(1)
Facultad de Ciencias Médicas, Pontificia Universidad Católica Argentina, Buenos Aires, Capital Federal, Argentina
Abstract
There are many reasons for the lack of sleep in our society that operates 24 h a day, 7 days a week, constantly. The main disturbing factor has been the technological advance of being able to light our evenings artificially. This Chapter analyzes the impact of the lack of sleep in the “24/7 Society”, principally the disruption of the three ANS physiological programs. Typical examples of a desynchronized ANS are jet lag, shift work and chronodisruption, the metabolic syndrome and mental illnesses being also examples of a desynchronized ANS. The chronobiological aspects of normal and pathological brain aging and cancer are discussed.
Keywords
24/7 SocietyAdjuvant chronobiological treatmentChronodisruptionChronodisruption in cancerChronodisruption in mental illnessesChronodisruption in metabolic syndromeChronodisruption of brain agingJet lagLight at nightMelatoninShift workObjectives
After studying this chapter, you should be able to:
Describe the mechanisms underlying the inadequate experience of day and night in the “24/7 Society.”
Describe the disruption of the three ANS physiological programs in a 24-h cycle due to the inadequate experience of day and night.
Describe the physiological changes observed in jet lag, shift work. and chronodisruption as examples of a desynchronized ANS.
Understand why the chronobiological treatment of a desynchronized ANS is needed for full recovery in most cases.
Describe the metabolic syndrome and mental illnesses as examples of a desynchronized ANS.
Understand the chronobiological aspects of normal and pathological brain aging.
Understand the chronobiological aspects of cancer.
Due to the “24/7 Society,” the ANS Has Lost Adequate Experience of Day and Night
There are many reasons for lack of sleep in our society, which operates 24 h a day, 7 days a week, constantly. The early hours of school or work, TV programs increasingly displacing the “prime time” ones late at night, the daily stress, or the widespread use of foods and beverages rich in caffeine are among the precipitating factors. However, the main factor has been the technological advance being able to artificially light our evening.
Our hominid ancestor, Homo erectus, used caves as shelter and must have begun to use fire about a half million years ago. Homo sapiens built artificial shelters protected from the sun’s rays and manufactured lamps that allowed him to extend the daily lighting period about 70,000 years ago. The first lamp invented was made of a shell, hollowed-out rock, or other similar nonflammable objects and was filled with a combustible material (probably dried grass or wood), sprinkled with animal fat (the original lighter fluid) and ignited.
In the last 200 years, we have shifted our routines from rural environments to the cities and from outdoor life to confinement in our homes. Furthermore, with the advent of electric light we have become progressively isolated from the natural cycles of light and darkness that shaped our biological rhythms for millions of years. This is an environmental mutation, with an increasing impact on the quantity and quality of our sleep.
The internet explosion has added a further complication. Increasingly, individuals spend part of their nights in front of lit monitors (LCD, tablets, smartphones), screens that produce at least two phenomena of concern to the sleep–wake cycle: (a) plundering the natural period of sleep by reducing it to dangerous levels; (b) adding a disruptor factor, the monitor light during the circadian period causes phase delays of the biological clock, which produces a later sleep on subsequent nights, tending to perpetuate the situation of nocturnal sleep deprivation [1].
The artificial light striking the retina from dusk until dawn exerts a strong inhibitory activity of hypothalamic neurons that induces sleep and a strong excitatory activity of brain mechanisms that maintains wakefulness. As we have already discussed in Chap. 2, suppression of the nocturnal release of melatonin occurs, which is responsible for synchronizing our circadian rhythms and for the “opening of the sleep gate,” (Fig. 2.12) [2]. If someone tries to sleep at 18:00 or 19:00 h, he or she would probably take a 1- to 2-h nap. If instead the time to sleep onset shifts to 21:00 or 22:00 h, a 6- to 8-h consolidated sleep tends to occur. This sharp increase in sleep propensity is due to inhibition of wakefulness promoting action of the SCN given by melatonin. Ambient light by inhibiting the secretion of melatonin, reduces sleepiness, promotes alertness, and interferes with sleep.
In daylight conditions, the SCN exerts maximum wake-up activity toward the end of the period of wakefulness, a “second wind” that keeps us awake despite the sleep debt already accumulated in wakefulness. Before the widespread use of electric light, people experienced this “second wind” in the afternoon (17:00 to 18:00 h) and possibly kept alert until nightfall. However, exposure to artificial light after dark has incorporated a signal indicating to the SCN a time of day that is not real, deferring the “second wind” and delaying the secretion of melatonin (Fig. 8.1).
Indeed, technology has disconnected us from the natural 24-h day in which our species has evolved. A comparison of the biological effects of reading an electronic book on a light-emitting device with reading a printed book in the hours before bedtime indicated that those reading an electronic book took longer to fall asleep and had reduced evening sleepiness, reduced melatonin secretion, later timing of their circadian clock, and reduced next-morning alertness than those reading a printed book [1].
Because about 20% of electricity consumption worldwide is devoted to the production of light, many governments are eliminating traditional incandescent lamps (emitting in the red) for more efficient LEDs in search of savings. However, this white light solid state is typically rich in blue light, which is the portion of the spectrum that most inhibits photoreceptor retinal ganglion cells and hence the secretion of melatonin, which further amplifies the disruptive effect on sleep–wake rhythm [3]. Therefore, sleep topics are not only a medical problem, but substantially influence the social and organizational frame of society.
Both longitudinal statistics in northern hemisphere countries and regional data in Latin America indicate that in just 40–50 years, we have reduced our sleeping time by 25% [4]. Longitudinal statistics from the National Sleep Foundation, USA, indicate that the number of daily hours of sleep has fallen since 1960 to date from 8.2 to 6 h daily. In our study, we verified that 65% of the population, regardless of age, reported having sleep disorders in the last 12 months; 40% of these disorders were described as moderate to severe, and there was a sleep deficit of about 2 h a day. All respondents recognized the negative consequences of poor sleep for health and quality of life [5].
In the USA, 30% of employed adults and 44% of night workers sleep less than 6 h per night, versus less than 3% of the adult US population 50 years ago [6]. Globally, children sleep about 1.2 h less on weekdays before school activity than a century ago. It is noteworthy that children tend to become hyperactive instead of sleepy when they do not get enough rest and have difficulty in concentrating, focusing attention; thus, deficiency of sleep can be confused with hyperactivity/attention deficit disorder, a condition “over-diagnosed” in many societies [7].
The Disruption of the Three ANS Physiological Programs (“Body Configurations”) Is a Major Consequence for the “24/7 Society”
We discussed in Chap. 2 how the three different “bodies” (wakefulness, slow-wave sleep, and REM sleep) necessarily follow each another harmoniously to ensure health. A 76-year-old man (the current life expectancy in our society) sleeping 8 h daily will live 50 years in the physiological state of wakefulness, 20 years in slow-wave sleep, and 6 years in REM sleep. However, our society has reduced by 25% the amount of time spent sleeping over the last 40 years. Therefore, the above calculation now changes to a distribution of 55 years of wakefulness, 15 years of slow-wave sleep and 6 years of REM sleep (Fig. 8.2).


