinically meaningful surrogate markers for therapeutic guidance that measure how a patient feels and functions, and predict the medication effect. This chapter focuses on the currently available imaging biomarkers for assessment and prediction of drug response and refractoriness.
15.2 Imaging Markers of General Treatment Response
A limited number of studies investigated the relationship of metabolic and structural imaging markers and general treatment response in epilepsy, i.e., not related to specific AEDs. These studies mainly investigated patients with temporal lobe epilepsy (TLE) and concluded that more extensive damage, probably related to an epilepsy precipitating injury or to active epilepsy itself, is associated with poorer treatment response.
A proton magnetic resonance spectroscopy (MRS) study contrasting patients with TLE who responded to the first AED to those who continued to have seizures reported significantly reduced N acetyl aspartate/creatinine (NAA/Cr) ratios in the treatment failure group. NAA/Cr ratios are considered markers of axonal damage and/or dysfunction.5 A voxel-based morphometry (VBM) study6 in a large TLE cohort (n = 165) identified more widespread gray matter atrophy, extending beyond the medial temporal lobe, in treatment-resistant and “relapsing-remitting” cases when compared to treatment-responders. However, seizure frequency was higher and age of onset younger in treatment-resistant cases, thus structural changes may not be specific to treatment response but attributed to overall worse epilepsy. Seizure recurrence after AED withdrawal has also been associated with structural markers, e.g., the degree of hippocampal atrophy.7
15.3 Pharmacological fMRI Studies
Regional network effects of distinct AEDs have mainly been studied with functional MRI (fMRI). Over the last years, fMRI studies have led to major advances in understanding epilepsy and hence provide the appropriate framework to study specific AED effects in different epilepsy syndromes.
The following commonalities have been identified using fMRI in several epilepsy syndromes:
1. Dysfunctional networks appear to extend far beyond the presumed main disease focus.8−10
2. These regions include major brain networks relevant to cognitive function, such as the working memory and default mode network.10−12
3. The default mode network has been identified as a key network to be implicated in both cognitive dysfunction and seizure manifestation.12,13 In addition, critical disease hubs colocalize with major subsystems of the default mode network, i.e., the medial temporal lobe; hence function within these networks is directly modulated by disease factors, such as seizure frequency and disease duration.14
Despite these advances in understanding disease processes in epilepsy and its comorbidities, studies assessing the effect of and response to AEDs, so called pharmaco-fMRI (ph-MRI) studies, are rare and usually of small sample sizes.15 This may be due to several methodological difficulties: The signal change in fMRI related to the drug is low; hence drug effects are generally studied as an interaction effect in task-related fMRI, i.e., task-related activation patterns for a drug are compared to those without the drug or placebo. Drugs can influence the blood-oxygen-level-dependent(BOLD) signal both at a neuronal and vascular level, complicating the interpretation of the effects observed. As the BOLD signal is contaminated by low-frequency noise, detection of slowly evolving medication effects can be challenging.16 A more epilepsy-specific problem is that patients usually are already on AED treatment, therefore the study design has to control for effects of comedication in addition to other confounders, such as disease activity, syndrome, and comorbidities.17 Nevertheless, ph-MRI has the major advantage that it can investigate effects of pharmacological agents at a network level and remotely from regions of highest target receptor densities, whereas PET and molecular studies can define target receptor occupancy and affinity without necessarily translating effects to large-scale networks.16 Hence fMRI enables a system evaluation of networks underlying behavioral effects of a drug, independent of its biochemical mechanism of action. As AEDs often target several receptor subtypes with varying regional distribution and AED efficacy differs across these targets, fMRI can monitor the combined effect of these interactions across multiple brain regions.18 A further advantage is that fMRI does not use ionizing radiation and has no known biological side effects.
Though not in epilepsy, ph-MRI has been widely applied in other CNS diseases, mainly affective disorders, addiction, and schizophrenia.19 Overall, these studies show that pharmacological agents with known clinical efficacy have consistent effects on disease relevant neural networks and modulation of brain activation at treatment baseline is a potential surrogate marker for long-term efficacy.19 Similar conclusions could potentially be drawn for anticonvulsive treatment with a larger body of studies present.
In the following, effects of specific antiepileptic agents on brain networks will be discussed.
