21.1 Introduction
Cognitive impairment is a frequent comorbidity in epilepsy and has a major impact on quality of life in these patients. Cognitive deficits either can result from the underlying disease as a consequence of seizures or interictal epileptic activity, or can be caused by adverse effects of antiepileptic drugs (AEDs).1
In temporal lobe epilepsy (TLE), as the most common type of focal epilepsy, typically material-specific memory impairment and naming difficulties have been reported.2 Even in some patients with epilepsy of recent onset3 cognitive impairment can be observed, which supports the hypothesis that cognitive problems cannot fully be explained by adverse effects of medication. In general, patients who are refractory to AED treatment are at higher risk of suffering from cognitive impairment.
In patients with medically refractory TLE, anterior temporal lobe resection (ATLR) is an effective and safe treatment option, leading to seizure remission in up to 60–70% of these patients.4,5 Unfortunately this procedure may be complicated by a decline in language and memory abilities. Therefore an important emphasis during presurgical evaluation is to identify the epileptic brain tissue that has to be removed for the patient to become seizure-free, and to predict the cognitive cost of this. Neuropsychological deficits must be avoided, which requires accurate localization of the brain areas that are responsible for motor, language, and memory functions (eloquent cortex).
In recent years, epilepsy surgery has been carried out earlier in the course of the disease, and so the potential benefits must be carefully weighed against the potential risks of decline. In order to successfully identify eloquent brain areas and guide resection, a range of imaging techniques such as functional MRI (fMRI) and diffusion tensor imaging (DTI) have been employed.
In particular fMRI has proven a valid and reliable tool to investigate cognitive functions during presurgical assessment of patients with focal epilepsy, and has increasingly replaced invasive techniques such as the intracarotid amytal test (IAT) as it is noninvasive, cheaper, and repeatable. Compared to standard neuropsychological assessment, it has the potential to provide additional information about the lateralization and localization of language and memory function and specifically allows evaluation of functional reorganization processes (over time). There is an increasing interest in its possible role for identification of the eloquent cortex and prediction of postoperative cognitive changes.
21.2 Methodological Considerations and Limitations of Cognitive fMRI
In the past, invasive methods have been routinely used to identify the eloquent cortex: the IAT was widely used to lateralize function, and electrocortical stimulation mapping (ESM) is still the gold standard to localize eloquent cortex. In recent years, however, noninvasive methods, predominantly fMRI, have been established for this purpose.6, 7 Obvious advantages of noninvasive methods are patient safety and tolerability. In addition they can be applied in healthy volunteers for research purposes allowing systematic comparison of different study groups. There are, however, some methodological aspects that need to be considered.
Functional MRI is an activation-based method. The rationale behind this is that if a certain cognitive function is used, relevant brain areas will be activated. Blood flow will increase in these areas to compensate for the higher demand of oxygen. In fMRI, this is displayed by the BOLD contrast, which represents the regional changes in blood flow over time. To be able to get a reasonable temporal resolution echo planar imaging (EPI), a fast MRI sequence, is used. Functional MRI has ahigh spatial resolution which, in principal, allows very good localization of areas in the brain that are involved in certain tasks. However, which areas will be activated depends on the fMRI paradigm. It is important that there is sufficient task performance, as only then can activation be interpreted in relation to function. A differential pattern of activation between patients and normal subjects is interpretable only if patients are performing the task adequately.8 This needs to be considered in fMRI paradigm design, especially when applying fMRI to patient groups in which cognitive performance can vary considerably. It is mandatory to make sure that participants understand and follow task instructions. However, during fMRI the subject’s performance cannot easily be assessed. The majority of fMRI paradigms applied to date use covert tasks or assess performance with a joystick or button press response. This can restrict the variety of possible responses that can be used in an fMRI paradigm. More recently developed devices such as MRI-compatible microphones can now be used to monitor speech during fMRI. For simple paradigms that require only basic analysis, e.g., as used for clinical language fMRI, scanner implemented software for online data analysis can be employed to show activation patterns during the scan.
One must be aware of differences in the questions being asked by cognitive neuroscientists and clinicians, which can lead to different approaches to data analysis. By looking at groups of matched controls, or patients and controls, performing the same task, neuroscientists aim to determine which brain regions are commonly activated across the group. The emphasis is on avoiding false positive results (Type I errors), which requires conservative statistical thresholds, which in general may lead to an underrepresentation of brain areas truly involved. Clinicians on the other hand are considering individual patients where the priority is to identify all brain regions involved in a task, and therefore aim to avoid false negatives (Type II errors). Less stringent statistical thresholds are required and thresholds used may need to vary on an individual basis.9
Most cognitive tasks do not rely on only one particular function. To differentiate between activation related to a certain function and other activation, neuroanatomical information (e.g., Broca’s area, Wernicke’s area for language, medial temporal lobe structures for memory) may be used to interpret results. Most fMRI paradigms include other tasks with the aim to control for irrelevant activations; only activation in the “real” task that exceeds activations during the control task is considered. The specific brain areas that are displayed as “active” vary substantially with different statistical thresholds. A high threshold reduces the sensitivity in detecting activations as well as the extent of activation clusters while a low threshold increases the risk of false-positive activations.
