Fig. 11.1
Detection of the transporter VGluT1 in lysates obtained from surgically reinnervated muscle endplates of animals with a positive or negative response to electrical stimulation. In contrast to the non-reinnervated negative control side, where no marker was expressed, brain tissue was positive
11.3.3 Detection of Fast Blue in α-Motoneurons
Using fluorescence microscopy, Fast Blue could be detected within α-motoneurons in the ventral horn of the spinal cord, rostral and caudal to the graft implantation site, as well as in rats that did and did not respond to electrostimulation (Fig. 11.2).
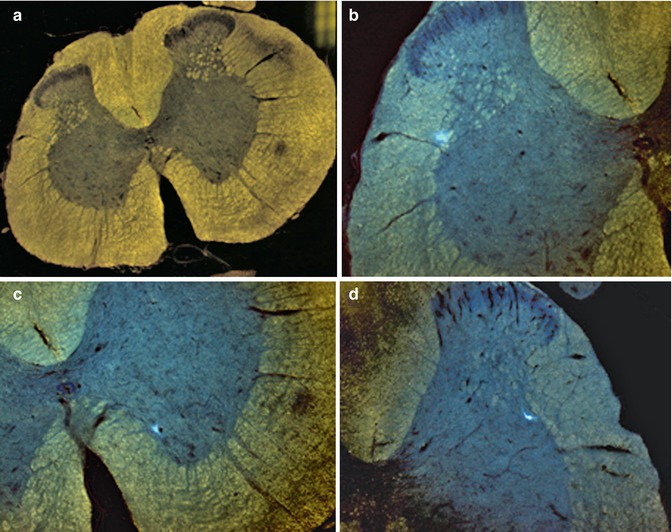
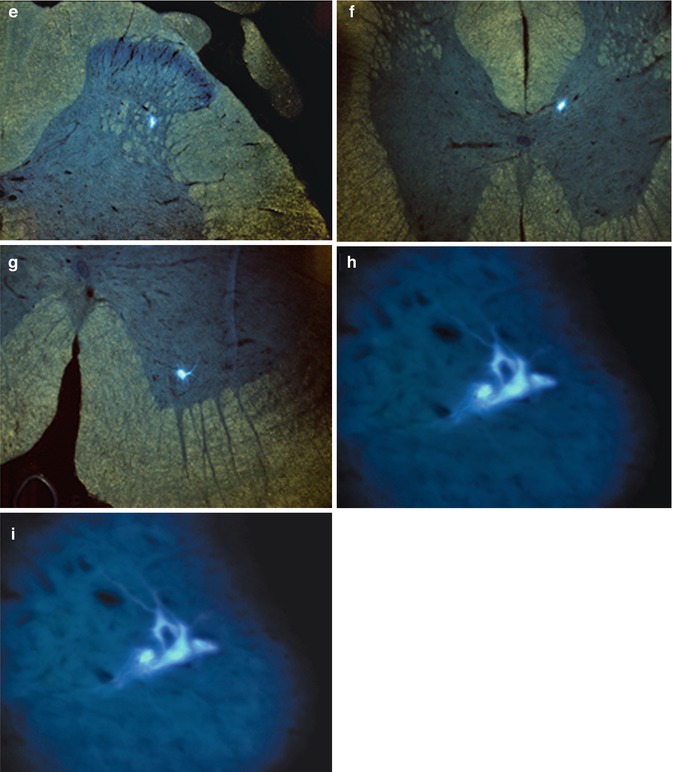
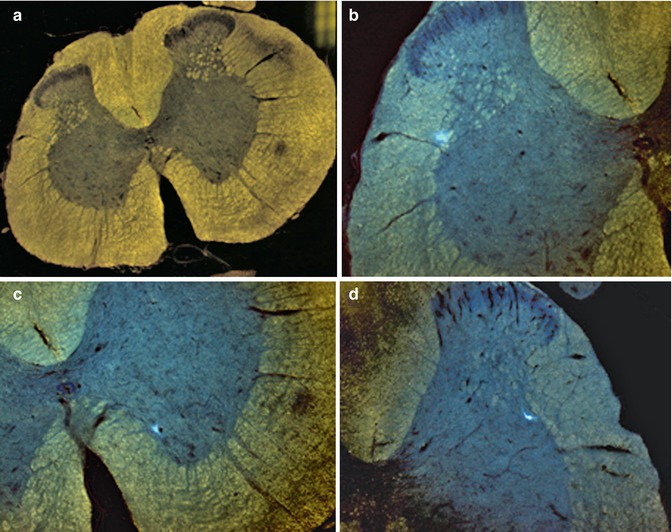
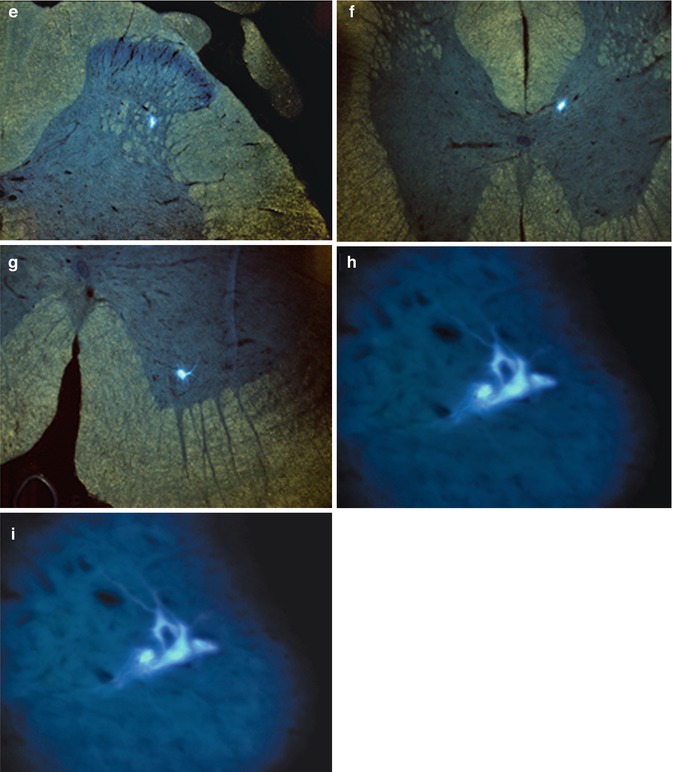
Fig. 11.2
Fluorescence microscopic detection of the neuronal retrograde tracer Fast Blue. Case 13. (a) General view of L1-2 segment of SC; (b) detail from A – FB + neuron in lamina 5/IML of L1-2 segment of contralateral SC; (c) detail from A – FB + neuron in lamina 9 of L1-2 segment of homolateral SC; (d) multipolar FB + neuron in IML of L1-2 segment of SC; (e) multipolar FB + neuron in IML of L1-2 segment of SC; (f) FB + neuron in DNC of L1-2 segment of SC; (g) FB + alpha motor neuron in lamina 9 of L1-2 segment of SC; (h, i) FB + alpha motor neurons in lamina 9 of Th 9-10 segment of SC
11.4 Discussion
Severance of the spinal cord and other traumatic lesions, such as central brachial plexus avulsion, entails a lifetime of disability and dependency for patients, for which no suitable therapeutic intervention exists. For successful regeneration, injured axons must overcome their poor intrinsic growth potential as well as the nonpermissive environment of the glial scar established around the lesion site. As axonal ingrowth into the myelinated cord past the site of the lesion is restricted, multiple approaches have been developed, such as surgical implantation of Schwann cells and olfactory ensheathing cells for bridging [1, 8, 19, 20, 23, 26]. In addition, direct reinnervation of voluntary muscles, by reimplantation of nerve roots [9, 10] or by the use of a nerve graft, to partially supplant the generally nonpermissive environment of the CNS with the more permissive environment of the peripheral nervous system [4], has gained popularity.