15.3.1 Carbamazepine and Oxcarbazepine
Jokeit and colleagues20 were the first to employ ph-MRI in an epilepsy cohort. They studied the relationship of mesiotemporal fMRI activation and carbamazepine (CBZ) concentrations in 21 patients with refractory TLE. Most patients were on monotherapy, and a visuospatial memory retrieval task was employed. The extent of task- and syndrome-specific fMRI activation within the medial temporal lobe was negatively correlated with the CBZ serum levels. The effect was most marked with close to toxic drug levels. However, there were no psychometric data available to relate effects to memory function in these patients.
Employing a graph theoretical approach and resting-state fMRI in a TLE cohort treated with CBZ or oxcarbazepine (OXC) and comparing to patients who were on other AEDs, Haneef and colleagues21 report altered hubness in those on CBZ/OXC, i.e., less highly connected nodes connecting distant parts of the brain. Whereas betweenness centrality, or hubness, was reduced within the limbic circuit and thalamus with CBZ/OXC use, it was increased in default mode regions, i.e., cingulate and posterior cingulate/precuneus.
Previous data in TLE suggest a “redistribution” of hub regions with high betweenness centrality to mainly paralimbic and temporal association cortices.22 It is therefore tempting to speculate that CBZ/OXC may have a region-specific effect on disease-related network changes.
15.3.2 Valproate
In a placebo-controlled, combined transcranial magnetic stimulation (TMS) and fMRI study, valproate (VPA) and lamotrigine (LTG) demonstrated network-specific effects. When TMS was applied over the motor region, both agents reduced TMS-specific effective connectivity between the primary motor and premotor cortex and the primary motor and supplementary motor area (SMA). Only LTG-treatment was associated with increased effective connectivity between the left dorsolateral prefrontal cortex and anterior cingulate when TMS was applied over the prefrontal cortex.23
For VPA, similar effects are seen in juvenile myoclonic epilepsy (JME). JME has been associated with increased functional and structural connectivity between central motor and prefrontal cognitive networks, likely accounting for cognitively triggered jerks, a reflex trait highly associated with the syndrome.11,24,25 Abnormal motor cortex coactivation with cognitive networks during an fMRI working memory task in JME was shown to be modulated by disease factors, i.e., the trait was enhanced with more frequent seizures and in the morning, when seizures occur more frequently due to the typical chrono-dependency of the syndrome. Vice versa, abnormal coactivation was attenuated with increasing VPA dose, consistent with the clinical impression that VPA is effective in JME, particularly in treatment of myoclonic jerks, and not necessarily associated with cognitive side effects.26
An EEG-fMRI study attributes VPA treatment response among patients with idiopathic generalized epilepsy (IGE) to regional differences in generalized spike and wave discharge (GSWD) generators.27 Spike-related activation in VPA-resistant patients was increased in the medial frontal cortex and anterior insula bilaterally when compared to VPA responders. The effect remained apparent in the medial frontal cortex even after controlling for spike frequency, but there was no difference between groups in the thalamus. Hence in the VPA-resistant group, seizure generators may be more extensively cortically distributed.
15.3.3 Levetiracetam
Several studies attribute a favorable cognitive profile to levetiracetam (LEV),28 which has been described superior to CBZ by some authors.29
Consistent with neurobehavioral data, fMRI studies show a beneficial effect of LEV to cognitive networks. Wandschneider and colleagues30 studied fMRI activation and deactivation patterns during a verbal and visuospatial working memory task in 53 left and 54 right TLE patients, comparing those treated with (n = 59) to those without (n = 48) LEV. The effect of LEV on functional network activations was task- and syndrome-specific, as well as dose-dependent: patients on LEV showed an augmentation of task-related deactivation in the diseased temporal lobe compared to patients without LEV; more specifically, this effect was seen in the left midtemporal gyrus in left TLE during the verbal, and the right hippocampus in right TLE during the visuospatial task and became more apparent with increasing LEV dose. As patients on LEV showed similar task-related deactivation patterns to healthy controls, LEV appears to be associated with restoration of normal fMRI activation.
Though out-of-scanner neurobehavioral data, including frontal lobe cognitive measures, did not differ between those treated with LEV and those without, LEV-modulated fMRI deactivation patterns can be interpreted as a beneficial drug effect. This is corroborated by data in healthy subjects and epilepsy patients where progressive deactivation of mesial temporal structures during cognitive tasks is observed with improved performance.12,31
Functional MRI data from individuals with amnestic mild cognitive impairment, which is associated with a risk of Alzheimer’s disease, demonstrated that dysfunctional, increased hippocampal activation in the dentate gyrus/CA3 was normalized by low-dose LEV treatment with improvement of memory performance.32,33 Hence across different disease entities, LEV appears to have a localization-specific effect, which is relevant to both seizure generation and propagation, and cognitive performance via a major hub of the default mode network.