Fig. 8.2
The three different “bodies,” wakefulness, slow-wave sleep (NREM sleep) and REM sleep, must necessarily follow each another harmoniously to ensure health. Upper panel: a 76-year-old man sleeping 8 h daily will live 50 years in the physiological state of wakefulness, 20 years in slow-wave sleep and 6 years in REM sleep. Lower panel: reduction of 25% of sleep over the last 40 years leads to predominance of the wakefulness state and reduction of the slow-wave sleep, associated with cardiovascular disease, metabolic syndrome, obesity, and type II and type III diabetes
A timed view of the ANS from clinical standpoints allows interpretation of symptom intensity and mortality of human diseases, conditions, and syndromes that exhibit a 24-h pattern and are thus related to the three body configurations. Twenty-four-hour patterns are characteristic of more than 100 acute and chronic common and rare human diseases (Fig. 8.3) [8]. The worst symptoms of many of these tend to be expressed either late evening/overnight or early morning, thus significantly compromising nocturnal sleep, daytime productivity, and overall quality of life, and are therefore of great relevance clinically to patient management, for example [8]:

Cardiac—atrial premature beats and tachycardia, paroxysmal atrial fibrillation, atrial–ventricular block, paroxysmal supraventricular tachycardia, ventricular premature beats, angina pectoris, acute (nonfatal and fatal) incidents of myocardial infarction, sudden cardiac arrest, acute cardiogenic pulmonary edema, heart failure
Vascular and circulatory system—hypertension, acute hypotension/syncope, intermittent claudication, venous insufficiency, standing occupation leg edema, arterial and venous branch occlusion of the eye, menopausal hot flash, sickle cell syndrome, abdominal, aortic, and pulmonary thromboembolism, deep venous thrombosis, cerebrovascular transient ischemic attack, stroke
Respiratory—viral and allergic rhinorrhea, reversible (asthma) and nonreversible (bronchitis and emphysema) chronic obstructive pulmonary disease, cystic fibrosis, high-altitude pulmonary edema
Gastrointestinal tract—esophageal reflux, peptic ulcer, cyclic vomiting syndrome, biliary colic, hepatic variceal hemorrhage
Renal—colic, nocturnal enuresis, polyuria
Neural—frontal, parietal, temporal, and occipital lobe seizures, Parkinson’s and Alzheimer’s disease, hereditary progressive dystonia
Psychiatric/behavioral—major and seasonal affective depressive disorders, bipolar disorder, dementia-associated agitation, addictive alcohol, tobacco, and heroin cravings, and withdrawal phenomena
Pain—cancer, post-surgical, diabetic neuropathic and foot ulcer, tooth caries, fibromyalgia, sciatica, multiple sclerosis muscle spasm, and migraine (tension, cluster, hypnic, paroxysmal hemicranial headache)
Autoimmune and musculoskeletal—rheumatoid arthritis, osteoarthritis, spondylarthrosis, gout, Sjögren’s syndrome, systemic lupus erythematosus
Infection—susceptibility, fever, mortality
Skin—atopic dermatitis, urticaria, psoriasis, palmar hyperhidrosis
Ocular—bulbar conjunctival redness, keratoconjunctivitis sicca, intraocular pressure, anterior ischemic optic neuropathy, recurrent corneal erosion syndrome

Fig. 8.3
Chronopathology. Acrophases for several clinical entities across a 24-h cycle
The imbalance at the expense of slow-wave sleep imposed by the “24/7 Society” (Fig. 8.2) can be costly in countless aspects of our life and health. The impact of sleep deprivation is widespread and affects not only the physical, but also the psychological and social wellbeing. With impaired cognitive performance in the areas of attention, memory, and executive functions, the added emotional and behavioral consequences of sleep deprivation may explain the exasperated social behavior found in our present life [9].
The predominance of the sympathetic configuration of wakefulness is a strong predisposing factor for chronic, low-degree inflammation. The term “inflammaging” has been coined to denominate the contribution of inflammatory processes to the progression of aging [10]. However, inflammation is not only a matter of normal senescence, but is also observed in several diseases that can be linked to the predominant sympathetic configuration given by prolonged wakefulness.
Table 8.1
Etiology, symptoms, and treatment of chronodisruption
Etiology | Acute or chronic phases of most diseases Sleep deprivation, light at night (LAN) | Alteration of the central circadian pacemaker indicated by the alteration in melatonin secretion |
---|---|---|
Symptoms (phase and amplitude changes of circadian rhythms) | ANS dysfunction | Insomnia, somnolence, metabolic syndrome, thoracic-muscular sympathetic predominance, parasympathetic abdominal predominance, gastrointestinal disorders |
Somatic alterations | Tiredness, fatigue, stiff neck/back, low back pain, headache, drowsiness | |
Cognitive functions | Disorientation, loss of sociability and motivation, poor attention, performance, memory, or concentration | |
Behavior | Aggressiveness, impulsivity, hyperactivity, irritability | |
Psychiatric disorders | Depressive symptoms, personality disorders, anxiety | |
Therapeutic approach | Chronobiological treatment (exposure to morning light, melatonin supplementation at bedtime) In the case of acute or chronic diseases, the chronobiological treatment must accompany the specific treatment for “ad integrum” recovery |
Chronic, mild inflammation is multiply intertwined with other potentially deteriorating processes, among which mitochondrial dysfunction is of premier importance [10]. An abnormally prolonged sympathetic predominance, as in sleep deprivation, implies several immune remodeling processes that include tendencies toward enhanced proinflammatory signaling (Chap. 4).
Numerous epidemiological studies indicate the association of sleep deprivation with cardiovascular disease, metabolic syndrome, obesity, and T2DM [4, 11]. Moreover, today the association of these symptoms is emphasized, with dementia, particularly Alzheimer’s disease , which is often called “type 3 diabetes”. There is also epidemiological evidence for the link between poor sleep when working shifts and cancer, especially breast cancer [12].
Jet Lag, Shift Work, and Chronodisruption as Examples of a Desynchronized ANS
Changes in the environmental timing cues (zeitgebers) are associated with alterations in the body’s 24-h rhythms (Chap. 2). Under most circumstances, this adjustment to the environmental light/dark cycle is normal and consistent. However, mismatches can occur when changes in environmental demands are either sudden or severe. In shift work, there is a phase shift of the activity/rest cycle with regard to the light/dark cycle, whereas jet-lagged time zone travelers encounter a pattern of light and darkness, activity, and social schedules shifting together in time [13]. The endogenous circadian system is slow to adapt to new time cues, and until the correct phase relationship between biological rhythms and external zeitgebers is re-established, a host of physiological and behavioral problems can manifest. Similar problems are encountered by shift workers operating under new work schedules out of phase with the normal light/dark cycle, other competing zeitgebers, and their endogenous body clock.
Chronodisruption comprises the changes in amplitude and phase of circadian rhythms that are found as a comorbidity of most acute and chronic diseases. The observed chronodisruption may be due to a failure in one or more components of the clock itself, in the output signal to the different systems, in the presence of synchronizers, or in the transmission of information from the zeitgebers to the circadian clock (Fig. 8.4). In the clinic, the exact cause of an alteration is unlikely to be known, and in any case, most are multifactorial (Table 8.1) [14–17].