Our study is the first to successfully reproduce Brunelli’s complex surgical technique and electrophysiological results, although not all of the animals exhibited a positive response to stimulation (this might be because of the complexity of the regeneration process, including the influence of numerous factors such as plasticity and neuromodulation). Furthermore, the number of animals showing a positive response to electrostimulation (indicative of successful regeneration) might also be affected by the intricacy of the surgical procedure, because transplant placement into the appropriate lamina of the spinal cord, without causing further damage to nerve tissue, requires a great deal of experience (Fig. 11.3). While Brunelli described a complete neurotransmitter switch, from cholinergic to glutamatergic, suggesting the outgrowth of the motoneuron without connection to a second motoneuron at the spinal cord level [5, 24], we interpret the presence of glutamate transporters, in addition to acetylcholine transporters, as indicating glutamatergic/cholinergic co-transmission after reactivation of embryonic potentials [25].
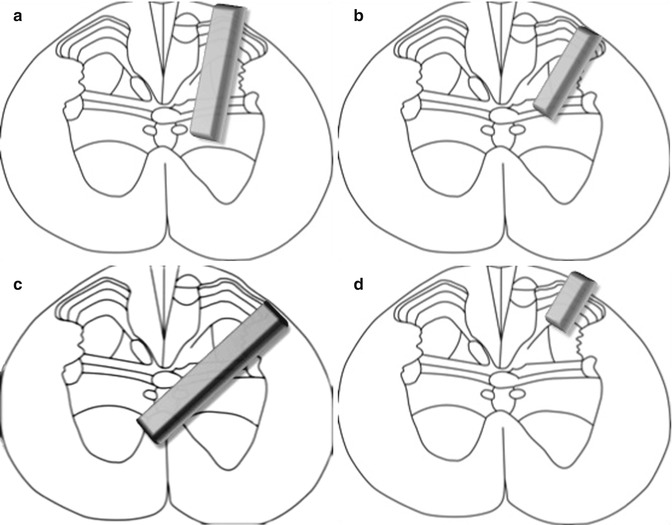
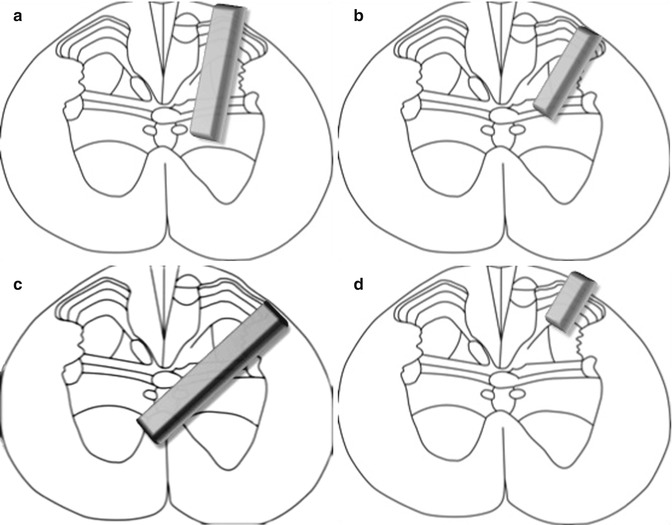
Fig. 11.3
Schematic of the nerve transplant localized in the spinal cord laminae with positive (a, b) or negative response to electrostimulation ((c): to deep and across the midline; (d) to superficial)
The Brunelli Paradigm, including the theory of single-cell plasticity following grafting of the spinal cord to skeletal muscles, enabling voluntary muscle movement without co-contractions, is worthy of further discussion and requires further research for clarification [4]. In this context, detailed analysis of neuromodulation using animal studies is necessary and should be performed in conjunction with functional imaging and corticospinal tract electrophysiological recordings. This will provide additional insight into the biological and morphological changes underlying CNS plasticity and secondary functional neuromodulation following reconstructive procedures for the damaged spinal cord.
