7.1 Introduction
While the study of the gray matter has been the main focus of epilepsy research, observations of white matter (WM) atrophy and gliosis date back to early seminal histopathological assessment of surgically resected tissue in patients with temporal lobe epilepsy.1 WM may subserve seizure propagation, forming an integral part of the epileptogenic network. Recent advances in MRI techniques and quantitative postprocessing algorithms have provided unprecedented tools to examine the microstructure and architecture of this compartment. While early studies based on manual segmentation have provided volume measurements of this compartment, more recent computational approaches integrating various imaging contrasts, such as T2 relaxometry, diffusion tractography, and myelin imaging, lend biologically meaningful indices of its microstructural properties. In the past two decades, a large body of work has indeed revealed novel information regarding the distribution and nature of WM pathology in epilepsy. Together with gray matter findings, these results consolidate the view that focal epilepsy is a system-level disorder.
7.2 Voxel-Based Techniques
Early MRI studies of patients with temporal lobe epilepsy (TLE) noted WM atrophy alongside hippocampal anomalies during visual inspection.2 Such observations motivated quantitative analysis using manual volumetry. Similar to hippocampal volumetry, manual tracing requires expert knowledge of anatomy and provides a single summary statistic of the queried structure. In particular, its application to the fimbria-fornix, the major projection of the hippocampus in TLE, revealed significant atrophy concordant with the side of hippocampal atrophy.3 While the time-consuming nature of the procedure has confined its application to small WM structures, automated methods enabled efficient quantitation of lobar and global volumes. Indeed, several studies have identified marked reduction of the WM lobar volume in the temporal, frontal, and parietal lobes mainly ipsilateral to the side of seizure focus.4 The degree of WM loss was associated with disease duration4 and the severity of cognitive impairment, including (but not limited to) processing speed and full-scale and verbal IQ.5 Voxel-based morphometry studies in TLE have shown WM reduction mostly colocalized with GM abnormalities.6 Particularly, our group found WM loss in the temporopolar, entorhinal, and perirhinal areas ipsilateral to the seizure focus, suggesting a disconnection process involving preferentially frontolimbic pathways.7
WM pathology has also been studied using T2 relaxometry, a quantitative analysis of T2-weighted signal. Region-of-interest analysis and voxel-based statistical comparisons reported group-level increases in the anterior temporal lobe WM in TLE patients.8 We showed that while hippocampal T2 relaxometry provides a correct lateralization of the seizure,9 prolongation of WM T2-relaxation time in the temporal stem occurs in a rather bilateral and symmetric fashion.10 Altogether, these early studies set the basis for large-scale extrahippocampal WM disruption in TLE.
7.3 Diffusion-Weighted MRI
Despite relatively coarse spatial resolution (typically 2 mm), diffusion-weighted MRI and its analytical extensions, particularly diffusion tensor imaging (DTI), exploit the diffusion of water molecules to probe tissue microstructure, which is especially useful in coherently organized tissue, such as the WM.11 While its sensitivity and specificity for the identification of the primary epileptogenic lesion are modest, this technique has revealed WM anomalies in several forms of epilepsy, particularly TLE.12
The most often reported diffusion MRI metric is related to diffusion anisotropy (e.g., fractional anisotropy, FA), which tends to be high in regions where densely packed axons have very similar orientations. Reduced diffusion anisotropy at the expense of increased radial diffusivity is interpreted as reduced axonal density and alterations of myelin architecture. Histological examination of the fornix and the temporal pole WM structures derived from TLE surgical specimens have confirmed these anomalies.13, 14 Small caliber axons, in particular, are reduced in number in TLE patients.15 Although perhaps to a lesser degree, other histological features, such as WM gliosis, may also play a role in altered water diffusion metrics. Notably, orientational coherence of axons is reduced by the infiltration of oligodendrocytes, which are more common in regions of WM hyperintensity, and may reflect an attempt to compensate for progressive myelin loss.16 Independently of gliosis, the number of heterotopic neurons, commonly seen in the superficial WM, is higher in TLE patients than in controls;17 however, their density and overall dimension appear to be insufficient to modulate water diffusion profiles.