Fig. 8.4
Chronodisruption found as a comorbidity of most acute and chronic diseases. The observed chronodisruption may be due to a failure in one or more components of the clock itself, in the output signal to the different systems, in the presence of synchronizers, or in the transmission of information from the zeitgebers to the circadian clock
Table 8.2
General symptoms of jet lag
Anorexia or loss of appetite |
Apathy |
Bowel irregularities (constipation or frequent defecation) |
Clumsiness |
Daytime somnolence |
Decreased vigilance and attention domains |
Depression |
Diminished mental abilities (i.e., cognitive performance, concentration, judgment, decision making, memory lapses) |
Diminished physical performance |
Disorientation |
Fuzziness |
Gastrointestinal symptoms (e.g., bloating and upset stomach) |
General feeling of malaise |
Generalized fatigue and lethargy |
Glucose metabolism dysregulation |
Headache |
Impaired alertness |
Impaired task performance (increased accidents and errors) |
Inappropriate timing of defecation and urination |
Irritability |
Menstrual irregularity |
Mood disturbances |
Muscular pain |
Sleep loss |
Sleeping difficulties (inappropriate sleep at local time) |
Slowed reflexes |
Stress |
Tiredness (traveler’s fatigue) |
Traveler’s thrombosis (deep vein thrombosis) |
Trouble initiating and maintaining sleep |
Tumor progression is noted in chronic animal model |
As discussed in Chap. 2, almost every physiological function has a circadian “phase map ” consisting of an ordered sequence of peaks and valleys (Fig. 2.1). While the period of the phase map for different physiological functions is similar, the peaks and valleys of the maps generally do not coincide. Phase maps are also sensitive to environmental changes and can be transiently affected by temporal disruptions such as jet-lag disorder or shift work, or by the disruption imposed by an acute or chronic illness, regardless of its severity.
Jet Lag
Flight dysrhythmia , more commonly known as jet lag, comprises a constellation of symptoms consisting of daytime fatigue, impaired alertness, nighttime insomnia, loss of appetite, depressed mood, poor psychomotor coordination, and reduced cognitive skills, among other (Table 8.2) [18]. These symptoms are caused by the temporary misalignment between the circadian clock and external time, which occurs because of rapid travel across time zones. The number of time zones crossed and the direction of travel influence the severity of jet lag symptoms. Eastward travel tends to cause difficulty in falling asleep whereas westward travel usually interferes with sleep maintenance.
In jet lag, the recovery time for restoring the normal rhythm profile (re-entrainment) can differ significantly from one physiological function to another. Although during the period of re-entrainment individual circadian rhythms generally move in a direction that corresponds with that of the environmental time shift, in some cases the circadian system moves in a direction that is opposite to that of the environmental change, giving rise to a phenomenon called “splitting.” Phase map “partitioning” is then more complex, involving a partial re-entrainment by some phase maps in a direction that is opposite to that of other phase maps [13].
Jet lag affects the health status of frequent air travelers. The disruptive effect of jet lag has been documented in experimental animals at the molecular level of clock genes in the SCN and in the clock genes present in peripheral tissues [19]. Eastbound travel causes a phase advance in all the body’s circadian rhythms, whereas westward flight has the opposite effect, i.e., it produces a phase delay. Consequently, travelers tend to synchronize their bodily rhythms at a speed of 1.5 h a day after westward and 1 h a day after eastward flight, irrespective of whether they travel during the day or at night. This difference in adjustment time is usually attributed to the greater ability of the internal body clock to adapt to a longer rather than to a shorter day (τ longer than 24 h, Chap. 2).
Jet-lag disorder falls under the category of circadian rhythm sleep disorders. The symptomatology of jet-lag disorder includes both physiological and psychological disturbances (Table 8.2). Several studies suggest that chronic or repeated jet-lag exposure can lead to cognitive decline and temporal lobe atrophy in humans if there is a short recovery time between flights. Model jet-lag disorder is also associated with tumor progression in rodents and elevated mortality rate in aged mice [13].
Jet-lag disorder symptoms show considerable inter- and intra-individual variability. Age is one important factor. In simulated jet-lag disorder, middle-aged male subjects had more symptoms than younger men. Moreover, those over 60 years are reported to have greater difficulty in adapting to jet-lag disorder [20]. Another important variable is the individual’s “chronotype” (Chap. 2). Individuals who are “morning chronotypes” generally have less difficulty in phase-advancing their body rhythms (i.e., adjusting after a flight from west to east) than “evening chronotypes,” and vice versa in the case of a phase delay. Generally, those who had “rigid” sleep habits had more severe symptoms after a transmeridian flight [21].
Several studies have examined the effects of simulated and real jet lag on physiological and psychological variables in different populations including aircrew members, and have confirmed that these frequent flyers suffer marked sleep–wake problems because of jet lag. For example, in a 2-year collaborative field study of Spanish pilots flying the routes from Madrid, Spain, to Mexico City, Mexico (−7 time zones) or from Madrid to Tokyo, Japan (+8 time zones) we used telemetry to record pilots’ activity, temperature, and heart rate [22, 23]. Subjective time estimation and other psychological variables such as anxiety, tiredness, and performance were recorded. Urinary 6-sulphatoxymelatonin and cortisol excretion (determined in 6-h intervals) were also measured. Activity/rest and heart rate rhythms, linked to a “weak” or exogenous oscillator, became rapidly synchronized, whereas temperature or 6-sulphatoxymelatonin excretion rhythms, which are closely regulated by the biological clock (Chap. 5) showed a more rigid response after the phase shift of the light/dark cycle [22]. In both young (<50 years old) and old (>50 years old) pilots arriving in Mexico or Tokyo, the activity/rest rhythm rapidly adjusted to the new schedule, whereas the acrophase of the temperature rhythm tended to fluctuate near the original temporal zone. This desynchronization was evident until the return flight (day 5) and persisted after arrival in Madrid [22]. The sequelae of desynchronization were less tolerable in older than younger pilots. Skin temperature rhythm did not become entrained neither on reaching Tokyo nor after the return flight to Madrid in the group of older pilots [22]. The changes in urinary 6-sulphatoxymelatonin and cortisol excretion were consistent with these conclusions.
Systematic and incorrectly planned work schedules of airline pilots produce a chronic disruptive condition, a higher incidence of stress-related emotional changes, and a diminished life expectancy. The optimal work strategy for this population is a compromise between two extreme possibilities: a long rest period at stopovers until full re-entrainment is achieved, or a short stop accompanied with relative isolation, maintaining the original “home” local habits to prevent re-entrainment. With the first strategy, aircrew would be systematically exposed to a re-entrainment process, with a permanent disruption to the circadian system, whereas the second approach, although less disturbing to the body clock, would probably not allow pilots to have the necessary rest and alertness for the return flight [24].
Sleep deprivation produces an allostatic overload that can have deleterious consequences [25]. Restriction of sleep to 4 h per night is associated with increases in BP pressure, decreases in parasympathetic tone, increases in evening cortisol and insulin levels, and increases in appetite through the elevation of ghrelin, a pro-appetitive hormone, and decreases in the levels of leptin, which has anorexic activity (Chap. 5). Proinflammatory cytokine levels are also increased, along with decreases in performance in tests of psychomotor vigilance after a modest sleep restriction to 6 h per night. Allostatic overload in animal models causes atrophy of neurons in the hippocampus and prefrontal cortex, the brain regions involved in memory, selective attention, and executive function. It also causes hypertrophy of neurons in the amygdala, the brain region involved in fear and anxiety, and aggression (Chap. 6). Thus, the ability to learn and remember and to make decisions may be compromised and may be accompanied by increased levels of anxiety and aggression.
Both long-term and short-term exposure to transmeridian flights have an impact on cognitive functioning. For example, in a group of individuals who were on a transmeridian flight and who underwent functional magnetic resonance imaging study, participants from the jet-lag group presented decreased activation in the bilateral medial prefrontal and the anterior cingulate cortex [26]. The results are suggestive of a negative impact of jet lag on important cognitive functions such as emotional regulation and decision-making during the first few days after individuals arrive at their destination.
Shift-Work Disorder
A wide range of work schedules is referred as “shift work.” They include occasional on-call overnight duty, rotating schedules, and steady, permanent night work (Fig. 8.5) [6]. Owing to the overlap of these categories, it is difficult to generalize about shift work disorder. Over 10% of night workers and of rotating workers met the minimal criteria for shift work disorder.
Several studies have now confirmed that there is a relationship between cardiovascular disease and shift work (Fig. 8.6). Shift workers are at a 40% higher risk of developing ischemic heart disease. The association between shift work and metabolic syndrome, a major risk factor for cardiovascular disease, also occurs. Alternating shift work has been reported to be a significant independent risk factor for high BP, an effect that was more pronounced than that of age or body mass index [6].