In the specific case of epilepsy, seizures and interictal epileptic activity as well as AEDs may have an effect on state or interaction between different brain regions and therefore additionally alter fMRI results.10
In summary, caution is needed in the interpretation of the results. First, areas activated by a particular fMRI paradigm are not necessarily essential for performing a task. Second, not necessarily all areas involved in a task will be activated by one particular fMRI paradigm; in addition the region of maximal BOLD activation does not always pinpoint the most eloquent cortical regions, and areas that do not surpass the chosen threshold are not necessarily functionally inert. Finally areas of BOLD activation vary greatly in size depending on thresholds used for display, while extent and magnitude of activation seen in a task do not necessarily relate to the competence with which the task is performed.11
21.3 Language Functions
21.3.1 Assessing Language Functions with fMRI
Functional MRI can map language networks in patients with epilepsy for research and clinical purposes. During evaluation for epilepsy surgery, fMRI is the most frequently applied noninvasive method for language imaging.7 The primary aims of preoperative language fMRI are lateralization and localization of language functions and to use this information to predict postoperative complications and advise patients accordingly. Clinically applied paradigms focus on classic language areas such as Broca’s area (inferior frontal gyrus [IFG]) and Wernicke’s area (supramarginal gyrus [SMG], superior temporal gyrus [STG]). Covert lexical word generation (i.e., thinking of words starting with a given letter) is an expressive language task that reliably activates the IFG (Figure 21.1), corresponding to Brodmann’s areas 44 and 45. Fluency tasks are usually less reliable at identifying receptive language areas in the dominant temporal lobe. Posterior temporal lobe activation (SMG, STG), corresponding to Brodmann’s areas 20, 21, and 39, can be achieved by more receptive tasks, e.g., semantic decision tasks (e.g., “Which word does not match the others: shirt, gloves, shoes, rose?”) or story listening tasks. Also reading tasks usually activate the superior temporal cortex extending to the SMG; these “receptive” tasks are less strongly lateralizing than verbal fluency tasks.
Figure 21.1. Language fMRI activations during covert lexical fluency. Language fMRI activations during covert lexical fluency in a single subject at p = .05, family-wise error. Strong activation can be seen in the left IFG, as well as in the left MFG and SFG.
Most of the time, these tasks are performed without performance monitoring in the scanner (covertly); however, numerous studies have replicated these activations in both healthy control subjects and patient populations. Within-subject reproducibility has also been demonstrated, although frontal activations have been replicated more reliably compared to temporoparietal activations.12
For the clinical application it is essential that subjects are able to perform the tasks and that these language tasks can reliably be applied to patients with a wide range of cognitive abilities. Verbal fluency and verb generation tasks not only provide us with usual language related activations, but also require executive processing and involvement of verbal and working memory, resulting in activations in the middle frontal gyrus (MFG) corresponding to Brodmann areas 46 and 49.
Most language paradigms are presented as blocked designs in order to detect regions of the brain showing greater activation during a task compared with rest. The major advantage of blocked designs is that they very reliably detect differences between two conditions (task vs. rest); however, they often lack sensitivity in detecting activation when studying more complex cognitive tasks (e.g., memory tasks).
As a measure of laterality, a so-called Laterality index is calculated by quantifying the degree of asymmetry: (A) = (L – R) / (L + R). L and R represent the strength of activation for the left (L) and right (R) sides, respectively, based on the number of activated voxels for the whole hemisphere or using regions of interest (ROIs) targeted to known language areas. As these methods are very much dependent on the various thresholds chosen, more recent methods are based on calculation of an overall weighted bootstrapped lateralization index, taking thresholds into account.13,14
21.3.2 Language fMRI in Epilepsy
Numerous different language paradigms have been applied to group studies, which may partly explain some differences in the results between studies.7,15 Many studies comparing language fMRI with the classic invasive methods in particular the IAT showed that fMRI is a valid method for identifying the language-dominant hemisphere,15–17 with an overall concordance of 90% between the two techniques. Functional MRI seems more likely to elicit bilateral language representation compared to the IAT; however, the meaning of this finding for language function after surgery is not fully understood.17 Concordance between fMRI and the IAT is the highest for right TLE patients with left language dominance and for frontal language areas, and the lowest for left TLE patients with left language dominance.18 Atypical language dominance on fMRI17 and interhemispheric language dissociation19 are correlated with IAT/fMRI discordance. This suggests that fMRI may be more sensitive than the IAT or cortical stimulation to map the whole network involved in language processing, but is less specific at an individual level as compared to cortical stimulation.
