Cranial Vascular Malformations
Etiology
The prevalence of cranial vascular malformations is between 15 and 18 per 100,000 adults.1 The detection rate ranges between 1.12 and 1.34 per 100,000 person years.2,3 Autopsy data suggest that there is an overall frequency of detection of arteriovenous malformations (AVMs) in approximately 4.3% of the population.4,5 Autopsy data suggest that as few as 12% of arteriovenous malformations are symptomatic during life.6 Cerebral arteriovenous malformations are thought to be congenital vascular lesions consisting of abnormal direct connections between the arterial and venous systems (Fig. 14.1).7,8 The exact embryological origin is still unknown, but both persistence of a primitive arteriovenous connection and development of such a connection before or after birth have been suggested.2
Genetic Factors
AVMs are traditionally thought to be sporadic, congenital vascular lesions. It has been suggested that cerebral AVMs originate at or before the 40 mm to 80 mm embryo length stage and may be related to a primary abnormality of primordial capillary or venous formation.7 Familial occurrences have been reported.8 However, genetic factors for brain AVMs are still largely unexplored because of the low incidence of familial cases, but genetic data for these congenital malformations are being pursued. Cerebral AVMs are diagnosed much more in adults than in children.8,9 Some AVMs, however, are associated with congenital or hereditary syndromes (Table 14.1).10
Fig. 14.1 Angioarchitectural features of AVMs.
Environmental Factors
Triggering environmental factors for AVM hemorrhage have yet to be well defined. The trigger could be mechanical, hormonal, thrombotic, hemodynamic, thermal, ischemic/hypoxemic, or inflammatory in nature.11,12
Diagnosis
Presentation
Intracranial AVMs are occasionally seen in the elderly but are typically diagnosed before the patient has reached the age of 40 years. The most common presentations of AVMs are hemorrhage (>50%) and seizure (20%–25%); less common are headaches (15%), focal neurological deficits from steal phenomenon (5%), and tinnitus.13–16
AVM rupture most commonly causes intracerebral hemorrhage followed by subarachnoid and intraventricular hemorrhages, although isolated cases of subdural hematomas have been reported. Severe vasospasm from AVM-related hemorrhage is uncommon.14 Seizures can be either focal or generalized and may be an indicator of the location of the lesion. In children younger than two years of age, presentation can include congestive heart failure, a large head due to hydrocephalus, and seizures.
Occasionally, an AVM-related steal phenomenon can cause focal neurological deficits by altering perfusion in the tissue near the AVM.15,17 The steal phenomenon can result from cerebral artery hypotension, leading to ischemia in brain tissue adjacent to the lesion.18,19 Venous hypertension and any mass effect of the malformation are alternative explanations for the progression of focal neurological deficits.20
Table 14.1 AVM-Associated Syndromes
Rendu-Osler-Weber |
Klippel-Trenaunay |
Parks-Weber |
Wyburn-Mason |
Sturge-Weber |
Clinical Examination
Thorough physical and neurological examinations are needed. Specific findings are related to the location of the lesion in question. When the lesion is associated with other congenital or systemic conditions, other physical exam findings can be found.
Diagnostic Imaging Studies
CT and CT angiography are the first and best imaging studies in hemorrhagic AVM cases, especially in an emergent setting. MRI may not be the first choice for imaging, due to the time required for acquisition of images. However, MRI can provide superior visualization of surrounding brain structures. Further, MR fluid attenuation inversion recovery (FLAIR) imaging can be helpful for detecting small AVM hemorrhages, focal parenchymal changes and mass effect. On MRI, AVMs are detected by inhomogeneous signal voids on T1- and T2-weighted sequences. Gradient-echo sequences can detect hemosiderin, which suggests prior hemorrhage. MR angiography provides information on the vascular anatomy of the AVM but frequently has a lower spatial resolution than CT angiography or cerebral angiography. However, time-resolved MR angiography continues to improve and has been used for general follow-up after treatment as well as radiosurgery treatment planning. A functional MRI can be used to determine the eloquence of the surrounding brain parenchyma, potentially altering management strategies.