Fig. 8.6
Potential physiopathological pathways by which shift work may lead to cardiovascular disease and cancer. Experimental circadian misalignment and sleep restriction protocols disrupt and enhance the activity of neuroendocrine stress systems, reduce immune defense (NK cells), and cause inflammation and oxidative damage. Inter-individual vulnerability to the adverse effects of sleep restriction and circadian misalignment contribute to a heterogeneous tolerance to shift work. Prophylactic naps could blunt the stress response, possibly correct stress-dependent immune changes, and improve the recovery of immune homeostasis. Reproduced with permission from Cardinali [75]
In 2007, the International Agency for Research on Cancer classified shift work as a probable human carcinogen (2A). Women who work on rotating night shifts are reported to be at a moderately increased risk of breast cancer after extended periods of working night shifts [27], as are female cabin crew [28]. Because melatonin has oncostatic effects, including effects on estrogen and fat metabolism, it may play a role in both breast and endometrial cancer. Light exposure at night reduces melatonin levels, but the role of melatonin in both of these cancers remains to be defined.
Misalignment between the circadian pacemaker and the timing of sleep, wake, and work occurs in shift workers, and shift work disorder, with insomnia, reduced sleep, and excessive sleepiness, is common. All these impair cognitive function, alertness, and mood, and increase the risk of accidents. For years, the public, media, and regulatory authorities have blamed the effects of excessive speed and alcohol as the main causes of road accidents. However, it is important to note that the lack of sleep produces the same effects on the ability to drive a vehicle as drinking alcohol. In psychometric studies, to be awake for 17–18 h disturbs the ability to drive a vehicle in a similar manner to the effect of an alcohol concentration in the blood of 0.05 g/dL [29]; moreover, both situations may add up to decreased attention. Between 20 and 25% of road accidents are caused by fatigue and sleepiness of drivers, being more frequent between 0200 and 0800 h. This trend is particularly evident on motorways and monotonous routes.
In several studies on this subject, high number of drivers refer to often being drowsy at the wheel. Clearly, there are more sleepy drivers than drunk ones on roads. For example, in representative samples of public transportation drivers in the Metropolitan Area of Buenos Aires and long-distance drivers covering various geographical corridors of the country, we conducted surveys on health and working conditions and applied objective measures of physiological variables, including evaluation of the sleep/wake rhythm using actigraphy, circadian rhythmicity by the peripheral rhythm of body temperature, alertness by determining psychomotor response to a stimulus, autonomic activity by heart rate variability, and endocrine response to stress by measuring cortisol in the saliva [30, 31].
In short-distance drivers, a high prevalence of work-related stress, overweight, obesity, physical inactivity, and hypertension was observed. The quantity and quality of sleep on weekdays was poor, with partial recovery at the weekend, a high frequency of daytime sleepiness, and high risk of apnea. The neurohormonal weekday pattern was consistent with stress and a significant drop in psychomotor performance was observed during working hours, especially the morning shift [30, 31].
In long-distance drivers, a high prevalence of cardiovascular risk factor s, such as overweight, physical inactivity, and smoking, was also found. Sleep patterns of poor quality, with little sleep at home, while traveling, and at the destination, and a decrease in amplitude circadian rhythms, were observed. The pattern was consistent with high cortisol levels, with little recovery in the days out of work, and a decrease in alertness at the end of the return trips [30, 31].
In addition to the known effects on sleep, eating patterns, and alterations in social life, gastrointestinal disorders are very common in shift workers. The association between rotational work shifts and gastrointestinal disorders has several causes. Irregular ingestion habits of workers affect the synchronization of numerous circadian rhythms, in particular, those related to digestive functions and metabolism [32]. Gastrointestinal disorders may be due to ingestion of food at the “wrong” times, which induces anomalous patterns of motility and digestive secretions. In addition, the absence of hot food, which occurs frequently during the night (with a predominance of snacks), a high carbohydrate intake, caffeine and alcohol, and high consumption of tobacco have all been proposed as causes of gastrointestinal disorders in shift workers.
Chronodisruption
This term defines the changes in amplitude and phase of circadian rhythms that are found in acute or chronic illness, even in mild situations such as common flu (“poor wakefulness, poor sleep”) [14–17]. The clock itself, the information pathways from synchronizing agents to the clock or the efferent pathways of the clock can be affected alone or conjointly (Fig. 8.4). The deterioration of circadian rhythms with age is an example of a change with many components, which implies a decrease in the effectiveness of many aspects of the circadian system, from a reduced influence of the synchronizers, to a deterioration of the clock itself, to a decrease in the ability to obey the clock output signal [33].
The observation that the circadian system is not functioning normally does not necessarily imply that it is the primary cause of the alteration. Rather, the primary defect may have originated elsewhere, and its effects on the circadian system be one of the many changes it causes. In this case, and although treating the circadian system could improve the individual’s nocturnal sleep and diurnal activity, we would not be addressing the real cause of the problem. A combined (specific and chronobiological) treatment is needed for full recovery (Figs. 8.7 and 8.8) [14–17].