From a clinical perspective in patients with focal epilepsy it is advisable to use a combination of expressive and receptive fMRI tasks (e.g., verbal fluency, reading comprehension, auditory comprehension) to reduce interrater variability and help to evaluate language laterality.20 Of members of the healthy population, 90% have left-sided language representation, while the remaining 10% show atypical (i.e., bilateral, right-hemispheric) language representation. Patients with epilepsy have a significantly higher incidence of atypical language representation (16% bilateral, 6% right-lateralized, 78% left-lateralized), which is additionally influenced by variables such as earlier age of epilepsy onset and the underlying pathology.21 It has been speculated that an early insult to the originally language-dominant hemisphere may lead to reorganization of language function to homotopic regions in the contralateral hemisphere. At the same time it is not obligatory that lateralization for different language functions (i.e., receptive and expressive) within one patient is the same (“crossed” language lateralization).22, 23 Temporal lobe foci have wide-ranging effects on the distributed language system, and TLE patients are more likely to have atypical language representation in Wernicke’s area compared with a frontal focus, while the effects of a frontal lobe focus appear restricted to anterior rather than posterior language processing areas.24 There is an increase and posterior shift of language related activation in the right inferior frontal gyrus after left hemisphere injury. However, activations in the left inferior frontal gyrus remain in the same location.25 There is also evidence that the hippocampus itself may be an important factor for establishing language dominance as patients with left hippocampal sclerosis compared to patients with left frontal and lateral temporal lesions had a higher incidence of atypical language representation.26 Language areas activated for abstract and concrete words are also different for TLE patients, with or without HS, suggesting that HS is associated with altered functional organization of cortical networks involved in lexical and semantic processing.27 Significant correlations between fMRI activation in the left hippocampus during basic language tasks and out of scanner naming performance have been shown in controls and patients with right TLE, while in left TLE patients naming function was reallocated to the left middle frontal gyrus, supporting the hypothesis that naming ability depends on the integrity of the hippocampus and connecting frontotemporal networks.28, 29 This also supports observations that surgical removal of the dominant hippocampus in patients with left TLE may cause language alterations, mainly naming deficits. In pediatric patients with lesions in close proximity to Broca’s area, expressive language function could be demonstrated in the perilesional cortex.30 In summary, apart from age at disease onset, other factors such as an underlying structural lesion, neuroanatomical substrates, as well as the underlying pathology of the lesion seem to contribute to the reorganization process of language functions, which needs to be taken into account for the clinical interpretation of such data.31
In patients with left TLE, Janszky and colleagues found that atypical language representation was associated with increased interictal epileptic discharges,32 which suggests that fMRI might also be suitable for investigation of dynamic changes in cognitive networks.33 AEDs can affect cognitive processing.34 Topiramate has an effect on activation in the basal ganglia, anterior cingulate, and posterior visual cortex and can cause reduced deactivation of the default mode network-related areas during a language task suggesting interference in cognitive processing by topiramate.35, 36
There is increasing evidence that pre- and postoperatively reorganization of language function can occur to the contralateral but also within the ipsilateral hemisphere.37 Atypical language lateralization to the right hemisphere may shift back to the left hemisphere in seizure-free patients after left selective amygdalo-hippocampectomy.38 In a large longitudinal study it has been shown that four months following ATLR, left TLE patients demonstrated greater bilateral fMRI activation during a verbal fluency task compared to preoperatively, suggesting an early postoperative reorganization of language function to the contralateral hemisphere, with evidence of multiple systems supporting language function. Right TLE patients on the contrary showed no postoperative change in language activation patterns.29 These findings were corroborated by functional connectivity analysis showing greater postoperative than preoperative connectivity to the contralateral frontal lobe in patients with left TLE, while there was no postoperative change in functional connectivity in right TLE.29
In terms of efficiency of this postoperative reorganization, left TLE patients without significant naming decline after left temporal lobe surgery showed a significant correlation between postoperative language fMRI activation and postoperative naming performance in the posterior remnant of the left hippocampus, while patients with a significant naming decline demonstrated a correlation in homotopic language areas (MFG) of the contralateral hemisphere,29 emphasizing the importance of ipsilateral reorganization even within the damaged hippocampus for maintaining naming function. Similarly, Wong and colleagues showed that after ATLR left TLE patients showed reduced activation in the left MFG and right IFG, whereas no difference was observed in the right TLE patients.39
Language fMRI may, however, be useful in patients other than those who are undergoing presurgical evaluation. In patients with generalized epilepsy, fMRI showed that language function was impaired as represented by reduced suppression of the default mode network, an inadequate suppression of activation in the left anterior temporal lobe and the posterior cingulate cortex, and an aberrant activation in the right hippocampal formation.40 In primary reading epilepsy, a study combining EEG and fMRI showed specific regions that were involved in seizure generation during reading.41 Language fMRI has also been used to study language networks in different epilepsy syndromes, showing that patients suffering from benign epilepsy were less likely to have left lateralized language representation compared with healthy controls.26 Children with benign epilepsy with centrotemporal spikes (BECTS) and other epilepsy types also showed bihemispheric language networks, which may represent a compensatory response for ongoing epileptic activity in the brain.42, 43 Also, anterior language networks are affected more in BECTS, resulting in language difficulties for functions dependent on the integrity of anterior language regions, e.g., sentence production.44 Brain activation patterns can be assessed repeatedly during language development in certain epilepsies, which may provide prognostic information regarding potential language achievement in relation to seizures and help finding new rehabilitation strategies.41, 45
21.3.3 Localization of Language Function and Prediction of Postoperative Deficits
Aphasia is rare after epilepsy surgery, but more subtle language decline such as word finding and naming difficulties has been reported in up to 50% of patients after ATLR of the language-dominant hemisphere.46 There is evidence that the risk for postoperative naming decline increases with both age of seizure onset and the extent of the resection of the lateral temporal neocortex.47 A recent fMRI study investigated the impact of focal epilepsy on the developing language system. In 21 children with focal epilepsy and left language dominance the authors demonstrated decreased activation of the ventral language network (compared to healthy controls), which was associated with poorer language outcome. The authors concluded that childhood-onset epilepsy preferentially alters the maturation of the ventral language system, and that this was related to poorer language ability.48 Using a semantic decision paradigm Sabsevitz and colleagues demonstrated that greater left- than right-sided activation in particular in the temporal structures was associated with a significantly higher risk for postoperative naming deficits.49 In addition, in left TLE, a predictive value of fMRI language lateralization for verbal memory decline50, 51 could be shown, emphasizing a link between the inferior frontal cortex and the hippocampus. Using a simple verbal fluency task in left TLE patients preoperative left middle frontal activation was predictive of clinically significant postoperative naming decline, with high sensitivity and relatively lower specificity.29 However, prediction models for specific language tasks on an individual level are not yet sufficient to be applied in clinical routine. Future studies will need to investigate whether specific language paradigms, particularly auditory and visual naming paradigms, will allow more accurate prediction in individual patients.