15.3.4 Topiramate and Zonisamide
Both topiramate (TPM) and zonisamide (ZNS) are broad-spectrum AED and TPM is further approved for migraine prophylaxis.34,35 Both contain a sulfamate moiety responsible for its weak carbonic anhydrase inhibitor mechanism.34,35
For TPM, cognitive dysfunction has been described in patients with epilepsy, those with migraine, and healthy controls, characterized by reduced attention, psychomotor speed, short-term memory, and, more specifically, impairment of expressive language and working memory. These deficits are noted even after single-dose administration and on steady dose in mono- or combination therapy, irrespective of seizure control. Psychometric measures consistently improve after significant dose reduction or discontinuation.37−41 Zonisamide treatment leads to similar, probably less pronounced neurocognitive impairment.41,42
Topiramate is the AED most studied in ph-MRI trials, though in relatively small cohorts. Five fMRI studies employed expressive language tasks in 2 healthy subjects, 5 to 16 epilepsy patients, and 10 migraine patients after a single dose or on steady-state TPM treatment. Combined results convey a pattern of reduced activation in language relevant regions, i.e., dominant inferior and middle frontal gyri, superior temporal gyrus,43−45 and a failure to deactivate task-negative regions, including the default mode network.44,46,47 Successful task execution in general has been associated with effective deactivation of task-negative areas.48,49
Figure 15.1. Group comparisons between patients with and without levetiracetam (LEV) during two working memory (WM) fMRI paradigms. Whereas healthy controls and patients on LEV show similar patterns of deactivation, patients without LEV show less deactivation in the medial temporal lobe areas than both controls and patients on LEV in either lateralizing task (Figure 15.1A). During the verbal WM task, left TLE patients without LEV significantly fail to deactivate the left midtemporal gyrus (Figure 15.1B; left TLE without LEV > left TLE with LEV, p < .001, 20 voxels threshold extent). During the right-lateralizing visuospatial task, patients with right TLE who are not treated with LEV fail to deactivate the right hippocampus (Figure 15.1B; right TLE without LEV > right TLE with LEV, p < .001, 20 voxels threshold extent). A post hoc analysis in patients treated with LEV demonstrated a dose-dependent effect of mesial temporal lobe deactivation through LEV. The lower the LEV dose, the lesser the right hippocampus is deactivated during the visuospatial WM task (Figure 15.1C; p < .001, 20 voxels threshold extent). A similar dose effect is observed in left TLE patients during the verbal WM task at a lower level of significance (Figure 15.1C; p < .05, uncorrected): the left > right hippocampus becomes less strongly deactivated with lower LEV dose. Figure 15.1C was inclusively masked for task-related deactivation networks (p < .05). CTR = healthy controls; LTLE = left temporal lobe epilepsy; RTLE = right temporal lobe epilepsy.
A recent study50 investigated commonalities and differences of TPM and ZNS effects on fMRI activation patterns during a verbal fluency task in a larger cohort of focal epilepsy patients (TPM = 32, ZNS = 51). Consistent with the previous studies and behavioral data reporting similar cognitive impairment patterns of TPM and ZNS, both AEDs led to decreased activations in language-associated frontal and working memory and sustained attention parietal lobe networks compared to a patient control group on LEV. In addition, TPM appeared to be specifically associated with attenuation of task-related deactivation, including subsystems of the default mode network implicated in language processing (i.e., right inferior parietal lobule, supramarginal gyrus).