Fig. 8.7
The concomitant chronodisruption occurring in most acute or chronic diseases must be adequately treated to obtain full recovery of health. Reproduced with permission from Cardinali [75]

Fig. 8.8
Exposure to light in the morning and the administration of melatonin in the evening provide a strong synchronization signal and an increase in the amplitude of sleep/wake cycle. From clinical standpoints, the changes in amplitude (“poor sleep together with poor vigilance”) is a paramount sign of the disease and its correction increases substantially the patient’s quality of life. The morning light and melatonin in evening hours are the natural resources to restore proper rhythmicity of sleep/wake rhythm. Reproduced with permission from Cardinali [75]
Chronobiological Treatment of a Desynchronized ANS
The jet lag and the alterations generated by shift work are two situations that reflect the functioning of a circadian system of a normal subject that has not adjusted to a schedule change of the sleep/wake cycle. Consequently, the treatment of these problems consists in the use of alternative zeitgebers that allow the adjustment of the circadian clock to the new schedule, or to avoid the adjustment in situations where it is unnecessary, as in rapid rotation shifts, or when the return flight takes place as soon as within 1 or 2 days. On the other hand, the treatment of clinical problems associated with circadian rhythm alterations needs both specific therapies and an adjuvant chronobiological treatment to obtain an optimal result (Figs. 8.7 and 8.8).
To successfully overcome the effects of jet-lag disorder, adjustment to the new time zone can be encouraged by adopting the social timing of life in the new time zone as soon as possible. In field and simulation studies suitably timed melatonin administration has been shown to accelerate phase shifts and to significantly improve self-rated jet-lag disorder symptoms in large numbers of time zone travelers. In addition, exposure to light has been shown to accelerate phase shifts. The combination of melatonin and light exposure, one in the evening and the other in the morning, is more effective than either treatment alone (Fig. 8.7) [20].
The effect of light or melatonin on the circadian system can be measured by a phase response curve in which the minimum in core body temperature is used as an estimate for the crossover point of the curve (Fig. 8.9). Light pulses administered before this point delay the circadian clock, whereas light pulses after it phase advance the clock. Light exposure close to the minimum core body temperature produces the greatest phase shifts [34]. Phase delays of approximately 2.5–3 h per day and phase advances of 1.5–2 h per day have been observed following carefully timed exposure to bright light [35]. The phase response curve for melatonin is the opposite to that of the light, although not symmetrical (Fig. 8.9).


Fig. 8.9
The phase response curves to light and melatonin are opposite, but not symmetrical. Reproduced with permission from Cardinali [75]
Melatonin is the prototype of a chronobiotic drug [36]. It is produced in most organisms from algae to mammals, but its role varies considerably across the phylogenetic spectra [37]. In humans, melatonin plays a major function in the coordination of circadian rhythmicity, remarkably the sleep–wake cycle (Chap. 2). Melatonin secretion is an “arm” of the biological clock in the sense that it responds to signals from the SCN. More particularly, the timing of the melatonin rhythm indicates the status of the clock, both in terms of phase (i.e., internal clock time relative to external clock time) and amplitude. From another point of view, melatonin is a chemical code of the night: the longer the night, the longer the duration of its secretion. In many species, this pattern of secretion serves as a time cue for seasonal rhythms (Chap. 2).
The usefulness of melatonin for ameliorating the symptoms of jet lag has been compellingly demonstrated in numerous investigations. A meta-analysis (Cochrane database) concluded that melatonin taken at bedtime in the place of destination (2200 h to midnight) was effective for decreasing the jet lag symptoms in air travelers who crossed five or more time zones [38].
There is considerable evidence that a light stimulus of sufficient intensity applied at a critical circadian phase can essentially stop the human circadian clock by resetting the circadian oscillator close to a phaseless position at which the amplitude of circadian oscillation is zero, i.e., type 0 resynchronization (Fig. 8.10) [39]. Indeed, exposure of humans to cycles of bright light, centered on the time at which the human circadian pacemaker is most sensitive to light-induced phase shifts, can markedly attenuate or reduce endogenous circadian amplitude.


Fig. 8.10
To suppress the circadian clock oscillation, the application of symmetrical light pulses in the first and second part of the night is needed. In this “knock out” of the circadian clock, melatonin administration at the local time has an immediate effect of synchronization to the new time schedule. Reproduced with permission from Cardinali [75]
Timed light and melatonin administration allowed an almost immediate resynchronization of circadian rhythms in a group of jet air travelers who had made a transmeridian flight over 12 time zones. Under the conditions of transfer of 12 time zones over a period of hours, a fully inverted (180°) relationship between the subjective day and the geophysical day occurs. Thus, a patterned exposure to natural light covering portions that symmetrically delay and phase advance the circadian rhythms resulted in suppression of the circadian pacemaker function. This allowed the use of melatonin at local night to resynchronize the circadian oscillator to the Tokyo time. Additionally, we administered a nonphotic stimulus (exercise) in a schedule to coincide with exposure to natural light to mask the circadian oscillator. The observed rate of resynchronization was about 2 days, significantly different from a minimum resynchronization of up to 8–10 days expected after a flight through 12 time zones [40, 41].
A positive correlation was found between the pre-flight melatonin production rates, evaluated by measuring urinary 6-sulphatoxymelatonin excretion, and sleep quality and morning alertness after a flight [40]. It is known that individuals who possess a weak circadian time structure, as revealed by the low amplitude of body temperature rhythm, are more prone to developing biological intolerance to shift work [42] and presumably to jet lag. In Table 8.3 the tentative recommendations for flights through >8 time zones are summarized.
Among the guidelines for the effective management of shift-work disorder, organizational level changes are important. Three types of intervention have been recommended: (a) switching from slow to fast rotation; (b) changing from backward to forward rotation; (c) self-scheduling of shifts [6]. There is evidence that a rapidly rotating schedule is less detrimental as it minimizes the time spent in a desynchronized state. Clockwise rotation, rather than counterclockwise rotation, was reported to be preferred by workers, probably because the body clock period is somewhat longer than 24 h. Longer duty shifts allow more time off work. It is possible that a flexible approach is best because of major individual differences in workers. The goal is to achieve at least 7 h of sleep per 24 h. Melatonin treatment before daytime sleep is used to promote sleepiness and to induce phase shifting.
Figure 8.11 summarizes the common situation of a night worker working a 5-day night shift [43]. The minimal central body temperature must be shifted toward daytime sleep by using bright light during the first part of the work period and wearing dark goggles on the commute home. Melatonin (3–6 mg) before daytime sleep causes sleepiness and phase shifting. Planned 30-min napping just before or on the job combined with caffeinated drinks reduce sleepiness and improve alertness while working. The wakefulness-promoting agents armodafinil and modafinil have been approved by the Food and Drugs Administration (FDA) for the treatment of excessive sleepiness in patients with shift-work disorder.