Different monomodal pharmacological approaches, i.e., the influence of a single physiopathological pathway in a cascade, have not yet achieved sufficient clinical application [28–30]. However, pharmacological modulation of endogenous defense activity after CNS damage is crucial for obtaining a better functional result [21, 22]. As there is crossover between the different stages after trauma, a multimodal strategy seems more appropriate. Neuropathology after spinal cord damage includes excitotoxicity, inflammation, and apoptosis-like processes. In our opinion, modulation of these processes is the key to efficient neuroprotection [21]. Endogenous defense activity of the nervous system is a continuous process that simultaneously performs and integrates the neurobiological processes of neurotrophicity, neuroprotection, neuroplasticity, and neurogenesis. This activity is under genetic control and attempts to counteract pathophysiological processes and stimulate endogenous recovery. Neurotrophicity denotes a natural biological process by which the continuous effort of the cell maintains correct DNA expression and a normal phenotype. Neuroprotection represents the sum of all of the mechanisms directed against harmful factors and is a short-term endogenous neurobiological process. Neuroplasticity is the permanent adaptation to new functional requirements. This concept describes the ability of the CNS to modulate ongoing processes in response to environmental stimuli, such as learning, new experiences, or injury [18]. Neurogenesis is the process by which new nervous tissue cells, such as neurons, astrocytes, and oligodendrocytes, are formed from stem cells. In a strict sense, neurogenesis is defined as the formation of new neurons. To successfully counteract pathophysiological mechanisms and stimulate recovery, the effect of endogenous defense activity must be pharmacologically enhanced.
It is becoming evident that pharmacological intervention should focus not only on suppression of pathophysiological processes but modulation as well, for a better therapeutic outcome in CNS protection and recovery. While monomodal drugs might modulate a single biochemical or cellular process, they may not significantly improve outcome as various negative factors would remain unaffected. From a pharmacological perspective, it is clear that focusing on molecules that are capable of mimicking the structure and function of endogenous molecules (e.g., neurotrophic factors and related molecules) with multimodal and pleiotropic neuroprotective effects is the best approach in neuroprotection and neuroplasticity. The brain uses the same endogenous molecules (i.e., trophic factors) for both neuroprotection and neuroplasticity, although in different combinations. Neurotrophic factors are the important players involved in brain protection and recovery. Therefore, they are attractive candidates for an integrative, pharmacological therapeutic strategy. These endogenous molecules are activated as a result of altered gene expression induced by lesioning [12]. Cerebrolysin is a nonxsuppressive multimodal drug with pleiotropic neuroprotective effects. It is the only drug available for clinical use containing active fragments of a number of important neurotrophic factors. Its small molecular size allows it to penetrate the blood-spinal cord barrier, thereby permitting systemic application to influence the CNS. It seems to protect neurons from neurodegeneration induced by axotomy [11] and reduces blood-cerebrospinal fluid barrier permeability changes [27]. Cerebrolysin also promotes sprouting of residual axons, enhancing functioning of the remaining neural circuitry and replacing destroyed spinal cord tissue due to demyelination around the injury cavities. Furthermore, it alleviates scar formation and degradation of spinal neuronal function below the level of the lesion (Fig. 11.4) [14–16]. Haninec et al. obtained comparable results after ventral root avulsion of the musculocutaneous nerve, followed by reimplantation into the spinal cord and intrathecal administration of Cerebrolysin [17]. The number of labeled motoneurons, their cell body diameters, and the density of retrogradely labeled axons were significantly increased. Remarkably, axon numbers were threefold higher than in BDNF-treated animals. The improved morphometric features of the regenerated axons were correlated significantly with higher behavioral test scores.