Both AEDs contain a sulfamate moiety, and specific detrimental effects on verbal intellectual abilities have also been described in a related drug, sulthiame.51 Sulfa-compound containing drugs share the carbonic anhydrase inhibition mechanism and the mechanism underlying the similar fMRI changes in TPM and ZNS may be comparable to acetazolamide, another carbonic anhydrase inhibitor, which has been shown to increase blood flow with constant oxygen consumption, leading to an enhancement of the resting BOLD signal and decrease of the activation BOLD.52,53
15.4 Molecular Imaging Biomarker of Drug Resistance
There are several hypotheses that explain the mechanisms associated with pharmacoresistance in epilepsy. Current theories on the causes of pharmacoresistance in epilepsy include the transporter hypothesis, the target hypothesis, the network hypothesis, the gene variant/methylation hypothesis, and the intrinsic severity hypothesis. However, none of these hypotheses is currently a stand-alone theory that is able to convincingly explain how drug resistance arises in human epilepsy.54
15.4.1 The Transporter Hypothesis
The drug transporter hypothesis proposes that pharmacoresistance is related to increased expression of multidrug efflux transporter proteins such as P-glycoprotein (Pgp). These proteins are thought to prevent AED entry by actively extruding AEDs from their target site.55 Multidrug efflux transporters are highly expressed in capillary endothelial cells and astrocytic foot processes that form the blood-brain barrier (BBB). They limit intracellular concentration of substrates by pumping them out of the cell through an active energy-dependent mechanism. Pgp (encoded by the adenosine triphosphate [ATP]-binding cassette subfamily B member 1 gene [ABCB1]) was discovered more than 30 years ago.56
15.4.2 Imaging Biomarker of Multidrug Transporter Function
Noninvasive brain imaging of multidrug efflux transporter function in pharmacoresistant and seizure-free epilepsy patients is a strategy to evaluate whether the overexpression of multidrug efflux transporters at the BBB as postulated in the transporter hypothesis has any functional consequences that underlie pharmacoresistance in epilepsy. Additionally, PET tracers for multidrug efflux transporters could be useful to identify epilepsy patients with increased multidrug efflux transporter activity who will benefit from treatment with multidrug efflux transporter modulation drugs and therefore hold great promise for individualized medicine.57
Almost all efforts to develop multidrug efflux transporters ligands have focused on Pgp expressed at the BBB or in tumors. To date only radiolabeled transporter substrates have been described to measure multidrug efflux transporters in vivo in humans.
15.4.3 [11C]verapamil
[11C]verapamil (VPM) is the best validated PET tracer to image Pgp function to date. (R)-[11C]verapamil is a high-affinity substrate of Pgp and is therefore very effectively transported by Pgp at the BBB. This results in low brain uptake of this radiotracer thus making it difficult to detect regional differences in cerebral Pgp function. A possible strategy to overcome the limitation of the low brain uptake of Pgp substrate radiotracer is to perform PET scans after partial blockade by Pgp modulating drugs such as cyclosporine A (CsA) or tariquidar (TQD).58 The third-generation Pgp inhibitor TQD is safer than the nonselective CsA for use in human subjects and was shown to lack interaction with metabolism and plasma protein binding of (R)-[11C]verapamil.59,60 Blocking Pgp with an inhibitor allows the radiotracer to enter the BBB and hence increase its uptake and signal in the brain.
The function of Pgp can be quantified in vivo from dynamic PET data using standard compartmental models. Pgp mediates movement of substrates, such as verapamil, from brain to plasma. Since we administer the Pgp substrate PET tracer [¹¹C]-verapamil intravenously, its entry into the brain is limited by Pgp activity, and the net effect is measured as K₁, the transport rate constant from plasma to brain; a low net influx from plasma to brain, indicated by a low K₁ value, therefore indicates high efflux from the brain and, thus, high Pgp activity. As shown in rodent models of Pgp overactivity,61 partial Pgp inhibition increases (R)-[¹¹C]verapamil K₁, but this increase is attenuated in areas of high P-glycoprotein activity, since a fixed dose of Pgp inhibitor (2 or 3 mg/kg) inhibits a lower proportion of binding sites in areas of high Pgp activity than in areas of low activity. Therefore partial Pgp inhibition should be used because if Pgp glycoprotein were fully inhibited, brain uptake of [¹¹C]-verapamil K₁ would be driven by passive diffusion only and differences in Pgp activity could not be detected.
