36 The surgical treatment of spinal tumors, both malignant and benign, has evolved dramatically over the last few decades. Primary tumors of the spine are uncommon, with the majority of the cases resulting from metastatic involvement of the spine by a variety of malignant primary tumors. In an extensive review from the Mayo Clinic, primary tumors accounted for only 10% of the cases seen. The treatment of patients with thoracolumbar tumors has evoked conflicting opinions with respect to the primary modality of therapy and indications of surgery.1–7 The surgical management of spinal tumors, which affect the anterior and middle columns of the lower thoracic and lumbar spine, has undergone a continuous evolution over the last few decades. Although the initial surgical procedures were directed to the posterior spine in an attempt to obtain a histologic diagnosis and decompress the spinal cord, these procedures were associated with a significant risk of complications.5,6,8 In recent years, anterior decompression and reconstructive procedures to decompress the spinal cord and assist in anterior load sharing has become an increasingly viable alternative. Many anterior instrumentation devices have been developed that can be classified into either anterior plate-screw or rod-screw systems. Extensive studies using cadaveric or animal tissues have been directed at evaluating the biomechanical properties of these devices. This chapter reviews the various thoracolumbar screw-plate systems, the biomechanical advantages of these systems, the indications for their use, and the potential complications. Muller of Germany initially developed anterior surgery for the thoracolumbar spine in the first decade of the 20th century. Capener9 in 1932 first described anterior interbody fusion, and then Ito et al10 described the technique for anterior spinal cord decompression. Lane and Moore11 reported on their techniques of anterior fusion for degenerative disc disease. Subsequently, Hodgson and Stock,12 Harmon,13 and Sachs14 described the anterior technique for management of the tubercular spine and degenerative lumbar disc disease. Humphries et al15 first developed internal stabilization techniques for the anterior lumbar spine in 1961. Since then, many devices have been developed, some of which are still being used. Although the indications for anterior instrumentation were at best controversial, in recent years their use has become more definitive and based on sound biomechanical parameters. Stabilization of a diseased spine has required the replacement of the vertebral body and/or disc with a variety of substitutes ranging from allografts to autografts to bone cement, titanium cages, ceramic spacers, or carbon fiber spacers. These vertebral body/disc replacements often require additional instrumentation to provide multidirectional spinal stability. The currently available systems can be classified into two types: rod systems and plate systems.16 Dwyer17 developed the cable systems in 1970s, which currently have only historic interest. Subsequently, the rod systems were developed for use in patients with instability secondary to trauma, tumor, and vertebrectomy or for deformity corrections. The rod systems provide excellent stability; however, the early systems were bulky and were occasionally associated with delayed vessel injury, as they were high profile.18,19 The plate systems, namely the Z-plate (Sofamor Danek, Memphis, TN), the ATLP (Synthes, Paoli, PA), and a wide variety of screw plates from other manufacturers are low-profile devices that provide excellent stability and support to the unstable spine. Spinal cord compression in metastatic disease can result from direct compression from soft tissue tumor, extruded retropulsed bone fragments, progressive kyphosis from vertebral collapse, and rarely, direct intradural invasion. Tumor invasion may in itself affect the ability of the spine to withstand load and may result in progressive kyphosis and neurologic deficit. Radical tumor decompression may further destabilize the spine. Spinal stability in the context of tumor invasion has different connotations to different authors.3,8,20,21 It is estimated that 10 to 30% of patients with metastatic spine disease have instability. Although there is ample literature on the guidelines of spinal stability in the traumatic thoracolumbar spine,22 there is little consensus on what constitutes instability in the neoplastic spine except when there is obvious translation or kyphosis. Holdsworth23 conceptualized the spine into two columns. The anterior column is composed of the disc, vertebral body, and both longitudinal