Compared to lateralization, accurate localization of language areas with fMRI is not yet established. First of all, test-retest series have shown that the localization of areas that were activated during a specific language fMRI task was less reliable than lateralization.12 Furthermore, ESM studies showed only imperfect overlap with activation clusters of fMRI: in some cases electric stimulation of fMRI activated brain areas did not result in language disturbances,52 while in others crucial areas were not displayed during fMRI.53 The differences may be related to either the applied language paradigms or the statistical thresholds. Rutten and coworkers developed an fMRI imaging protocol that involved four different language tasks for the intraoperative localization of critical language areas. The authors suggested that retrospectively, regions where no fMRI activation was present during four different language tasks (verbal fluency, word finding, naming, sentence comprehension) could have safely been removed without performing ESM.54
To date, language fMRI localization is not suitable for resection decision but may be helpful in planning electrode placement for ESM.6
21.4 Memory Functions
Memory impairment is common in patients with epilepsy. Working and long-term memory (autobiographical, verbal, visual memory) may become affected, in a material specific way, based on the site of lesion. Functional MRI can reveal memory networks noninvasively and reliably, and also the effect of surgery on these networks.
21.4.1 Autobiographical Memory
This network including the hippocampus, the medial prefrontal cortex, temporal poles, the retro-splenial, and lateral parietal cortex showed reduced activation in patients with left HS and transient epileptic amnesia.55, 56 The connectivity of a sclerosed left hippocampus was also reduced, whereas connections between extrahippocampal nodes were increased.55, 56 In patients with transient epileptic amnesia, there was reduced activation of the right hemisphere, more specifically of the posterior parahippocampal gyrus, the temporoparietal junction, and the cerebellum, for midlife and recent memories.55, 56 In addition, there was reduced effective connectivity between the right posterior parahippocampal gyrus and the right middle temporal gyrus.56 These findings suggest that there is functional reorganization of the neural network supporting autobiographical memory retrieval in patients with TLE and transient epileptic amnesia.55, 56
21.4.2 Material Specificity and Episodic Memory
A cognitive process that enables the explicit recollection of unique events and the context in which they occurred,57 including the transformation of an experience into an enduring memory trace (memory encoding) and the subsequent recollection of this event at a later time (memory retrieval), is defined as episodic memory. Several brain regions including the medial temporal lobe (MTL) and prefrontal cortices are involved in these processes. The MTL consists of the hippocampus, the amygdala, and the parahippocampal regions and is strongly associated with memory function. TLE and ATLRs are both associated with reduced memory function. Initial lesion studies have provided evidence that the hippocampi play a crucial role in memory functioning.58 While bilateral injury to these areas leads to a characteristic amnesic syndrome59 patients with unilateral TLE often present with memory impairment, which is specific to certain materials (e.g., verbal and visual). After temporal lobe surgery to the language-dominant hemisphere more often verbal memory decline can be observed,60 while temporal lobe surgery in the nondominant hemisphere is more likely to result in visuospatial memory decline.61 Sustained anterograde amnesic syndrome after unilateral ATLR, however, is rare. Most of these patients have subsequently been found to have evidence of contralateral hippocampal pathology, on postoperative EEG,62 postmortem pathological findings,63 or postoperative volumetric MRI.64
Many fMRI studies have demonstrated material-specific lateralization of memory function in prefrontal but also medial-temporal regions.65–68 For clinical purposes, paradigms are usually applied that show bilateral MTL activation in healthy controls.69, 70 Previous fMRI studies in patients reported reduced activation in the temporal lobe ipsilateral to the seizure onset.66, 67, 71, 72 These results were comparable with the Wada test.67 Most of these studies employed blocked analyses and showed more posterior hippocampal activations. More recent studies using event-related analyses showed material-specific lateralization of memory function in more anterior hippocampal regions during successful memory encoding68 and therefore in an area that is most likely to be resected during standard ATLR (Figure 21.2). The reduced activation within the affected temporal lobe and increased contralateral MTL activation during memory fMRI have provided further evidence of reorganization of memory function in TLE.70, 72 It is a matter of debate if reorganization toward the healthy hemisphere is effective, and whether it may be protective for memory decline after surgery. By correlation of fMRI activation and performance on standard neuropsychological memory tests, it has been shown that higher MTL activation ipsilateral to the pathology was associated with better memory performance while contralateral, compensatory activation correlated with poorer performance.70 Functional reorganization of networks involving extratemporal and temporal structures for verbal, visual, and non-material-specific memory encoding suggests compensatory mechanisms to mitigate the failure of the sclerosed hippocampus.73–75 In one study that investigated patients with left and right TLE, higher MTL activation was observed in the healthy temporal lobe. However, better verbal memory was still related to left MTL activation.76 This explains why good verbal memory is a risk factor for postoperative decline.
Figure 21.2. Material specificity of verbal and visual memory in healthy controls. Main effects of verbal and visual memory fMRI. (A) Word encoding—left hippocampal activation. (B) Face encoding—right hippocampal activation. Threshold p < .01, uncorrected. Significant regions are superimposed onto an averaged normalized mean EP image from 30 healthy controls.