7.3.1 Diffusion Tensor Imaging of Deep White Matter Tracts
Diffusion data can be processed through tractography algorithms,18 which reconstruct fiber pathways running along plausible trajectories in voxel space. While somewhat challenged in regions of crossing fibers and gray-white matter interface, tractography can generate consistent results for a number of deep WM tracts.19 Probabilistic tract atlases further enable automated tract labeling by providing a standard template for fiber location and orientation.20 The virtually reconstructed trajectories have shown good correspondence with postmortem dissection, and have been cross-validated by comparative sacrificial tracing studies in nonhuman primates.21 Diffusion characteristics can be also assessed throughout the brain without a priori hypothesis. Tract-based spatial statistics (TBSS) allow projecting individual FA values onto a group-wise WM skeleton, so that the structural core is reasonably aligned even in the presence of slight misregistration across subjects;22 furthermore, sensitivity is increased due to fewer number of voxel-wise comparisons.
In TLE, diffusion abnormalities are evident in limbic structures,23 including the fornix and cingulum,24 but also in the temporal lobe association tracts, such as the arcuate and uncinate fasciculi.25 Although changes are more marked ipsilateral to the seizure focus, there is a considerable degree of bilateral involvement in a number of tracts. Tracked-based segmental analysis of these tracts has revealed centrifugal pattern of mean diffusivity increases, where the degree of anomalies gradually tapered off as the tracts exited the temporal lobe.26 Outside the temporal lobe, diffusion anomalies are found in the corpus callosum and the frontoparietal pathways. Studies on the effect of hemispheric laterality on diffusion parameters have yielded conflicting results, with some reports showing more alterations in right TLE,27 while others showed the opposite.28 These discrepancies could be due to differences in the TLE cohorts with respect to the severity of hippocampal damage, precipitating factors, or disease duration. Several factors appear to influence the degree and extent of WM diffusion abnormalities, yet the largest effect appears to be related to the presence of mesial temporal sclerosis. Patients with severe mesiotemporal lobe sclerosis have more widespread and severe diffusion abnormalities than those with subtle pathology.27 Diffusion anomalies have also been shown to be positively correlated to longer disease duration.29 Combining TBSS with resting-state functional MRI, we have highlighted the relationship between temporal lobe WM anomalies and reduced functional connectivity of the default mode network,30 an assembly of regions thought to play a key role in internally generated cognition, memory, and future planning.
Electron microscopy of TLE fornix specimens obtained at surgery revealed considerably more damage in patients with severe hippocampal sclerosis, consisting of reduced axonal density, and myelin abnormalities.13 Similar characteristics were noted bilaterally in the TLE fornix of autopsy specimens.15 Axonal degeneration is expected in the fornix ipsilateral to the focus, and although a fraction of axons crosses the midline toward the contralateral hippocampus, ipsilateral hippocampal pathology may not be the only factor leading to bilateral abnormalities of the fornix and other WM fascicles. The fact that diffusion anomalies may be present soon after diagnosis31 is suggestive of a likely contribution of neurodevelopmental processes. Recently, a multifactorial model of WM damage in TLE was proposed, in which the underlying pathology and effects of seizures play a significant role in comparison to genetic factors.32
Postoperative diffusion MRI studies have revealed two distinct profiles that may inform of specific histopathological changes. Comparing post- to presurgical values, several studies have found decreased FA and increased MD in the fimbria fornix, parahippocampal cingulum, uncinate fasciculus, and inferior fronto-occipital fasciculus ipsilateral to the epileptic focus.33