ligaments, whereas the posterior column includes all structures posterior to the posterior longitudinal ligament. In the three-column model of Denis, the anterior column of Holdsworth was divided in two. The middle column consisted of the posterior half of the vertebra and the posterior longitudinal ligament (PLL). Denis suggested that this column was the primary determinant of mechanical stability. In this model, involvement of two of three columns indicated instability. Panjabi and colleagues24 supported this biomechanical concept. Kostuik et al25 based their model of spinal stability on the three-column concept of Denis but further subdivided each column into the right and left halves. According to this model if one or two of the six columns were destroyed, then the patient was probably stable. If three or four of the columns were destroyed, the spine was considered unstable. Angular deformity of more than 20 degrees was also considered a sign of instability.26 The other criteria used by them were anterior and middle column involvement or more than 50% collapse of vertebral height, middle and posterior column involvement or shearing deformity, three-column involvement, involvement of the same column in two or more adjacent vertebra, laminectomy in the setting of anterior and middle column disease, and resection of more than 50% of the vertebral body. Harms27 suggested that the spine be understood as a mobile two-column weight-sharing system in which the anterior column supports 80 to 90% of the axial load, with the remaining going through the posterior articulations. They suggested that anterior reconstruction alone may not be adequate to restore torsional stability or tensile strength when pedicles and facets are involved. James et al28 suggested that the integrity of the posterior column was crucial in resisting flexion-deforming forces in patients with a thoracolumbar burst fracture. In an experimental model of L1 burst fracture, they showed that the posterior column compromise increased the instability due to an anterior column injury, whereas the addition of a middle column injury did not affect stability. They concluded that thoracolumbar burst fractures could be managed conservatively if the posterior column were intact. The thoracic spine is structurally and biomechanically distinct from the cervical and lumbar spine. As a transitional region, it is designed for rigidity, maintaining an erect stance, and protecting the vital thoracic structures and the spinal cord. The upper thoracic vertebra resembles the cervical spine. The angulation of the facet joints in the sagittal plane is steeper. This protects against translation but allows axial rotation, which is the major motion in the thoracic spine. The orientation of the facet joints changes in the caudal thoracic spine, where it resembles the lumbar spine, which may happen between T9 and T12. The facets joints at these locations provide less stability against translation. At the same time the vertebra also become larger and resemble the lumbar spine. This is reflected in an increase in size of the thoracic pedicles at caudal levels. The thoracic spine normally has an element of kyphosis, which predisposes it to instability in flexion; however, the costovertebral joints and the rib cage afford much stability to the thoracic spine. The anatomy of the lumbar spine is designed to bear loads generated by the body and additional forces involved in standing, sitting, and bearing weights. Intradiscal pressure measurements in different body postures show that the highest pressures are observed in sitting or standing in flexion.29 The facet joints are oriented sagittally and prevent axial rotation. Range of motion studies in the lumbar spine show that flexion-extension and lateral bending are the main movements of the lumbar spine, with the L4–L5 and L5–S1 being the most mobile segments. The lumbar spine has a gentle lordosis that assists in the maintenance of posture. Internal fixation methods across the lumbar spine should maintain this lordosis. Few animal studies have been done to evaluate the ideal approach for stabilizing the spine following removal of the anterior column. Most studies have shown that rates of fusion were higher when the anterior fusion was supplemented by anterior instrumentation. All biomechanical studies using cadaveric models have potential drawbacks, as the direct extrapolation of these results from experimental studies should take into consideration variations in age, bone mineral density, soft tissue fatigue, etc.30 These authors used a synthetic vertebral model made of an ultrahigh-molecular-weight cylinder