21.4.3 Prediction of Postoperative Memory Changes
In two-thirds of patients with medically refractory TLE, an ATLR leads to seizure freedom, but may be complicated by memory impairment, typically verbal memory decline following left, and visual memory decline following right ATLR. Investigation of patients’ ability to sustain memory is critical for planning an ATLR as memory decline is not an inevitable consequence of temporal lobe surgery. One of the ultimate goals of clinical neuroimaging is to accurately predict likelihood and severity of postoperative memory decline in order to make an informed decision regarding surgical treatment.
Two different models of hippocampal function have been proposed to explain memory deficits following unilateral ATLR, the hippocampal reserve model, and the functional adequacy theory.77 According to the hippocampal reserve model, postoperative memory decline depends on the capacity or reserve of the contralateral hippocampus to support memory following surgery, while the functional adequacy model suggests that it is the capacity of the hippocampus that is to be resected that determines whether changes in memory function will be observed.
Over recent years many studies have focused on the identification of prognostic indicators for risk of memory loss after ATLR. The severity of hippocampal sclerosis on MRI turned out to be an important predictor, being inversely correlated with a decline in verbal memory following left ATLR, with less severe HS increasing the risk of memory decline.78 Another recognized prognostic factor for memory decline after ATLR was preoperative performance on neuropsychological tests, with higher preoperative scores indicating a greater risk for postoperative decline.79, 80 Language lateralization assessed by the IAT or more recently language fMRI has been found helpful to predict memory outcome.81, 82 These risk factors reflect the functional integrity of the resected temporal lobe and suggest that patients with residual memory function in the pathological hippocampus are at greater risk of memory impairment after ATLR (supporting the functional adequacy theory of the ipsilateral MTL, rather than the functional reserve of the contralateral MTL).
Other epilepsy-related factors such as age of epilepsy onset and duration of epilepsy83 have also been identified as useful predictors of postoperative outcome.
Memory fMRI has also shown to be a potential predictor of postoperative memory decline after ATLR.
Several small studies have investigated the predictive value of fMRI for verbal memory decline.82, 84–86 Only a few fMRI studies have investigated visual memory after ATLR.71, 86, 87 In patients with left HS, greater verbal memory encoding activity in the left hippocampus prior to surgery predicted the extent of verbal memory decline following left ATLR.72, 84, 86 These findings have since been replicated and extended to patients undergoing right ATLR.65, 86 A recent study in patients with left and right TLE demonstrated that preoperative recruitment of more extensive networks, mainly in extratemporal regions, during memory encoding was associated with better memory outcomes.75 Other groups employed asymmetry indices to account for contralateral hippocampal activation and demonstrated that relatively higher activation in the ipsilateral hippocampus was associated with greater memory decline.71, 87 Using a complex visual scene-encoding paradigm, Binder and colleagues also demonstrated asymmetric MTL activation in TLE patients, which was not predictive for postoperative verbal memory decline.50 A recent fMRI study combined mediotemporal activation asymmetry during a memory task with preoperative verbal memory scores and the information regarding the laterality of the epileptogenic focus. Greater ipsi- than contralateral fMRI activation was associated with a greater decline of verbal memory function. Using all the variables provided, postoperative verbal memory deficits could be predicted in 90% of all patients in this study.88 Material-specific activation paradigms are particularly attractive for prediction of material-specific memory deficits. Stronger left anterior hippocampal activation on word encoding was predictive of a more severe postoperative decline in verbal memory after left-sided temporal lobe resection (Figure 21.3), and stronger right anterior hippocampal activation on face-encoding predicted a more marked decline in visual memory function after right-sided resection. In addition, patients with relatively higher ipsi- than contralateral posterior hippocampal activation had the best postoperative verbal or visual memory outcome. These findings suggested that reorganization of function to the posterior ipsilateral hippocampus was associated with preservation of memory after ATLR. In this study, preoperative memory fMRI was also the strongest predictor of postoperative verbal and visual memory decline compared to other epilepsy-related variables. Symmetry of fMRI activation with verbal memory encoding, lateralization of language function, and performance on preoperative neuropsychological tests predicted clinically significant verbal memory decline in all patients who underwent left ATLR, but were less predictive of visual memory decline after right ATLR.65
Figure 21.3. Prediction of verbal memory decline using memory fMRI. Left anterior hippocampal activation for encoding words is associated with change in verbal learning scores after left ATLR, characterized by greater verbal memory decline in subjects with greater fMRI activation. Threshold p < .01, uncorrected. The correlation at the peak voxel is illustrated on the right. Significant regions are superimposed onto an averaged normalized mean EP image from 30 healthy controls, 15 patients with left and 15 patients with right hippocampal sclerosis.
Taking these results further, Sidhu et al. demonstrated that predominantly left-sided activations within the frontal and medial temporal lobes correlated with significant verbal memory decline after left ATLR. They found a strong correlation between a memory LI using a mask in the left frontal and medial temporal lobes and regression-adjusted change in ability to learn a supra-span word list over serial presentations.89 With this fMRI method they demonstrated involvement of frontal networks in verbal learning processes that appear useful in predicting postsurgical memory change emphasizing the role of the networks involved in cognitive processes rather than single structures.