Cerebral angiography is an invasive study but remains the gold standard for defining the angioarchitecture of AVMs. Most institutions advocate cerebral angiography at some point in the management phase of AVMs. Cerebral angiography not only can provide highly detailed information on anatomic features in all phases, including points of fistulous connections, associated aneurysms, nidal size, and arterial and venous flow patterns necessary for utilizing the Spetzler-Martin grading scale, but also can provide important hemodynamic information regarding dominant arterial filling and pedicle arrangements. A meta-analysis demonstrated that the risk of conducting diagnostic angiography is significantly lower in patients with AVMs (0.3–0.8%) than in those evaluated for transient ischemic attack or stroke (3.0–3.7%).21
Spetzler-Martin Grading Scale
The Spetzler-Martin grading scale predicts the neurological outcome in case of surgical resection, and it has become the scale most often used by treating physicians to describe anatomic details for a specific AVM22 (Table 14.2). In the endovascular era, the Spetzler-Martin scale has generated much debate regarding its utility in assessing endovascular outcomes. Thus, alternative grading scales have been postulated but not frequently accepted. Therefore, the Spetzler-Martin grading scale has remained the dominant classification scale. In this classification scale, AVMs are defined by three variables: size, location, and the pattern of venous drainage.
• Sizes are graded as small (0 to 3 cm, 1 point), medium (3 to 6 cm, 2 points), or large (greater than 6 cm, 3 points).
• AVM location is divided into eloquent (1 point) and non-eloquent (0) regions. Eloquent areas include sensorimotor, language, and visual areas, as well as the thalamus, hypothalamus, internal capsule, brainstem, cerebellar peduncles, and deep cerebellar nuclei.
• The pattern of venous drainage can affect surgical accessibility and is divided into superficial (0) and deep (1 point). Only cortical venous drainage and cerebellar venous drainage into the straight or transverse sinus are considered to be superficial. However, the importance of drainage patterns has been challenged in recent years, as superficial venous drainage can often obstruct surgical resection whereas deep drainage does not obstruct resection.
Table 14.2 Spetzler-Martin Grading Scale
Graded feature | Points assigned |
Size |
|
Small (<3 cm) | 1 |
Medium (3–6 cm) | 2 |
Large (>6 cm) | 3 |
Eloquence of Adjacent Brain |
|
Non-eloquent | 0 |
Eloquent | 1 |
Pattern of Venous Drainage |
|
Superficial | 0 |
Deep | 1 |
The scale does not include characteristics like associated aneurysms, venous stasis or stenosis, or venous aneurysms or varicies that have been associated with increased hemorrhagic risk. It has been established that several morphologic factors, such as small AVMs, impaired venous drainage, deep draining vein, single draining vein, feeding artery or nidal aneurysm, venous varix, and high intranidal pressure, are all associated with AVM hemorrhage, but their absolute relevance remains unclear. Clinical outcomes after surgical resection of intracranial AVMs can be predicted with this scale.23 The predicted lifetime risk of hemorrhage is:
1 − (1 − annual hemorrhage risk)n
where n = the remaining years of life expected. This risk should be weighed against the risk of surgery as predicted by the Spetzler-Martin grading scale (Table 14.3), the experience of the neurosurgeon, and prior clinical status after presentation.
Evidence-Based Medicine
The main goal of AVM treatment is to eliminate the risk of hemorrhage and all related symptoms, such as seizures and neurologic deficits, without any or with only minimal morbidity or mortality to the patient. The available methods of treatment are microsurgery, radiosurgery, endovascular treatment with embolization, or a combination of these techniques.
Several factors are considered in the decision to treat an AVM, such as symptoms, age of the patient, medical condition, the size and location of the AVM, the type of venous drainage, the history of hemorrhage, and the natural history of AVMs in general.
Existing data indicate that only complete eradication of the lesion provides the most complete protection from future hemorrhage; partial treatment may not be helpful and may, in fact, increase the rate of future hemorrhage.
A current attempt to unravel the mysteries of unruptured AVMs has been undertaken by several prospective databases across the world. In the United States, the ARUBA trial encompasses a prospective database involving multiple academic centers where AVMs are randomized to either observation or treatment when the treating physician feels the AVM does not pose any immediate risk. While this trial is still undergoing enrollment, it will hopefully shed some light regarding what the natural history of AVMs dictates as true hemorrhagic risk. Although promising, this trial has met with much criticism.
Bleeding and Rebleeding Rates
• The AVM hemorrhage rate has been classically reported to be approximately 2%–4% per year,24–30 but the actual rupture risk may differ between distinct patient subgroups.