according to the dimensions of the vertebral body. Two cylinders were spanned across by a variety of plate and rod constructs. These were tested in static and fatigue modes. Although there was no substantial difference among these devices, the Synthes plate and Kaneda SR titanium performed better at higher loads. Similarly, fatigue testing at 400, 500, and 600 N showed that the Synthes plate, Olerud plate, and Kaneda SR titanium plate withstood 2 million cycles without failure, a significant difference over the remaining systems tested (p <.05). Failures were noted at the upper bolt screw interface in the Universal plate system, and plate fractures and bolt/plate dislodgment occurred in the Z-plate system. Several other studies have compared the biomechanical properties of anterior spinal instrumentation systems.31–33 Zdeblick et al33 conducted nondestructive mechanical testing of four anterior systems in a calf corpectomy model. In both plate and rod devices tested, the Kaneda and TSRH systems were most effective in restoring overall stability. An et al34 studied four anterior fixators, namely the Universal anterior plating system, the Kaneda device, the Z-plate, and the TSRH system, using fresh calf spines. With an interbody graft in place, all devices showed adequate stabilizing effects. The stabilizing effects of the devices in flexion, extension, and lateral bending were similar to each other except that the Z-plate provided significantly less stability in extension than the Kaneda device (p <.05). The torsional or axial rotational stability was restored with all constructs; however, the Kaneda device exceeded the torsional stability beyond that offered by the intact spine. To assess the role of the interbody graft, the movements of the construct were tested in all directions after removing the graft. Once again the Kaneda device reduced motion in all directions, whereas the anterior plates were less restrictive. Lim et al32 evaluated the University plate, Kaneda device, and posterior Isola system. Dick et al31 used a synthetic polyurethane saw bone model and performed static and fatigue testing on four devices. They showed the superiority of the Synthes anterior thoracolumbar locking plate system. In vitro biomechanical studies on human cadaveric specimens following one-level vertebrectomy were conducted by Hitchon et al.35 Two anterior plating systems, the ATLP and the Z-plate, were compared with the SRK system. There was no difference between the Kaneda and the Z-plate in any state. Ashman et al36 tested four anterior fixation systems: the Zielke-Slot, the Kostuik-Harrington device, ASIF T-plate, and a broad compression plate. They concluded that the compression plate was stiffer in axial load and torsion than the other systems. Anterior decompression and plate reconstruction may be augmented by a posterior instrumentation, as recommended by Vahldiek and Panjabi.37 They studied fresh human cadaveric spine and showed that posterior and combined instrumentation provided additional stability over that of the stand-alone anterior instrumentation. The combined construct provided greater torsional and flexural rigidity. Although biomechanical evaluation of anterior plating systems provides useful information for the clinician, one has to consider additional clinical factors in making a decision as to whether posterior reconstruction needs to supplement an anterior fusion construct. Some patients may need to be supplemented with a posterior construct to provide a posterior tension band to increase stability, particularly if the patients’ bone quality is poor (osteoporosis) or the patients have under-gone previous radiation or will require radiation soon after surgery. In some slow-growing primary tumors, which cannot be radically extirpated, it is sometimes useful to fuse multiple levels posteriorly initially so that if subsequent decompressive surgery is anticipated in the future because of local recurrence, it can be done in the setting of a fused spine. Anterior approaches to the thoracolumbar spine allow direct access to the tumor involving the ventral aspect of the spine without sacrificing the bony and ligamentous structures of the posterior column of the spine. This allows radical decompression and stabilization/reconstruction at the same time. Appropriate patient selection is mandatory in the treatment of primary malignant and metastatic tumors of the spinal column. Complication rates can be diminished by avoiding surgery in patients who are old or have multiple medical problems, poor cardiorespiratory reserve, a compromised immune status, or heavy metastatic disease burden. The