21.4.4 Working Memory
Working memory is also affected in TLE patients with hippocampal sclerosis. Compared to healthy controls there was reduced right superior parietal lobe activity in patients with TLE and hippocampal activity from the healthy hippocampus was progressively suppressed as the working memory load increased, with maintenance of good performance in patients with TLE.90 Stronger functional connectivity between the superior parietal lobe (BOLD activation) and the sclerosed hippocampus (BOLD deactivation) was associated with worse performance, suggesting that the segregation of the task-positive and task-negative functional network was disrupted resulting in working memory dysfunction in TLE.90, 91 Patients with frontal lobe epilepsy (FLE) recruited more widely distributed networks for working memory encoding than did controls; particularly activation of the frontal lobe contralateral to the seizure focus was associated with better performance, suggesting an effective compensatory response of the brain to maintain memory function.92 Moreover, pediatric patients with FLE showed decreased frontal lobe connectivity, which was associated with cognitive impairment, despite intact fMRI activation patterns for working memory. This decreased frontal lobe connectivity may explain the cognitive problems encountered in children with FLE.93 In addition, high numbers of secondary generalized seizures may induce functional reorganization of working-memory-related networks, e.g., increased activation and reduced functional connectivity of the prefrontal cortex explaining working memory dysfunction in patients with focal epilepsy.94, 95
21.4.5 Postoperative Reorganization of Memory Function
Functional MRI may also be used to investigate the extent to which the brain may functionally reorganize following epilepsy surgery. Functional reorganization—both before and after surgery—can occur within the unaffected ipsilateral or contralateral hemisphere, but under which circumstances reorganization becomes effective is still poorly understood.
There is increasing evidence that in TLE patients, memory outcome depends on the extent of the removal of nonlesional functional tissues.96–99 To date, there are only a few fMRI studies in which memory function was systematically investigated before and after surgery. Cheung and coworkers assessed postoperative memory function using a complex visual scene encoding task. In their study postoperative memory function was significantly associated with functional activation contralateral to the side of resection in nine left and eight right TLE patients; therefore the authors suggested a role for the contralateral medial temporal lobe in supporting postoperative memory.100 Using a material-specific memory encoding task, Bonelli et al. showed that preoperative memory reorganization to the posterior part of the hippocampus was important to preserve postoperative verbal memory function after left ATLR. Conversely, if reorganization to the ipsilesional posterior remnant of the hippocampus occurred early (within 4 months) after surgery, this additional, early postoperative reorganization worked inefficiently. No significant visual memory reorganization was seen after right ATLR, providing evidence that verbal and visual memory function was affected differently by left and right ATLR.101 One study examined extratemporal network plasticity after surgery in TLE patients in a ROI in the IFG. Pre- and postoperative signal change within this ROI was compared quantitatively in this word and face classification study. Reduced right frontal activation was shown postoperatively during word encoding in left TLE suggesting a more left lateralized network postoperatively. No differences were seen for visual encoding.102
21.5 Functional MRI in the Temporal Lobes—Technical Challenges
Echo planar imaging is the sequence most commonly used for fMRI studies, as these require images to be acquired at high speed. Memory fMRI in the medial temporal lobe is in particular challenging due to limits on resolution and the possibility of geometric distortions and signal drop out caused by susceptibility effects associated with the use of EPI.103 Inhomogeneities in the magnetic field occur due to the different magnetic properties of bone, tissue, and air, as soon as the head is introduced into the scanner. Brain regions close to borders between sinuses and brain or bone and brain are most affected and therefore most likely to suffer geometric distortions or loss of BOLD signal. The occurrence of material specific memory impairment after ATLR indicates the major relevance of these areas for successful memory function. Intracranial electrophysiological recordings during verbal encoding tasks have shown greater responses in anterior hippocampal and parahippocampal regions for words remembered than those forgotten.104 At first, these results could not be replicated with functional imaging studies, with many showing encoding related activations in posterior hippocampal and parahippocampal regions, which would be left intact following ATLRs. One possible explanation for this apparent conflict is that anterior temporal regions are subject to signal loss during fMRI sequences, which is most prominent in the inferior frontal and inferior lateral temporal regions.105 Signal loss leading to sensitivity loss is unrecoverable by image-processing techniques and therefore may be more serious.9 The anatomical position of the hippocampus that rises from anterior to posterior may explain greater susceptibility-induced signal loss in the anterior (inferior) relative to the posterior (superior) hippocampus, which may have been one reason for the relative lack of anterior hippocampal activation in early fMRI studies of memory. One study demonstrated that there was a differential effect of susceptibility artifacts on the activation in the anterior versus the posterior hippocampus. They showed that the averaged resting voxel intensity in an anterior hippocampal ROI was significantly less than in a posterior hippocampal ROI; intensity decreases were substantial enough to leave many voxels below the threshold at which BOLD effects could be detected.106 Even more though, in a different study it could be demonstrated that the sensitivity to BOLD changes was proportional to signal intensity at rest so that voxels with a lower baseline signal (such as those in anterior hippocampal regions) would be more difficult to activate than those with higher baseline signals.107 Shimming, which is a process whereby the static magnetic field is made more homogeneous over the ROI, has been shown to be a useful method to correct some of these artifacts.
Paradigm design may be an alternative explanation for the lack of anterior hippocampal activation seen in many early memory fMRI experiments. Most of the paradigms used were not optimized for detecting subsequent memory effects as, for example, most of these early studies used blocked experimental designs. Studying memory function in general requires more complex paradigms and ways of analysis, as there are several different components involved in memory processing (encoding and retrieval) and as also the nature of the material being encoded or retrieved influences which brain areas are activated.