• The first episode of bleeding is associated with a mean mortality rate of 10%, which increases to 15% with the second hemorrhage and to 20% with the third one. An additional 20% mean rate of neurological morbidity accompanies each hemorrhage.31 After a hemorrhagic episode, the rate of rehemorrhage increases to 6% in the first year and then returns to 2%–4% per year thereafter.31 Grade IV and V AVMs appear to have a lower rate of hemorrhage, which is estimated at 1.5% per year.31
• Data from the New York Islands AVM Hemorrhage Study suggest that the AVM detection rate is 1.21/100,000 person-years, and the incidence of AVM hemorrhage is 0.42/100,000 person-years.32
• Data from the prospective, longitudinal, population-based Northern Manhattan Stroke Study suggest that the crude incidence for first-ever AVM-related hemorrhage is 0.55/100,000 person-years.33
Surgery
• The Spetzler-Martin grading scale has also been applied prospectively. Grade I, II, or III AVMs were found to have low treatment-associated morbidity.34 However, grade IV lesions conferred 31.2% treatment-associated morbidity, and grade V lesions had 50% new treatment-associated morbidity. In addition, the rate of permanent deficit was 29.9% for grade IV lesions and 16.7% for grade V lesions.34 The authors recommend surgery for all grade I and II lesions. Grade III lesions should be treated on a case-by-case basis. Grade IV and V lesions require a multidisciplinary approach with individual analysis.34 However, these figures were developed at a time when there were no other adjunctive therapies, such as endovascular embolization. Current multimodality therapy may alter these results, but the data have yet to be determined.
• In one study, 67 patients underwent resection of AVMs less than 3 cm in their largest diameter, regardless of location. Complete angiographic obliteration of the AVM by microsurgical technique was accomplished in 63 patients (94%) with a surgical morbidity of 1.5% and no operative mortality. Patients with hemispheric AVMs had a cure rate of 100% and no neurological morbidity.35
• Accumulating data suggest that partial AVM resection does not reduce, but rather increases, the risk of future hemorrhage.36 Partially treated grade IV and V AVMs carry a 10.4% risk of hemorrhage, compared with a 1% risk in patients with no previous treatment.31
Embolization
• The rate of complete obliteration of brain AVMs by using embolization as the sole method of treatment is thought to be between 5% and 40%.37 These AVMs are usually smaller than 3 cm in diameter and have only one or two feeding arteries. The chance of complete obliteration was inversely proportional to AVM volume and the number of feeding pedicles. To achieve permanent obliteration, a nonbiodegradable agent should be used to occlude the AVM nidus. Complete obliteration of the brain AVM should be demonstrated by the immediate postprocedure angiogram and confirmed by follow-up angiography.37
• Valavanis and Yasargil reported complete AVM obliteration with embolization alone in 39% of 387 patients. In the subgroup analysis of 182 AVMs that were treated with intent-to-cure, they reported a cure rate of 75%.38 Yu et al. reported achieving complete obliteration rates of 60% in patients treated with embolization alone.39 An initially high degree of obliteration of the AVM nidus may be followed by its recurrence shortly after embolization. The extent to which the collateral supply develops and the time required for its development may depend on the degree of nidus obliteration and the material used for the obliteration.40
• Although the reported rates of complete endovascular obliteration vary, most estimates are in the range of 10%. However, it is important to note that these estimates are based on series that predate the introduction and widespread application of the liquid embolic Onyx (MicroTherapeutics, Inc., Irvine, CA) and, therefore, may be expected to increase with Onyx.36
Radiosurgery
Radiotherapy is recommended for small (<3 cm in diameter), deep malformations for which a surgical approach involves passing through functionally important brain tissue, for malformations in an eloquent area, and for ones in which the large number of feeding arteries or the presence of endovascularily inaccessible feeders makes embolization impractical. In general, the success of radiotherapy is inversely proportional to the size of the AVM nidus to be treated.41 AVMs with nidal volumes less than 10 mL (diameter <3 cm) are frequently curable by radio-surgery, with rates of obliteration at 2 years estimated at between 80% and 88%.39,42 However, it takes two to three years before the efficacy of the treatment can be determined, and the hemorrhage risk either remains or can increase up to 11–16% during the first six months after treatment. Furthermore, secondary complications from the effects of radiation on the surrounding parenchyma or cranial nerves may confer unacceptable morbidity.
Combination Therapy
The goal of combination therapy is to maximize the benefits of each option and decrease the overall morbidity and mortality of AVM treatment. Embolization of AVMs can be beneficial prior to microsurgical resection or radiosurgery (Table 14.4) to reduce the treatment volume or potential blood loss during resection.45,46,47
Gobin et al. reported their experience with 125 patients undergoing embolization (predominantly with n-BCA) prior to radiosurgery. They achieved total occlusion in 11.2% of AVMs after embolization alone, with an additional 76% of lesions reduced sufficiently in size to undergo radiotherapy. A 65% rate of total occlusion was observed after radiotherapy in patients undergoing combined treatment.43 Despite the straightforward rationale for pre-radiosurgical embolization, very little data currently exist to support this approach. From the existing data, no compelling evidence exists to justify or refute the usefulness of pre-radiosurgical embolization.36
Preprocedural Preparation for AVMs
Unruptured AVMs
• Similar to other endovascular interventions.