Bartels et al.62 studied the Pgp function using [11C]-verapamil PET in 17 healthy volunteers aged 18–86. Analysis with statistical parametric mapping showed significantly decreased Pgp function in older subjects than in younger subjects in the internal capsule and corona radiata white matter and in orbitofrontal regions, thereby suggesting that Pgp function declines with increasing age. Furthermore, haplotypes of nucleotide polymorphisms at positions 1236, 2677, and 3435 of the MDR1 gene have been shown to alter Pgp activity in vivo and to alter substrate specificity in vitro. However, imaging studies with [11C]-verapamil show that pharmacokinetics were unaffected in healthy volunteers who expressed either the TTT or the CGC (wild-type) haplotype.63
The promising concept of performing (R)-[11C]-verapamil PET scans after blockade of Pgp was translated into healthy human subjects.60 Five healthy volunteers underwent paired (R)-[11C]verapamil PET scans before and after intravenous administration of TQD (2 mg/kg of body weight). TQD administration resulted in significant increases in K1, +49% ± 36% of (R)-[11C]verapamil across the BBB. The data from this first human study were reanalyzed region-wise using an automated atlas approach to define 43 different brain regions as well as parametric maps. No regional differences in TQD-induced Pgp inhibition were detected, suggesting that there were no regional differences in Pgp function in healthy human brain.64 Shortly after, Eyal and coworkers confirmed the results in a study with [11C]verapamil before and during infusion of CsA.65 Thereby K1 estimates were similar across gray-matter regions of the brain and the magnitude of Pgp inhibition was comparable across BBB-protected brain structures.
A pilot study used PET and the radiotracer (R)-[11C]-verapamil to test for differences in Pgp activity between epileptogenic and nonepileptogenic brain regions of patients with pharmacoresistant unilateral mTLE.66 In this study of five subjects, there was a trend toward asymmetric uptake of (R)-[11C]verapamil, favoring the temporal cortex and hippocampus ipsilateral to the main seizure focus. Using in vivo PET experiments with the Pgp-substrate (R)-[11C]verapamil (VPM), Feldmann et al. studied 14 pharmacoresistant mTLE patients and eight seizure-free mTLE patients due to unilateral hippocampal sclerosis (HS) as well as 13 healthy controls, testing the hypotheses that Pgp-activity is higher in pharmacoresistant than seizure-free mTLE patients and healthy controls, and that Pgp overactivity is most pronounced in the epileptic focus.67 Voxel-based analysis revealed that pharmacoresistant patients had lower baseline K₁, corresponding to higher baseline Pgp activity, than seizure-free patients in temporal lobe regions. Higher Pgp activity was associated with higher seizure frequency in whole-brain gray matter (p = .016) and the hippocampus (p = .029). Voxel-based comparison of globally normalized images revealed lower VPM-K1 in pharmacoresistant mTLE patients in temporal lobe regions compared to healthy controls. Voxel-based SPM analysis before and after TQD showed significant differences in increases of VPM-K1 between pharmacoresistant mTLE patients and healthy controls with the maximum difference in the ipsilateral hippocampus, suggesting that there is regionally specific Pgp overactivity for the epileptogenic hippocampus which is functionally relevant. Additionally, comparison of VPM-PET with epileptic tissues removed during epilepsy surgery, increases in VPM-K1 after TQD were less pronounced in three pharmacoresistant mTLE patients, suggesting relatively higher Pgp activity in the ipsilateral (sclerotic) hippocampus than the ipsilateral temporal neocortex.
In accordance with this observation, the same three pharmacoresistant mTLE patients also showed a higher percentage area of Pgp immunopositive labeling in the sclerotic hippocampus compared with the ipsilateral neocortex. In vivo PET measurements of Pgp activity correlated with ex vivo Pgp expression in the surgical temporal lobe specimens of those patients who had undergone surgery, in keeping with the hypothesis that there is localized Pgp overactivity in pharmacoresistant mTLE. Recently Bauer et al. examined seven patients with mTLE in a longitudinal study using VPM-PET before and after temporal lobe resections to assess whether postoperative changes in seizure frequency and AED load are associated with changes in Pgp function and correlated their in vivo VPM-PET measurements of Pgp function with ex vivo immunohistochemistry from surgical temporal lobe specimens.68 They found that pharmacoresistant mTLE patients who became seizure-free after surgery had lower VPM-K1 values, hence increased temporal lobe Pgp activity before surgery, increased Pgp expression in their surgically resected hippocampal specimens, and reduced global Pgp activity postoperatively, i.e., higher VPM-K1 values postoperatively, compared with patients who continued to have seizures postoperatively and had a poorer surgical outcome.