By using blocked design paradigms we are looking for regions of the brain showing greater activation during task blocks compared with rest blocks, which makes it difficult to separate brain activity due specifically to memory from that due to other cognitive processes involved in the task. To overcome this problem, event-related designs were introduced in recent fMRI memory studies.9
In brief, the detection of transient hemodynamic responses to brief stimuli or tasks is defined as event-related fMRI. With this technique, which was derived from techniques used by electrophysiologists to study event-related potentials, trial-based rather than block-based experiments can be carried out. These trial-based designs have a number of methodological advantages, but also some drawbacks: a subject’s individual performance on a subsequent cognitive test can be taken into account so that trials can be categorized post hoc according to each subject’s performance. When for example studying memory encoding, activations for the different items can be contrasted according to whether they are subsequently remembered or forgotten in a subsequent memory test in each individual. In this way we can identify brain regions with greater activation during encoding of different (material-specific) items that are subsequently remembered compared to items that are subsequently forgotten (subsequent memory effects) representing the neural correlates of memory encoding.108
A previous study compared results from a blocked and event-related analysis of memory fMRI of words, pictures, and faces: only the event-related analysis of successfully encoded stimuli showed significant activations in the anterior MTL, whereas simply viewing the different stimuli (using a blocked analysis without taking into account whether items were subsequently remembered or not) revealed predominant activation in the posterior hippocampus.68 This study provided evidence for a functional dissociation between anterior and posterior hippocampal regions.
In summary, although event-related designs are less powerful than blocked designs at detecting activation and are also more vulnerable to alterations in the hemodynamic response function, they have the big advantage of permitting specifically the detection of subsequent memory effects due to successful encoding.
21.6 Functional Connectivity
The possibility to analyze functional connectivity in patients with epilepsy has significantly improved our understanding of seizure generation and propagation. The analysis of functional connectivity during cognitive tasks allows studying the neuronal networks that subserve these tasks.109
During the last few years several studies have demonstrated altered connectivity within the epileptic network but also in networks of cognitive function such as language and memory. In patients with left TLE compared to healthy controls the functional connectivity within the expressive language network was reduced, most likely due to the underlying disease.110 More specifically, functional connectivity was decreased in the left hemisphere irrespective of the epileptogenic focus111 and within the prefrontal and frontotemporal networks,112 which was associated with impaired performance on language assessment.112 In right TLE, coupling between functional and structural measures for left language lateralization has been shown, while in left TLE decoupling between structural and functional measures suggested more complex language networks.113
Other studies evaluating memory function showed that functional connectivity was reduced between the posterior cingulate and the epileptogenic hippocampus and increased between the posterior cingulate and the contralateral hippocampus.114, 115 A recent study used a visual scene encoding task to evaluate memory function in healthy controls compared to patients with TLE. In this study, patients with left TLE demonstrated a significant decrease in functional connectivity to the inferior temporal, occipital, cingulate, and parietal cortices and the thalamus. In addition the authors showed that orbital frontal activity correlated with structural measures of tract coherence in the fornix, which led to the suggestion that this might be the structural correlate of reduced functional connectivity.116 Vollmar and colleagues reported similar observations of increased connectivity of motor and cognitive networks in patients with juvenile myoclonic epilepsy.117 However, whether functional connectivity studies may prove clinically useful still needs to be established. A potential application is the use as a clinical predictor of postoperative outcome following surgery, and studies are just beginning to emerge that address this possibility.
21.7 Conclusion
In conclusion, fMRI is a promising, noninvasive tool to study the effects of chronic epilepsy and epilepsy surgery on cognitive functions and factors that are associated with effective functional reorganization/ plasticity after surgery.
From a clinical perspective, many of those recent imaging studies increasingly emphasize the need to consider selective as opposed to extended temporal resections (and in particular to spare as much as possible of nonlesional functional tissue) to minimize the risk of cognitive impairment, but at the same time provide the same chance of good seizure control. It is hoped that, in the near future, cognitive fMRI may be able to provide reliable results in individual patients, which when used in concert with other clinical, electrophysiological, and imaging results will not only predict postoperative cognitive decline in single patients but ultimately help prevent them.
Therefore, one of the aims of future work is now to integrate fMRI data with structural MRI into neuronavigation systems, including interventional MRI, and to repeat fMRI studies intraoperatively in order to enable image guided resections.11 Hurdles to overcome include the different anatomical distortions of T1-weighted MR and EPI fMR images but also physical distortions such as brain shift, which may be as much as 2 cm after surgery.118
There is hope that constant improvements in hardware and software and in particular functional MR imaging at higher magnetic fields will lead to improved signal-to-noise ratio. A recent fMRI study compared the presurgical localization of the primary motor hand area on a 3 T and 7 T Siemens scanner with identical investigational procedures and comparable system specific sequences. Results showed significantly higher functional sensitivity of the 7 T system (measured via percentage signal change, mean t-values, number of suprathreshold voxels, and contrast-to-noise ratio), although 7 T data suffered from a significant increase of artifacts such as ghosting and head motion.119 However, results are promising that ultra-high-field systems provide a clinically relevant increase of functional sensitivity for patients also in the MTL, which might lead to strong and reliable hippocampal activation at an individual level.
Another exciting area is the use of fMRI to study brain network dynamics. Functional connectivity can elicit neuronal networks that contribute to various cognitive tasks. It has been shown that cognitive impairment is often accompanied by reduced functional connectivity. Whether these methods may add to the prediction of postoperative cognitive outcome remains a topic of current research. Together with tractography, which can provide a means to understand the distributed and interrelated structural networks of the brain, functional connectivity can be useful to understand seizure propagation and spread and how this may be related to impaired function. An appealing speculation is that, ultimately, this information, along with data from modalities such as MEG, ESI, and EEG-fMRI, could be used to disconnect seizure foci from the rest of the network.