ideal patient to consider for a ventral approach and reconstruction has localized anterior spinal disease and has a well-controlled primary malignancy. The preoperative workup should include a detailed metastatic workup, pulmonary function tests, and cardiac evaluation. The upper thoracic spine can be accessed via a straight anterior approach by splitting the manubrium and occasionally the sternum as well. In the rare patient with a long slender neck, a complete T1 corpectomy and reconstruction can be performed without splitting the anterior chest wall. The dissection is similar to that of an anterior cervical approach with a plane achieved between the esophagus and trachea medially and the carotid and internal jugular vein laterally. The longus colli muscles are retracted laterally to access the midportion of the body. The inferior limit of exposure relates to the retraction of the great vessels and aortic arch inferiorly. One can generally access T4 without difficulty. Reconstruction after a corpectomy can be accomplished with the use of anterior cervical plating systems (Fig. 36-1). Specific structures that must be avoided in this area include the thoracic duct on the left and the recurrent laryngeal nerve, which is also prone to injury. A left-sided approach is generally preferred at the level of the thoracolumbar junction, as the liver can limit exposure with a right-sided approach at this level. In addition, the inferior vena cava is thin walled and prone to injury with retraction. Injuries to the vena cava can result in catastrophic bleeding, which is usually more difficult to control when compared with bleeding from the aorta. The right-sided approach can be used if the tumor is entirely restricted to that side. In addition, tumors that involve the upper to midthoracic spine (T4 to T7) are frequently more easily approached from the right because at these more rostral thoracic levels the aorta is more lateral in location and can cover the working surface of the lateral thoracic body. The patient is positioned in a strict lateral decubitus position on a beanbag and brought to the edge of the table. An axillary roll is placed in the axilla to avoid pressure on the brachial plexus. Careful incisional planning is crucial and is dependent on the precise level of pathology as well as the rib slope, which varies between individuals, and the level of the spine that is to be operated on. The rib slope is more pronounced at lower thoracic levels, and thus the incision tends to be about two levels above the spinal pathology, whereas at more rostral thoracic levels the rib incision is only one level above. For example, a T12 corpectomy entails making an incision over the 10th rib, whereas a T8 corpectomy entails making an incision over the 7th rib. The incision usually extends from the anterior axillary line posteriorly toward the erector spinae. The appropriate rib is resected subperiosteally and the pleura opened along the bed of the rib. Once the pleural cavity is entered, the ribs are counted from above and the appropriate level confirmed. Intraoperative plain x-rays should be performed to confirm the diseased vertebra. A careful comparison of these films with preoperative films of the thoracic and lumbar spine can be very helpful in confirming the appropriate level of surgery. Exposure of the L1–L4 vertebrae can be achieved through a flank incision depending on the level of the exposure necessary. The external oblique muscle is split in line with the incision to the lateral border of the rectus muscle. The internal oblique and the transversus cut across their fibers to reach the retroperitoneal space. The peritoneum is reflected from the transversalis fascia, and all peritoneal tears are closed primarily. The kidney is reflected along with the Gerota’s fascia, and the spine is exposed. At this stage one must be very careful to stay anterior to the psoas and the quadratus lumborum muscles. The lumbar plexus is located posterior to these muscles and may be damaged if the psoas is transgressed. The sympathetic chain can be identified at this stage as a yellow band traversing the lateral aspect of the vertebral body. It should be gently separated from the vertebra. The segmental vessels need to be divided at this stage. The segmental arteries should be ligated closer to the origin of the aorta than directly within the intervertebral foramina to avoid disturbing any potential segmental medullary branches. The veins should also be coagulated and divided between ligatures or clips. Difficult bleeding may be encountered if the veins tear from the inferior vena cava (IVC) or the iliac vessels.