Fig. 8.11
The common situation of a night worker working in a 5-day night shift is depicted. The minimum central body temperature must be shifted toward daytime sleep to ensure a recovery sleep at home. (a) Light and activity at night cause a phase delay that is counteracted by sunlight on return home. Although light and physical activity during the night work shift cause some phase delay, light on the commute home opposes this process (causing phase advance). (b) Periods of bright light progressively longer in the first part of the night shift give a phase delay that is sustained by using dark glasses on the return home and by taking melatonin before sleep at home (melatonin treatment before daytime sleep is useful for causing sleepiness and inducing phase shifting). Planned 30-min napping just before or on the job combined with caffeinated drinks reduce sleepiness and improve alertness while working [43]
In conclusion, both jet-lag disorder and shift-work disorder share a similar cause and management has major similarities. In both cases, three factors are important: (a) sleep scheduling; (b) resetting the body clock with light and/or chronobiotics; (c) use of drugs to promote wakefulness if needed.
For the treatment of clinical chronodisruption it is important to note that the primary alteration may have originated elsewhere, and its effects on the circadian system are one of the many changes it causes. In other words, a chronobiological treatment could be effective because it fights some of the symptoms of the disorder rather than the disorder itself. These warnings are of little importance to those who are limited to treating patients, but they pose a problem of interpretation for those who wish to understand the substance of the problem and try to develop a more rational treatment [14–17]. The combination of the specific and chronobiological treatment is needed for full recovery (Figs. 8.7 and 8.8).
Subjects suffering from insomnia of various causes usually take melatonin, administered 1 or 2 h before the time when it is desired to sleep. In addition to insomnia associated with jet lag and shift work, melatonin is also effective in insomnia of the (otherwise healthy) elderly, in patients with senile dementia, in blind subjects, in subjects who can see but present free-course rhythms, and in patients with delayed-phase sleep syndrome. Its efficacy is demonstrated in both objective and subjective sleep time estimates and in the objective measurement of actimetry. In a meta-analysis including 19 studies and involving 1683 subjects, melatonin showed significant efficacy in reducing sleep latency and increased total sleep time [44]. Trials of longer duration and the use of higher doses of melatonin demonstrated greater effects. Several consensus statements encourage the use of melatonin to treat insomnia [45].
On average, humans cannot do well without sleep for more than a few days (about 2 or 3 days). With only 24–48 h of sleep deprivation, failure of short-term memory appears, there is an increase in the feeling of fatigue, sleepiness, and aggression, and a depressed mood. After 72–98 h without sleep, fatigue is severe and episodes of mental confusion and distortion may occur; in certain individuals, sleep deprivation may produce persecutory delusions.
To solve sleep deprivation, there is no choice but to sleep. In general, after sleep deprivation, we recover one third of the total lost sleep time, 100% of slow-wave sleep and 30–50% of REM sleep [46]. Therefore, the estimated 10 h of sleep deficit accumulated during the week if we sleep 2 h less than needed daily can be recovered by sleeping 1.5 h more on Saturday and Sunday. Hence, the extraordinary importance of not using strict schedules for sleep at the weekend and let it flow without using an alarm clock.
Some Clinical Autonomic Entities Associated with a Desynchronized ANS
Metabolic Syndrome
In the present world, food has become abundant and simultaneously, the need for physical effort has been greatly reduced. From an evolutionary perspective, this is another “environmental mutation,” that, together which the advent of artificial lighting, has greatly contributed to the loss of the experience of a differentiated day and night. Chronodisruption, with a disturbed balance among the three different configurations of organ and system regulation in a 24-h cycle, is a direct consequence of this (Fig. 8.2).
Obesity has a profound impact on health, such as T2DM and hypercholesterolemia, mainly through its influence on secretion and insulin sensitivity. The metabolic syndrome comprises a group of metabolic abnormalities (hyperinsulinemia, insulin resistance, hypertension, obesity, hyperlipoproteinemia, hypertriglyceridemia) that increase the risk of cardiovascular disease and T2DM. The metabolic syndrome is also associated with an increased risk of nonalcoholic fatty liver disease and renal dysfunction. Similarly, there is evidence for the correlation of metabolic syndrome with dementia (“type 3 diabetes mellitus”) and with cancers, mainly of the breast, pancreas, and bladder [47].
There is impressive information indicating that the obesity is associated with low-grade inflammation of the white adipose tissue, which can subsequently lead to insulin resistance, impaired glucose tolerance, T2DM, and type 3 diabetes [48, 49]. Adipocytes actively secrete proinflammatory cytokines such as TNF-α, IL-1β, and IL-6 and trigger a vicious circle that leads to additional weight gain, largely as fat (Fig. 8.12). Increased circulating levels of C-reactive protein and other inflammatory biomarkers also support the occurrence of inflammation in obesity.


Fig. 8.12
The metabolic syndrome is the consequence of obesity-induced changes in adipokine secretion that lead to the development of systemic insulin resistance, T2DM, type 3 diabetes, and cardiovascular disorders. Overnutrition that results from a combination of increased food intake and reduced energy expenditure leads to adipose tissue expansion, increased adipocyte size and number, and increased macrophage infiltration that, together, lead to increased free fatty acid release, dysregulated secretion from adipocytes of a variety of adipocytokines, including adiponectin, leptin, and resistin, and increased release from resident macrophages of the inflammatory cytokines (TNF-α, IL-6). Dysregulated secretion of these adipokines elicits a variety of adverse effects on numerous tissues and leads to the development of systemic insulin resistance that increases the risk for development of the metabolic syndrome, a variety of cardiovascular disorders, and T2DM and type 3 diabetes (when combined with dementia). Reproduced with permission from Cardinali [75]
The altered production of proinflammatory cytokines modulate adipocyte size and number through paracrine mechanisms that exert an important role in the regulation of fat mass (Fig. 8.12). The amounts of proinflammatory molecules derived from adipose tissue in obese patients diminishes after weight loss [50]. Therefore, the fat cells are both a source and a target for TNF-α, IL-1β, and IL-6 (Fig. 8.12).
A number of factors play an unequivocal role in increasing the risk of developing T2DM [51]. These factors include dysfunction of pancreatic β cells, abnormal adipogenesis and absence of adequate insulin responsiveness in the liver, genetic susceptibility, physical inactivity, excessive food consumption and/or high calorie food intake, and a sedentary life-style.
In addition to these predisposing factors, there is now an increasing amount of evidence that disruption of circadian timing mechanisms is a major contributor to the development of T2DM [49]. Various types of circadian disturbances have been correlated with T2DM, including disruption of the timing of bodily functions that are normally synchronized, improper timing of food intake, dampened clock gene expression and polymorphisms, sleep loss/disturbance and impairments of the melatonin signaling pathway [52].
In a study on 593 patients with a recent diagnosis of T2DM, sleep debt resulted in long-term metabolic disruption, which may promote the progression of the disease [53]. Sleep quality rather than sleep duration played an important role in insulin resistance in these newly diagnosed T2DM patients [54]. The increased obesity rate recorded in 100,000 women of the Breakthrough Generations Study, associated with increased levels of light at night exposure, supports this assumption [55].
Glucose metabolism is among the numerous physiological functions that are governed by the circadian apparatus [56]. It is known that the distribution of glucose to all parts of the body is organized by the molecular clock present in liver. Circadian regulatory mechanisms utilize both neural and humoral communication to exert close control over insulin, leptin, and plasma glucose levels (Chap. 2).
Direct evidence for the association between circadian clock disruptions and T2DM has been provided by studies in mice in which linkages between clock gene mutations and diabetes states were examined [57]. Per2 mutant mice show several abnormal profiles, including the absence of rhythmicity in plasma glucocorticoid levels, obesity, and low levels of neuropeptides involved in appetite regulation. Clock gene mutations promote delays in pancreatic gene expression, which in turn affects the regulation of the growth, development, and survival of insulin cells and thus of the glucose signaling pathway [58]. Clock-mutant mice showed a lack of rhythmicity in the action of insulin, a state that was reversible once the clock protein had been reintroduced [59].
Additional confirmatory evidence for circadian control over metabolic activity was provided by a study of mice in which the Bmal1 gene was specifically knocked in the pancreas. This produced an animal model that replicated T2DM in that blood glucose levels were found to be elevated throughout the 24-h cycle [58].
In diabetes-prone, genetically engineered rats that were maintained in continuous light and jet-lag-like conditions, circadian disruption ensues [60]. Similar findings were obtained in a study of humans subjected to a forced desynchronization protocol for a period of 28 h. The treatment produced circadian misalignment in all subjects, with 30% of individuals exhibiting a disturbed glucose metabolism that resembled diabetes [61]. Also, all subjects showed a decreased concentration of leptin, a reversal of cortisol rhythm, and high amounts of glucose (postprandial) even in the presence of increased insulin. Additionally, the increased levels of cortisol late in the day, i.e., during the end of wakefulness, were a potentiating factor for insulin resistance and hyperglycemia [59]. These findings suggest that the misalignment of clock functions accelerates the development of T2DM (Fig. 8.13).