Alterations of diffusion parameters of WM fascicles directly affected by surgical resections are likely due to Wallerian degeneration. Indeed, acute reduction of diffusion anisotropy at the expense of reduced longitudinal diffusivity34 is reflective of axonal fragmentation or beading; conversely chronic increased radial diffusivity likely relates to reduced axonal density and myelin degradation.35 WM distant from the resection, including the corona radiata and external capsules, showed increased FA.28, 33 While originally deemed as plastic phenomena and functional adaptations, the paradoxical increase of FA in ipsilateral tracts is likely due to the degeneration of a single fiber population in regions of fiber crossing. Conversely, increased anisotropy in the hemisphere contralateral to surgery may in fact be related to plastic changes in language networks.28
Compared to the large body of literature in TLE, fewer studies have addressed WM alterations in focal cortical dysplasia (FCD). Voxel-based analysis has revealed reduced anisotropy and increased diffusivity, subjacent to the MRI-visible lesion or diffusely across both hemispheres.36, 37 Aside from the direct effects of the structural pathology, reduced anisotropy may be related to abnormal myelination or the presence of ectopic neurons. Conversely, increased diffusivity has been interpreted as a defect of neurogenesis or cell loss resulting in increased extracellular space. Only a few studies to date have used fiber tractography in FCD. An early study showed both a reduction of the subcortical fibers and reduced connection between the subcortex and deep WM.38
7.3.2 Surface-Based Analysis of the Superficial White Matter
Most DTI studies have focused on assessing the integrity of deep WM bundles. The superficial WM immediately subjacent to the cortical mantle has been largely neglected. Given its anatomical proximity to neocortical networks and role in maintaining long-range connectivity, the analysis of this compartment may provide novel insights in the pathophysiology of various epilepsy syndromes. The complex fiber trajectories of the less densely myelinated superficial WM challenge conventional diffusion tractography and TBSS.39 Conversely, surface-based approaches allow vertex-wise sampling of diffusion parameters at multiple WM depths. In TLE, diffusion alterations (increased diffusivity and decreased anisotropy) of the superficial WM are found primarily in ipsilateral limbic regions40 (Figure 7.1). In extratemporal epilepsy related to FCD Type II, subtle diffusion anomalies are found both at the lesional site and in the normal appearing perilesional WM41 (Figure 7.2).
Figure 7.1. Surface-based mapping of superficial white matter (SWM) diffusion in TLE. (A) After generating the inner (WM-GM, green) and outer (GM-CSF, red) cortical surfaces, we computed a Laplacian potential field between the WM-GM interface and the ventricular walls to guide placement of a surface running 2 mm below the WM-GM boundary (SWM, yellow). Fractional anisotropy (FA) and mean diffusivity (MD) were sampled on this surface. (B) SWM diffusion anomalies in right (RTLE) patients relative to controls showing predominantly ipsilateral temporal and limbic anomalies. Similar anomalies are found in left TLE (not shown). Findings were corrected for multiple comparisons thresholded at PFWE < .05 using random field theory for nonisotropic images (cluster threshold p < .01).
Figure 7.2. Multisurface profiling of diffusion MRI (dMRI) parameters in focal cortical dysplasia. (A) For lesion profiling, individual patients (normalized with respect to corresponding regions in healthy controls) are plotted as a function of intracortical and subcortical level, separately for Type IIA (red dot) and Type IIB (black dot); zero reference line indicates the mean of controls; mean values and standard deviations in patients are shown as horizontal lines, color coded by patient group. An example case is with arrowheads pointing to the lesion on the T1-weighted MRI. Overall, Type IIB lesions display increased subcortical mean diffusivity (MD), while IIA are associated with decreased fractional anisotropy (FA) at the gray matter (GM)-white matter (WM) interface and subcortical WM. (B) For distance-based profiling, normalized features are averaged across cortical and subcortical surfaces, and plotted relative to the geodesic distance from the primary lesion in steps of 4 mm. Subcortical diffusion alterations extend up to 16 mm, with anomalies being more marked in Type IIB. Conversely, reduced cortical FA, specific to Type IIA, extended up to 6 mm outside the lesion. Asterisks indicate significant differences with controls and between cohort contrasts after correction of multiple comparisons (FDR < .05); small dots indicate uncorrected findings at p < .05.