Finally, fMRI may help to improve the individual prognosis of even subtle cognitive deficits, but also stimulate the development of new therapeutic strategies for cognitive rehabilitation. An exciting possibility for future work could therefore be to evaluate whether it is possible to modify dysfunctional networks prior to surgery, i.e., by applying specific learning strategies to the patients in order to optimize or even improve the chances of a good cognitive outcome following epilepsy surgery.
References


















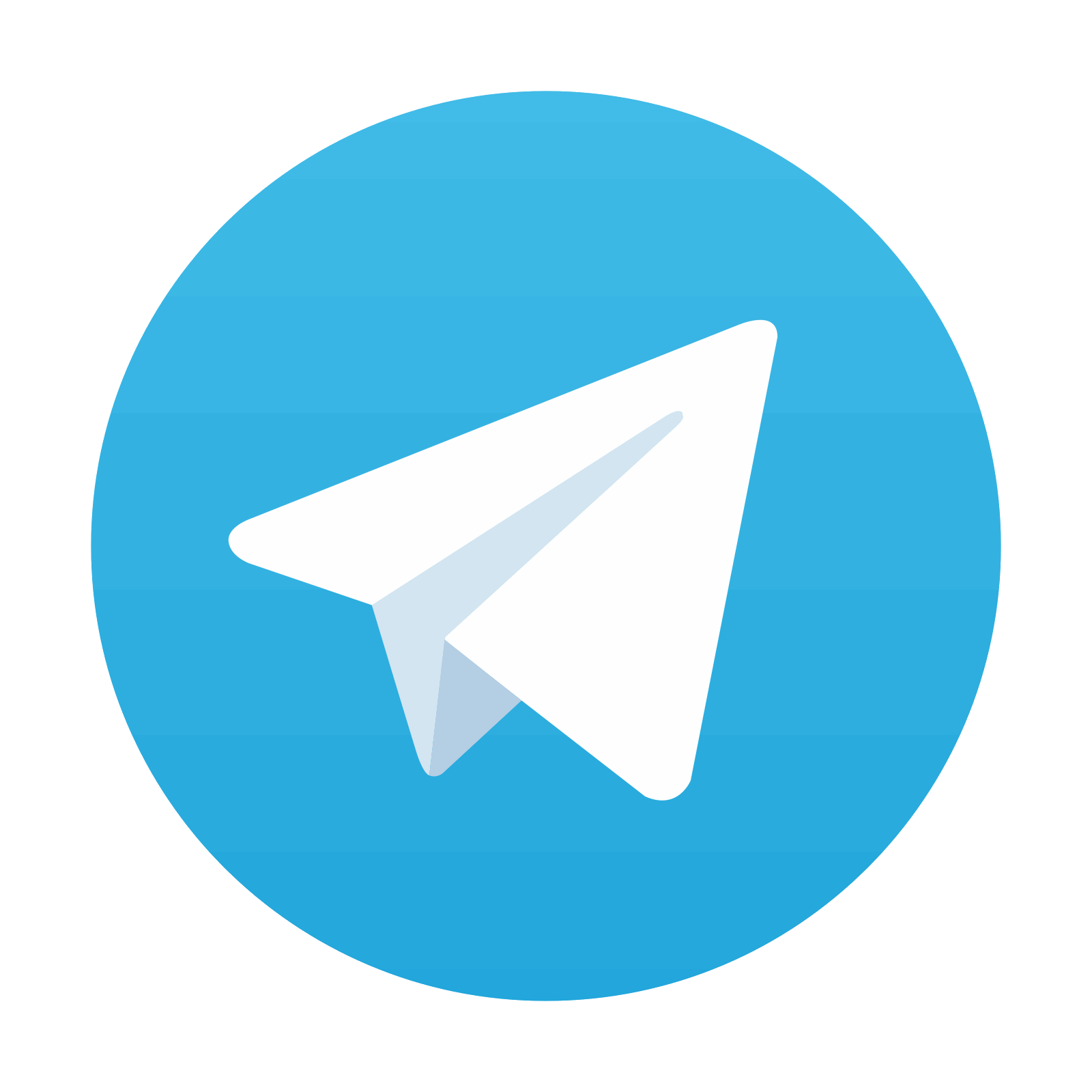
Stay updated, free articles. Join our Telegram channel

Full access? Get Clinical Tree
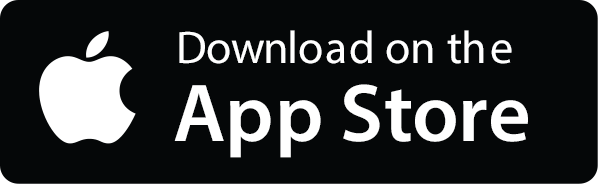
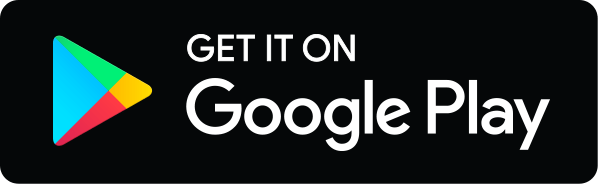