Fig. 8.13
As a disruption of the timing of bodily functions that are normally synchronized such as the sleep/wake cycle, improper timing of food intake, metabolism, and dampened clock gene expression occur. Metabolic dysfunction leads to the metabolic syndrome. Reproduced with permission from Cardinali [75]
Light exposure at night, even at low levels, has been reported to alter food timing and body mass accumulation, thus suggesting that artificial lighting is an important contributing factor to the increased prevalence of metabolic disorders [56]. As discussed in Chap. 5, circadian rhythms could be entrained by manipulating the timing of food administration, and that this could be accomplished without the participation of the SCN (Fig. 5.33). Taken together, the data indicate that the administration of food at inappropriate times may disrupt the metabolic profile, thus creating a desynchronized physiological state that is causally linked to the development of T2DM (Fig. 8.13).
The possible association between clock gene polymorphisms and T2DM has been explored in humans. In one study a polymorphic allele was identified in Cry2 that correlated with T2DM [62]. Two Bmal1 variants have been found to be associated with diabetes and hypertension in a British population [63]. In a study to examine the existence of Per3 variants in patients with T2DM we reported that, compared with the group without diabetes, the frequency of the occurrence of the five repeat alleles of Per3 among affected patients was greater, and that of the four repeat alleles was less [64]. Circadian clock variants have also been found to correlate with body mass index [65], and with weight loss, sleep duration, and total plasma cholesterol in obese Caucasian individuals [66]. Clock and Cry1 polymorphisms are involved in individual susceptibility to abdominal obesity in a Chinese Han population [67]. Single nucleotide polymorphisms in Bmal2 gene have been associated with a high risk of developing T2DM in obese patients [68]. Cross-sectional studies have reported associations between the clock gene polymorphisms and the prevalence of obesity, plasma glucose levels, hypertension, and T2DM. The association of the Clock polymorphism and stroke in T2DM was reported, indicating that core clock genes significantly contribute to increased cardiovascular risk in T2DM [69].
A high-fat diet that contributes to insulin resistance, impaired glucose metabolism, and obesity, can feedback to influence the biological clock. Rats on a 35% fat diet exhibited a disrupted 24-h rhythmicity of Per1, Per2, Cry1, and Cry2 expression, the Per2 expression profile being almost inverted by the high-fat diet (Fig. 8.14) [70]. These results indicate that the inherent transcription, translation, and post-translational modifications that give the clock its own natural rhythmicity are disrupted in obese rats. The current evidence thus suggests that these processes might have a reciprocal relationship, as an abnormal functioning of metabolism promotes altered expression of the clock genes, whereas impaired clock functioning can disrupt metabolic activity. Indeed, in T2DM patients, clock gene expression was directly associated with fasting glucose levels and with insulin mRNA and protein concentration [71].


Fig. 8.14
Circadian oscillating expression of clock genes is sensitive to a high fat diet. In this experiment, rats were fed a 4% (control) or a 35% fat diet for 11 weeks. Wistar male rats (n = 6–8 per group) were killed by decapitation at six different time intervals throughout a 24-h cycle. Adenohypophysis was collected and RNA extracted. The means ± SEM of mRNA expression by real-time PCR (**p < 0.01, *p < 0.05 compared with control rats in a Student’s t test). Results from Cardinali et al. [70]. Reproduced with permission from Cardinali [75]
In a recent survey, samples of subcutaneous adipose tissue from 50 overweight subjects were collected before and after an 8-week administration of a hypocaloric diet. The expression of core clock genes, Per2 and NR1D1, increased after the weight loss, and their levels correlated with the expression of several genes involved in fat metabolism [72]. Another study demonstrated that a weight loss intervention based on an energy-controlled Mediterranean diet influences the methylation levels of three clock genes, Bmal1, Clock, and NR1D1, being an association between the methylation levels and the diet-induced serum lipid profile [73]. Thus, an abnormal functioning of metabolism promotes the low expression of clock genes, whereas impaired clock functioning can disrupt metabolic activity. Figure 8.15 summarizes the factors and their output that may be responsible for T2DM. The findings thus underscore the importance of circadian regulation for normal metabolic functioning, and further, that clock gene expression appears to be disturbed in T2DM (Fig. 8.16).



Fig. 8.15
Adapted fishbone diagram including the factors and their output responsible for type 2 diabetes mellitus

Fig. 8.16
A hypothetical distribution of circadian alterations in organs and tissues in the metabolic syndrome . Data are extrapolated from studies in transgenic mice that have provided evidence of the association between circadian clock disruptions and metabolic and behavioral events in metabolic syndrome. Reproduced with permission from Cardinali [75]
The possibility that a relationship might exist between melatonin and T2DM is supported by findings that insulin secretion is inversely proportional to plasma melatonin concentration. It is known that melatonin can alter insulin function and that, compared with normal healthy individuals, T2DM patients have lower circulating levels of melatonin. In vitro co-incubation of pancreatic cells with melatonin has been shown to inhibit the glucose-mediated release of insulin, additionally supporting the conclusion that melatonin activity plays a role in the function of insulin. Further, circadian disruption has been shown to alter melatonin secretion and to cause dysfunctions in pancreatic β cells. Genetic association studies that have shown that mutations in the melatonin receptor gene correlate with an increased susceptibility to T2DM [74].
It can be postulated that the alteration of phase, amplitude, and synthesis of melatonin could lead to impaired glucose metabolism in circadian disrupted individuals. Suppression of melatonin secretion by nocturnal light exposure could be a crucial factor for T2DM development [56]. In this respect, the successful management of T2DM may require an ideal drug that besides antagonizing the triggers of T2DM, also corrects the disturbed sleep–wake rhythm.
Melatonin, together with morning light, is an interesting chronotherapeutic option that can reset the phase and amplitude of circadian rhythms in T2DM patients. The combination of the specific and chronobiological treatment is needed for full recovery (Figs. 8.7 and 8.8). As has been shown in animal models of diabetes and obesity, melatonin also possesses cytoprotective properties that may prevent a number of unwanted effects in T2DM [75]. The efficacy of melatonin treatment for treating metabolic and cardiovascular comorbidities in T2DM has been reported (Fig. 8.17) [76–81]. However, melatonin treatment was reported to impair insulin release in humans who carry MTNR1B, the risk allele of the MT2 receptor gene [82]. To what extent insulin resistance is ameliorated by melatonin in these patients remains to be defined. At an early stage of T2DM treatment, nonpharmacological approaches such as lifestyle modification, low-fat diet, and exercise are recommended [52].