7.3.3 Diffusion Tensor Imaging: Relation to Cognition
Cognitive abilities are dependent on the orchestrated action of several brain regions interconnected by association and commissural fibers, many of which are affected in epilepsy. It is thus not surprising that TLE patients may have cognitive deficits beyond temporal lobe functions.42 Diffusion metrics of the superior longitudinal fasciculus, cingulum, and temporal lobe WM correlate with working memory performance.43 Similarly, diffusion anisotropy of the uncinate, arcuate, and inferior fronto-occipital fascicles, as well as temporal cingulum positively correlate to verbal memory.44 Moreover, alterations of diffusion parameters in the temporal lobe WM are associated with delayed and immediate memory performances.45, 46 Preliminary data in patients with both idiopathic generalized and focal epilepsy show WM diffusion alterations already at disease onset,31 which were shown to correlate with executive functions.47
Tractography has shown potential clinical utility in presurgical mapping of the language network with the goal of avoiding deficit. In TLE, diffusion abnormalities in the arcuate, uncinate, and inferior fronto-occipital fasciculi have been shown to be associated with impaired language performance.48 Left TLE patients who exhibited atypical language activations in functional MRI presented reduced FA asymmetry in connecting WM pathways,49 and the structural disruption was correlated with decreased functional connectivity between frontal and temporal cortices.50 Notably, a greater asymmetry in pathways connecting to eloquent cortices correlated with worse decline in naming function after anterior temporal lobe resection also in the dominant hemisphere.51 WM diffusion characteristics may thus provide valuable information to predict which patients are at higher risk of developing cognitive deficits. So far however, most studies have been group-based; future efforts should assess the predictive value of this technique at an individual level. Using FA asymmetry of the arcuate fasciculus, language could be lateralized in 83% of patients, with increases up to 96% when combining information from functional MRI and handedness.52 Additionally, if progressive WM abnormalities are secondary to ongoing seizures, then appropriate treatment could halt further cognitive deterioration. Another application of tractography has been in surgical planning to avoid functional deficit. More recently, WM damage in TLE patients has been shown to be a factor associated to poor postsurgical outcome. A study using tractography and automated fiber quantification found that diffusion abnormalities in the ipsilateral dorsal fornix and in the contralateral parahippocampal bundle contribute to persistent postoperative seizures.53
7.4 Analytical Approaches to the White Matter beyond Tensor Model
The tensor model has provided extremely valuable information regarding WM microstructure in epilepsy, yet DTI has a number of limitations. First and foremost is its incapability of resolving fiber crossing, which may result in erroneous estimations of diffusion anisotropy. Second, the tensor model assumes that diffusion is Gaussian, while in reality the complex intracellular and extracellular environment causes the diffusion of water molecules to deviate considerably from this pattern; this oversimplification prevents precise description of tissue microstructure. Many alternative analytical methods have been proposed in the last decade that extend the capabilities of diffusion MRI and are beginning to be used for the study of epilepsy as acquisition requirements are relaxed to the point that they can be applied in clinical populations. For example, diffusion kurtosis imaging, an extension of tensor model to measure the degree to which diffusion deviates from Gaussian behavior, i.e., mean kurtosis, yields complementary information regarding diffusion heterogeneity.54 In TLE, in addition to revealing more marked diffusion profiles along ipsilateral WM fibers,55, 56 this technique has shown abnormalities in both gray and white matter extending to regions not detected by conventional DTI measures. Diffusion spectrum imaging, a method querying complex distributions of intravoxel fiber orientation, has revealed that reduced FA in the mesiotemporal lobes in TLE patients likely results from the reduction in intracellular diffusion related to neurite (i.e., dendrites and axonal) loss, whereas reduced FA in extratemporal regions was confounded by fiber orientation changes, potentially reflecting disorganized fiber orientation and packing.57 Restricted spectrum imaging is based on a multicompartment model that quantifies the degree to which diffusion heterogeneity within a voxel is driven by intra-axonal water versus extracellular diffusion, while accounting for fiber orientation. This multishell acquisition method has detected more widespread reduction of neurite density than anisotropy.58
Fixel-based analysis is a diffusion-weighted MRI reconstruction technique that combines the measurements of fiber cross-sectional area, a measure of morphology, with microstructural information of fiber density,59 thereby providing a sensitive marker of intra-axonal volume. Fixel-based morphometry has assisted in identifying WM atrophy secondary to repeated focal seizures and global insults in TLE patients.32 Neurite orientation dispersion and density imaging, commonly referred to as NODDI,60 is another advanced diffusion imaging reconstruction technique based on a multishell acquisition protocol that allows an estimation of intra- and extracellular volume fractions in order to measure neurites morphology. This technique has the advantage to model both gray and white matter, a desired ability in the investigation of epilepsy, yet so far not exploited. As more complex models are introduced to probe individual tissue components, histologic-MRI correlational studies may help validate the biological underpinning of the novel metrics.