• Check blood level of antiseizure medications if the patient takes them.
• Review all CT, MRI, and angiography imagery thoroughly prior to the procedure.
• Often done on an elective basis.
Table 14.4 Benefits of AVM Embolization before Microsurgery and Radiosurgery
Microsurgery | Radiosurgery |
Occludes the deep and surgically inaccessible feeding pedicles. | Decreases the size of large AVM (>3 cm) so radiosurgery can be performed. |
Decreases blood flow and nidal size, resulting in less blood loss during the surgery. | Treat AVM-associated aneurysms prior to radiosurgery (radiosurgery may not be effective for the aneurysms). |
Onyx or n-BCA in the feeding arteries or nidus provides a good road map during resection. | Smaller residual AVMs have higher radio-surgical cure rate.69,80,81 |
Treat AVM-associated aneurysms prior to the surgical resection. |
|
Ruptured AVMs
• Same for unruptured AVMs.
• Head CT or MRI to assess intracranial hemorrhage, hydrocephalus, or other acute intracranial lesions.
• Often done on a more emergent basis. Surgical evacuation of a significant hematoma may precede the actual treatment of the AVM by several weeks.
AVM-Associated Aneurysms
• Same for unruptured AVMs.
• In case of ruptured AVM aneurysms, treatment of the aneurysm should be done before or during definitive treatment of AVM. Although rare, if the aneurysm rupture confers a significant amount of hemorrhage into the subarachnoid space, vasospasm should be anticipated, which may change the timing or strategy of definitive AVM therapy.
• Despite its inherent difficulty, determination of the source of hemorrhage should be considered, that is, whether the AVM or aneurysm is the source.
Equipment
Sheath
Selection of a sheath is often based on preference and the experience of the operators. A 4 Fr or 5 Fr sheath 11 cm long is most commonly used for diagnostic angiography. A 6 Fr or 7 Fr sheath 11 cm long or longer (up to 35 cm) for tortuous proximal access or a 6 Fr Shuttle Select Tuohy-Borst introducer (Cook, Inc.) (90 cm in length) can be used for embolization.
Catheters
Angled glidecaths are useful for uncomplicated or Type I arches. The Simmons II catheter is useful for tortuous great vessels or a complicated aortic arch. For more stability, co-axial guiding systems like the 6 Fr Envoy (MPC or Sim 2) (Cordis Neurovascular, Inc., Miami Lakes, FL), 6 Fr Neuron (ID 0.070 or 0.051) (Penumbra, Inc., Alameda, CA) catheter, or 6 Fr Chaperon MP2 (Microvention, Inc., Aliso Viejo, CA) guide catheters can be used through a 6 Fr or 7 Fr shuttle sheath. Two techniques to place guiding catheters or long or shuttle sheaths in the common and internal carotids and vertebral arteries of difficult aortic arches are described in Table 14.5. In addition, distal access catheters can be used in conjunction with standard guide catheters to stabilize the catheter system further and allow for more stable distal access.
Glide Wires
Most commonly, a 0.035 or 0.038 guide wire is used in 150 cm or 260 cm lengths. Stiff wires are normally used for tortuous access. Exchange length 0.035 or 0.038 glide wires can be used as well, especially in anticipation of exchanging a diagnostic catheter for a guide catheter. The Amplatz J-wire can be useful, especially in cases where the external carotid artery is used as an anchor while delivering a guiding catheter or shuttle sheath.
Microcatheter
As mentioned above, selection of the microcatheter is most frequently based on the preference and experience of the operator, as well as the microcatheter’s trackability or support. Tips are shapeable with hot steam. Several pre-shaped microcatheters are available, among them 45 degree, 90 degree, J, C, or S-shaped tips. Microcatheters are divided into flow-guided and microwire-directed types (Table 14.6).
Microwire
Selection is based on preference and experience. Larger and stiffer wires have more stability and trackability, but they also have more risk of perforation of the vessels (Table 14.7).