Fig. 8.17
Effects of melatonin in the metabolic syndrome . Melatonin normalizes high blood pressure and circulating indexes of inflammation. It improves insulin sensitivity and restores disrupted circadian rhythms. Reproduced with permission from Cardinali [75]
Mental Illnesses
The human species is very vulnerable to psychiatric diseases: 1% of the population suffers from schizophrenia, 4% from severe uni- or bipolar depression, and 15% of some form of reactive emotional illness in isolated episodes. There are epidemiological elements to suspect a genetic predisposition in the appearance of several of these diseases.
Emil Kraepelin, at the beginning of the twentieth century, was the first to develop a modern classification of mental illness, differentiating “precocious dementia” (schizophrenia) from the more benign and recurrent forms of illness (“manic disease”). Following Kraepelin, cognitive alterations (“disorders of thought” such as schizophrenia) and affective disorders (“emotional disorders” or “affective diseases” such as depression, manic depression, and anxiety) have been identified [83].
Emotional illnesses are characterized by an abnormality of emotional and expressive experiences that constitute affectivity. Normally, a person’s affectivity is in neutrality, with mild episodes of euphoria (called “happiness”) and mild episodes of sadness (called “unhappiness”). When one trespasses these limits, one enters emotional pathology. The pathological end of euphoria is called “mania ”; The pathological end of unhappiness is called “depression .” When individuals express both abnormal ends in a single episode or at different times throughout their life, they are diagnosed with bipolar disease type 1 or 2 (depending on the intensity of the manic phase).
Mania is defined by the presence of an abnormally expansive and irritable affectivity, coupled with several related symptoms. They include decreased need for sleep, excessive loquacity, changing thoughts, grandiosity, easy distraction, and increased activities with pleasurable objectives. This has important consequences: non-normal sexual behavior, risky economic ventures, etc. A maniac patient is often enthusiastic and positive, but tolerates little frustration. Because mania is not an unpleasant experience, patients rarely admit to being treated. However, relatives or close friends recognize this situation as dangerous for the patient. Ultimately, the persistence of the patient in the manic state may be accompanied by serious affective or economic breakdown for the patients and their families.
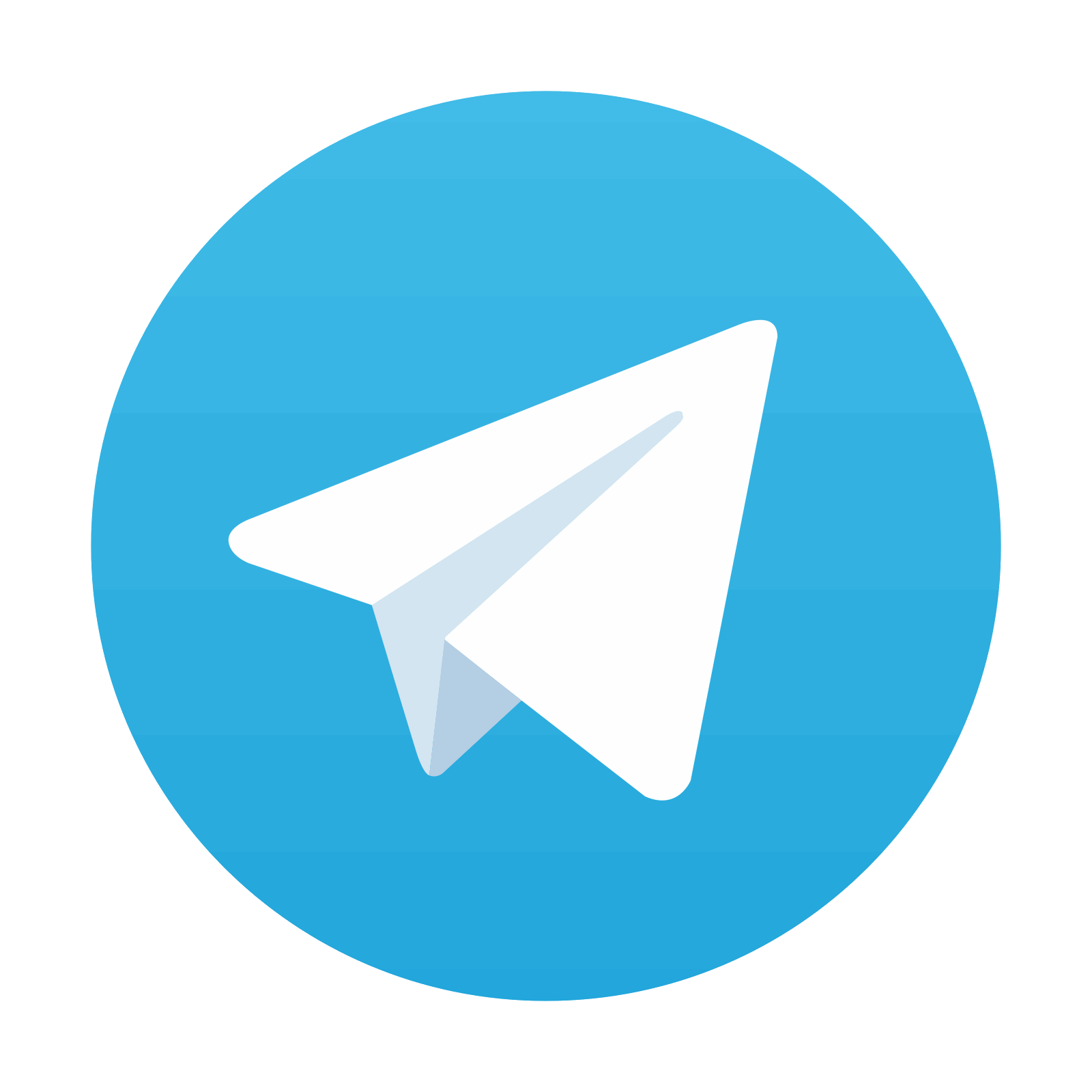
Stay updated, free articles. Join our Telegram channel

Full access? Get Clinical Tree
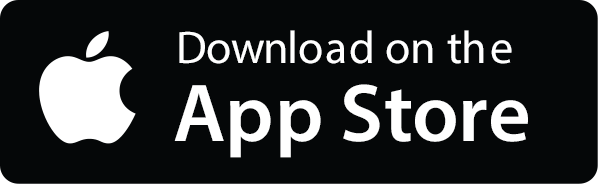
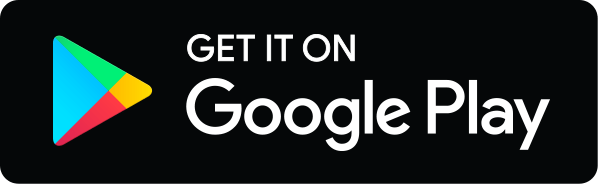