7.5 White Matter Connectome
Growing evidence of extensive structural and functional abnormalities in epilepsy has emphasized the notion that epilepsy is a disorder affecting distributed neural networks. Recent methodological advances in graph theoretical analysis lend tools to characterize topological aspects of interconnected regions.61 In this mathematical formalism, the brain connectome is modeled using a parcellation scheme of gray matter regions, i.e., nodes. Importantly, WM pathways reconstructed by in vivo diffusion tractography provide a good approximation of the actual anatomical connectivity, forming the edges between nodes. Notably, however, there is so far no consensus on metrics of connectivity strength, with some studies adopting parameters based on deterministic tractography (including FA, number of streamlines, and fiber lengths), while others using fiber connections density derived from probabilistic tractography.
Most DTI-based network studies have focused on focal epilepsy, particularly TLE. Collectively, findings have argued for a reorganization of macrolevel topological properties. In local circuits, on the other hand, increased integration was found within limbic and default mode networks, even though fiber connectivity among these regions was reduced.62 This dichotomy of changes between large-scale topology and individual fiber level illustrates the advantage of network analyses in addressing how regions interrelate as a whole, which is not possible via querying the integrity of a specific connection, and may potentially provide useful diagnostic and prognostic information for the assessment of individual patients.63 Indeed, combined with machine learning algorithms, presurgical connectome data have shown the ability to predict surgery outcome in 70% of TLE patients, an accuracy similar to “expert-based” clinical decision.64 Moreover, the presurgical connection strengths between ipsilateral temporal and extratemporal regions have been able to predict seizure-free surgical outcome in TLE patients with performances far beyond that of presurgical clinical data.55 Specifically, compared to seizure-free patients, presurgical connectomes in non-seizure-free patients exhibited higher integration within ipsilateral temporal lobe subnetwork.61
In light of work in healthy populations that utilizes structural connectomes to simulate functional dynamics,65 recent advances in network diffusion science offer new models to simulate the communication process between network nodes and have provided preliminary evidence for the spreading pattern of neuronal damage from the seizure focus at a group level in TLE.66 Emerging data suggest that computational models of seizure spread and epileptogenicity based on structural connectomes67 may also help localizing surgical targets68 and possibly improve the prediction of postsurgical seizure outcome.69 In patients with IGE, network analysis based on diffusion MRI and resting state functional MRI data has identified altered topology in regions that had previously been hypothesized to play a role in the epileptogenesis of this condition, including the mesial frontal cortex, putamen, and thalamus.70, 71
To date, WM organization in cohorts with validated FCD has been seldom studied. Our recent study employing gray matter structural covariance and functional connectivity analysis suggests marked network rearrangement particularly in FCD I.72 In patients with frontal lobe epilepsy and suspected subtle dysplasia, overall reductions of global and nodal efficiency have been observed, as well as network segregation. Given the macro-scale analyses, it is likely that some connectome findings may relate to cognitive difficulties commonly seen in epileptic populations. Indeed, a structural connectivity study reported that patients with severe cognitive impairment may have more marked alterations in network topology compared with healthy individuals as well as patients with only little cognitive impairment.73
7.6 Conclusion
Epilepsy is a network disorder and the WM is the substrate connecting its various components. A proper conceptualization of this condition entails equal attention to both the gray and white matter at the site of the seizure focus and at a distance from it.74 Novel in vivo diffusion-weighted MRI acquisition and reconstruction techniques, but also emerging imaging methods assessing myelin content,75 provide an increasingly comprehensive view of this pivotal compartment and its relationship to neuronal processes. They have successfully characterized pathology and mapped its regional distribution. Although initial studies have suggested the relevance of single markers of WM integrity to clinical outcomes, a better understanding of this relationship demands combined analysis of descriptors of gray and white matter microstructure, connectome-level features, and lesional markers. In this context, machine learning is the method of choice to extract critical features, patterns, and relationships from such high-dimensional datasets that might otherwise be missed. Moreover, paralleling the move toward big data analyses in neuroscience, epilepsy research would benefit from data-sharing initiatives to validate results and address new hypotheses.