Genetic polymorphism of Pgp might play a role in pharmacoresistant mTLE. Only two imaging studies have been performed so far to investigate the role of Pgp and of its polymorphisms in pharmacoresistant mTLE. In a pilot study with seven patients with mTLE no relationship between the ABCB1 genotype and the (R)-[11C]verapamil efflux rate constant k2 could be described but the sample size was small.66 On the other hand a SPECT study in patients with epilepsy the 3435CC genotype was associated with reduced brain uptake of (99mTc)sestamibi, which was correlated with drug resistance.69 Furthermore, phenobarbital concentration in the cerebrospinal fluid and CSF/serum phenobarbital concentration ratio were significantly lower in patients with the CC genotype than in patients with CT or TT genotypes of the ABCB1 C3435T polymorphism. Additionally, the seizure frequency was also higher in CC homozygotes.70
Figure 15.2. Differential effects of Pgp inhibition on VPM-K1 in pharmacoresistant TLE patients and healthy controls (a) Tariquidar (TQD, 2–3 mg/kg) is least effective in increasing VPM uptake in the epileptogenic hippocampus (p < .05, small volume correction) in pharmacoresistant TLE patients (n = 14) compared to healthy controls (n = 13). At a lower level of significance (p < .05 uncorrected), an area of attenuated increase in VPM-K1 is seen in the ipsilateral superior temporal gyrus. (b) Increases in VPM uptake following 2–3 mg/kg TQD in pharmacoresistant TLE patients (PR, n = 14) are attenuated in the epileptogenic hippocampus compared to the contralateral side (left side = isilateral; right side = contralateral to seizure focus), showing saturation at the higher dose of 3 mg/kg in healthy volunteers (HV, n = 13). Patient 2, who became temporarily seizure-free and suffered from marked AED-specific side effects after TQD, is indicated by a red line. Immunolabeling of two regions (ipsilateral hippocampus (c) and superior temporal gyrus (d) from one of the five pharmacoresistant TLE patients who underwent resective surgery. (e) Negative correlation (Spearman r = −.900, p = .034) of the difference in percentage area of Pgp immunopositive labeling between the hippocampus (HC) and the superior temporal gyrus with the difference in percentage increase in VPM uptake after 2–3 mg/kg TQD between the same two regions in pharmacoresistant TLE patients who underwent resective surgery (n = 5).
15.4.4 [11C]-N-desmethyl-loperamide
The radiotracer [11C]-N-desmethyl-loperamide has the advantage that it is metabolized to a lesser extent than (R)-[11C]verapamil. The first human studies using PET with [11C]-N-desmethyl-loperamide at baseline71 and after Pgp inhibition with TQD72 have been recently carried out. At baseline there is virtually no uptake of this radiotracer in the brain. After 2 mg/kg of TQD the brain uptake of radioactivity increased only slightly (approximately 30%). In contrast, 4 and 6 mg/kg of TQD increased brain uptake two- and fourfold, respectively. Until now, [11C]-N-desmethyl-loperamide has not been used clinically to study disease in patients. On the other hand, [11C]-N-desmethyl-loperamide is a high affinity Pgp substrate that virtually shows no brain uptake, which makes it unsuitable to map regional differences in Pgp function at baseline.
15.4.5 Radiolabeled AEDs
It is also possible to label AEDs with a positron emitter. [11C]phenobarbital and [11C]phenytoin have been shown to be weak substrates for Pgp and are expected to have higher brain uptake than the high-affinity Pgp substrate [11C]verapamil.73 They could therefore be better suited to assess regional differences in Pgp function, in particular Pgp overexpression. A study in eight patients with pharmacoresistant focal epilepsy and two patients without epilepsy showed that [11C]phenytoin concentration ratios were lower in the visual cortex in epilepsy patients who had an average value of 1.32 (range 1.05–1.66) compared to 1.61 (1.34–1.87) in nonepileptic patients.74 Whether phenytoin concentrations are lower within the epileptic focus was not addressed in this study.
15.4.6 Other Radiolabeled Pgp Substrates
Several more radiolabeled drugs and radioligands have been investigated as PET tracers for Pgp. The radioligand [18F]MPPF has been developed as an alternative to short-lived (11C)-labeled tracers for PET studies of serotonin 5-HT1A receptors. [18F]-MPPF has been used in animal studies together with the third-generation Pgp inhibitor TQD to study Pgp activity. Preliminary results obtained in a clinical study with 10 mTLE patients showed that the Pgp inhibitor CsA significantly increased the [18F]MPPF binding potential (the ratio of receptor density and radioligand affinity) by 14% in most brain regions, regardless of their involvement in seizure generation or propagation.75
15.5 Conclusions
Neuroimaging, particularly fMRI, has demonstrated syndrome and regionally specific effects of distinct AEDs in epilepsy. So far, as a potentially common theme, AEDs appear to lead to attenuation of either task-related activation and/or deactivation in colocalized hubs critical to both the specific epilepsy syndrome and the network relevant to the cognitive function studied.