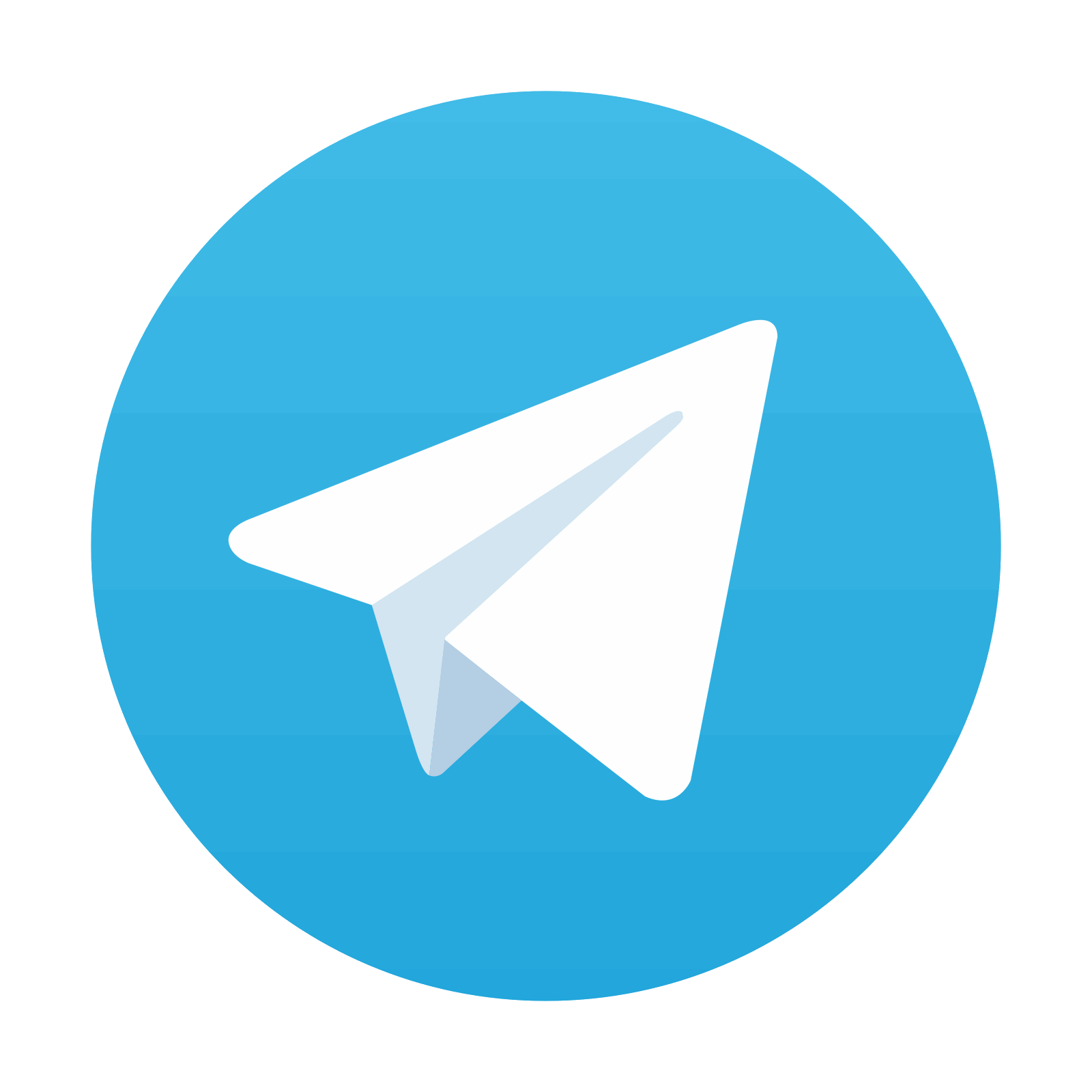
Stay updated, free articles. Join our Telegram channel

Full access? Get Clinical Tree
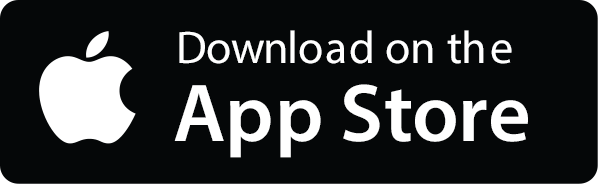
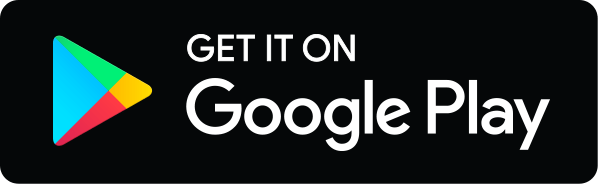