Corpectomy and Anterior Lateral Thoracic and Lumbar Screw Plate Fixation in the Management of Spinal Tumors
History of Anterior Thoracolumbar Fusion Surgery
Evaluation of Instability in Spinal Tumors
Anatomy of the Thoracic and Lumbar Spine
Biomechanical Evaluation of Plating Systems
Operative Technique
Surgical Approaches
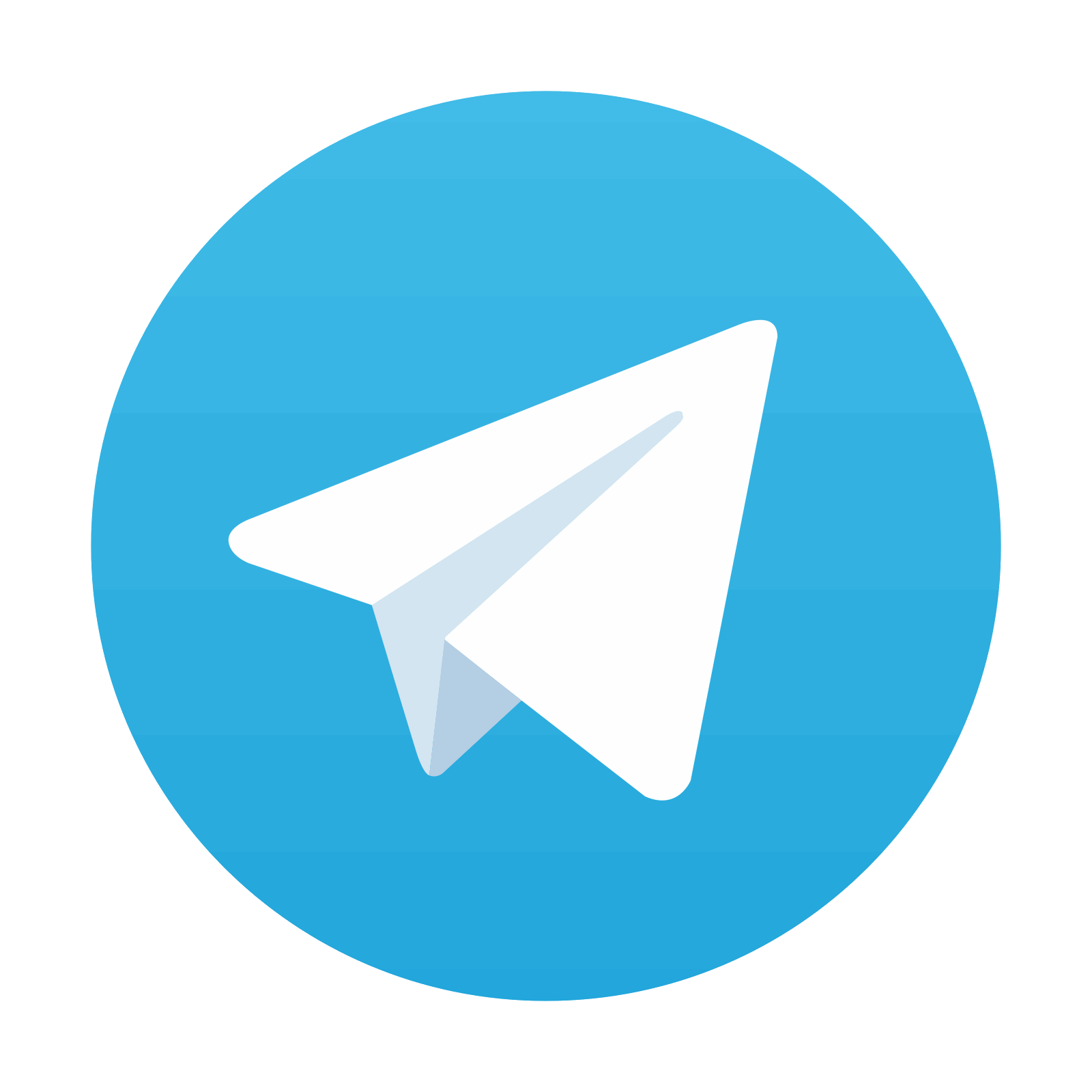
Stay updated, free articles. Join our Telegram channel

Full access? Get Clinical Tree
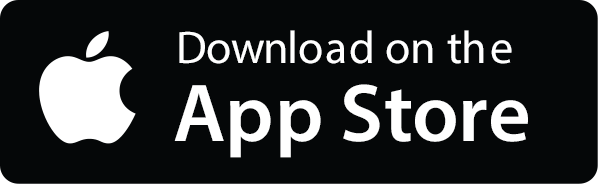
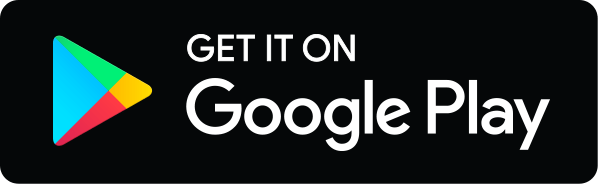