It has been argued that general conclusions on specific AED effects from the studies available are difficult to draw since effects are seen in the context of a specific task.53 However, others argue that evaluation of CNS drug effects are necessarily investigated with tasks as activation of specific brain networks defines the consequences of a drug effect on cognition and behavior and/or on disease processes. Activation patterns in the context of a task are stable and highly reproducible, and their alteration by disease and medication may therefore serve as distinct biomarkers.18 Translating from other areas of fMRI research, e.g., pain drug discovery, fMRI can be envisioned as a tool to integrate several aspects of CNS drug research, such as functional pathology due to the disease, the contribution of genes to both functional networks and drug response, and early surrogate markers of drug efficacy and side effects.18
Molecular imaging techniques have helped to investigate mechanisms of pharmacoresistance in epilepsy. In particular, PET has been used to evaluate the transporter hypothesis and demonstrated that Pgp is increased in pharmacoresistant epilepsy. Several open questions remain and molecular imaging techniques can help to enhance our understanding of underlying mechanisms for pharmacoresistance in epilepsy.
Using noninvasive imaging with PET enables us to identify individual patients where pharmacoresistance is caused by Pgp overactivity and potentially individualize treatment. Moreover, comparative studies between pharmacoresistant and seizure-free epilepsy patients can enable testing for a correlation between Pgp function and the pharmacoresponse. This imaging technique can also guide patient selection for future clinical studies. In particular, PET imaging of Pgp function may allow individualized application of approaches to overcome Pgp-associated pharmacoresistance. In the future combined imaging and clinical trials of novel treatment strategies, such as Pgp inhibitors or modulators of overexpression in patients who have Pgp overactivity on VPM-PET could be employed. Targeting Pgp by modulators can enhance the efficacy of AEDs. The compound verapamil is a substrate for Pgp at low concentrations (which is used in PET), but, like many substrates, verapamil is also an inhibitor for Pgp at high concentrations.76 Verapamil was among