References













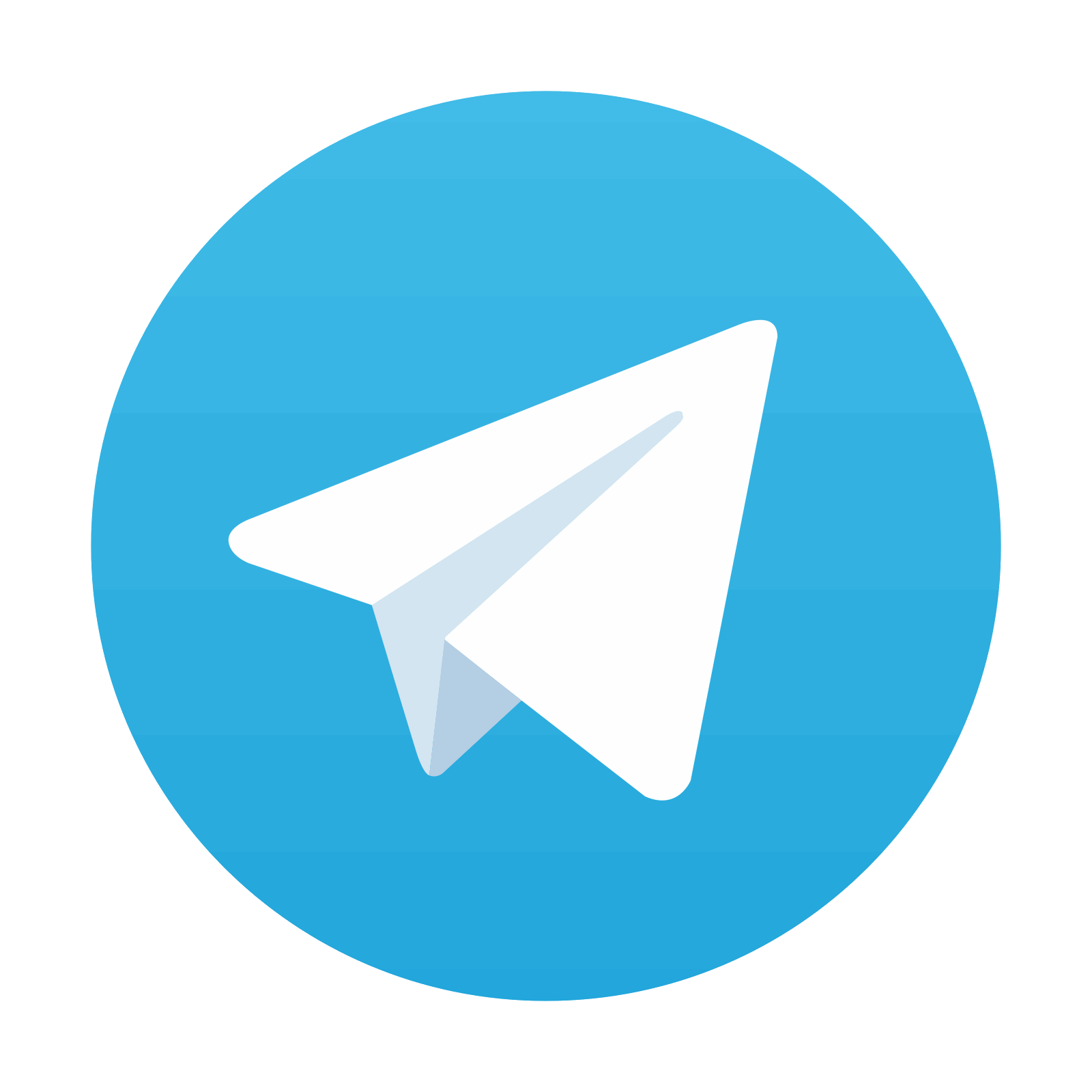
Stay updated, free articles. Join our Telegram channel

Full access? Get Clinical Tree
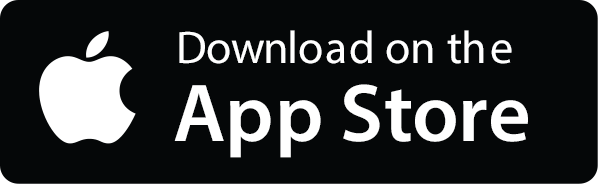
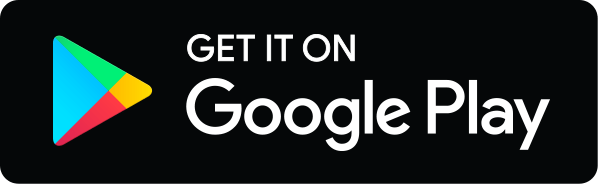