the first identified inhibitors of Pgp and may function to block Pgp–modulated efflux of AEDs in the brain, thereby raising the intracellular concentration of AEDs.77 The third-generation modulator TQD increased the efficacy of phenytoin in a chronic rat epilepsy model and helped to overcome pharmacoresistance to phenobarbital in chronic rat epilepsy models.78 Third-generation inhibitors are considered fairly specific. But there is recent evidence for the third-generation inhibitor TQD that it can also affect the efflux transporter BCRP/ABCG2. Moreover, long-term inhibition of this transporter needs to take into account that Pgp serves as a protective mechanism and gatekeeper in several blood tissue barriers as well as hematopoietic cells.79 In addition to limiting access of harmful xenobiotics to sensitive tissues or cells, Pgp also accelerates extrusion of xenobiotics based on its efflux function in the liver and kidneys. Therefore, alternate approaches that leave basal transporter expression and function unaffected might offer advantages for tolerability and safety issues. Preventing seizure-associated transporter upregulation might offer an intriguing alternate approach to overcoming transporter associated pharmacoresistance.80,81
Although recent studies provide the proof of concept for the transporter hypothesis and show that there is functionally relevant Pgp overactivity in pharmacoresistant mTLE,67 the critical question remains whether it is sufficient to overcome Pgp overexpression as one putative mechanism of a multifactorial problem or whether other mechanisms such as intrinsic disease severity, alterations in targets, different gene variants, or network alterations need to be taken into account and further investigated in the future. Given the complexity of epilepsy, it is unlikely that pharmacoresistant epilepsy is caused by a single mechanism but instead is due to several mechanisms that may even occur in the same patient. Overcoming pharmacoresistance in epilepsy represents a challenge and will necessitate a multifactorial approach and the combined efforts of basic and clinical epilepsy researchers.54
References











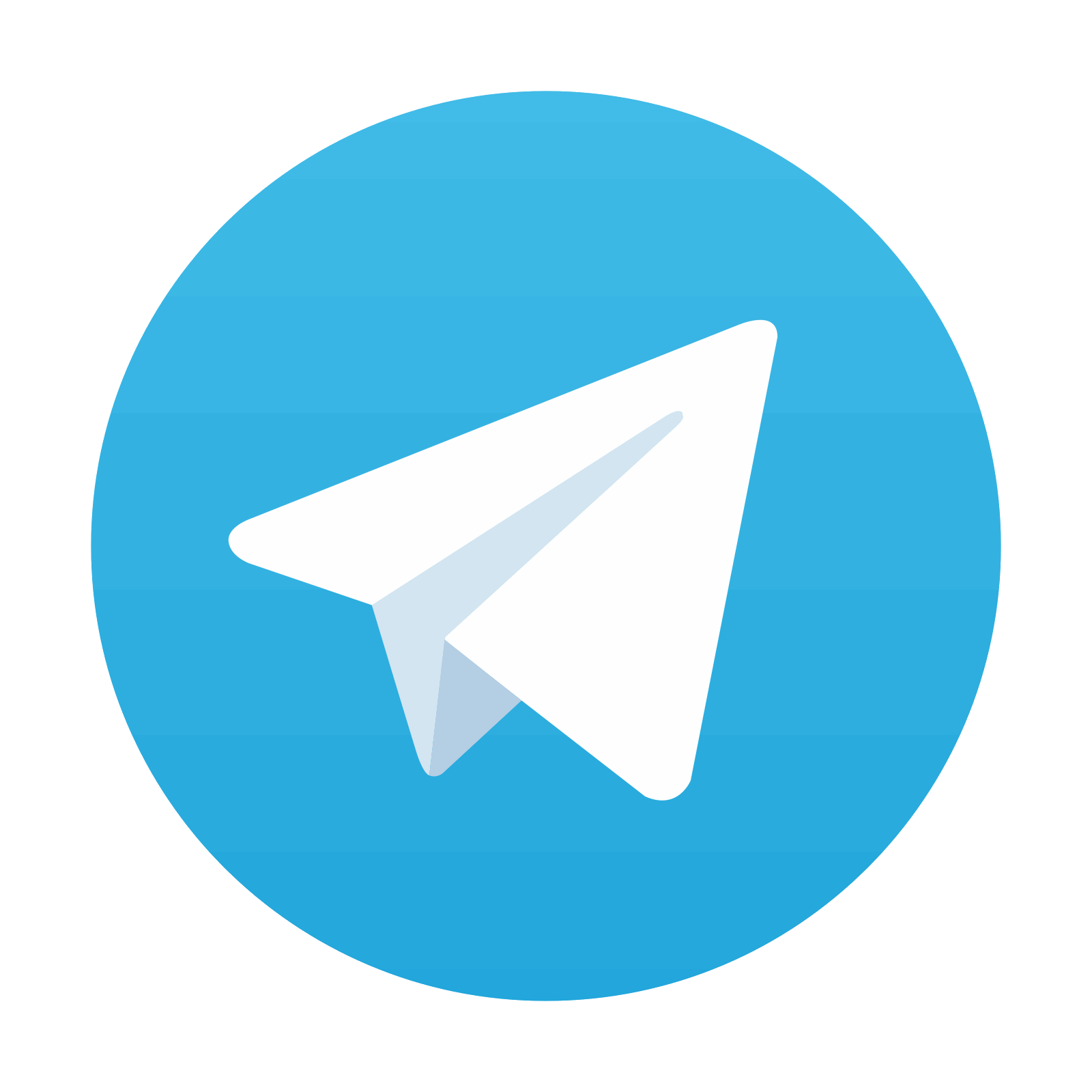
Stay updated, free articles. Join our Telegram channel

Full access? Get Clinical Tree
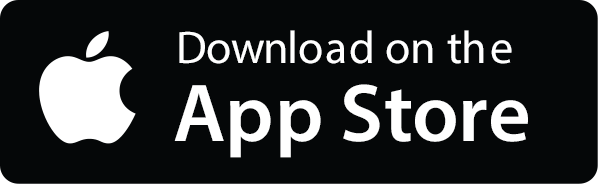
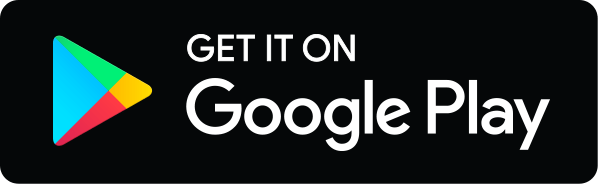
