(1)
Department of Motor Neuroscience and Neurotherapeutics, Institute of Health Bioscience, Graduate School of Medical Science, University of Tokushima, Tokushima City, Tokushima Prefecture, Japan
11.1 Introduction
Essential tremor (ET) is the most common movement disorder in adults (Deuschl et al. 2011). The postural and action–intention components affecting distal and proximal musculature are the typical manifestations of advanced ET. ET affects the upper extremities, head, voice, legs, and trunk. The primary treatment for ET is pharmacotherapy. Two drugs, primidone and propranolol, have been established as effective (level-A evidence by the criteria of the American Academy of Neurology) (Zesiewicz et al. 2011). However, 30–50 % of patients will not respond to both drugs (Koller and Vetere-Overfield 1989). Five drugs, topiramate, alprazolam, atenolol, gabapentin, and sotalol, have been shown to be compatible to level-B evidence (probably effective). Irrespective of these medications, 25–55 % of patients manifest medication-refractory ET (Louis 2001). Around 10 % of patients experience significant physical impairment and markedly decreased quality of life. Although the evidence for surgical therapies remains at level C (possibly effective) due to a lack of class-I and class-II studies, thalamotomies and deep brain stimulations have been applied to drug-resistant ET patients, and they have been reported to be highly efficacious for upper limb tremor in patients with ET (Deuschl et al. 2011).
Although the therapeutic efficacy of ablation surgery may be comparable to that of DBS (Schuurman et al. 2000, 2008), DBS is currently preferred over thalamotomy due to its reversibility and adjustability and to the undesirable and irreversible tissue damage that is caused by the ablation surgery (Tasker 1998). The ventral intermediate (VIM) nucleus of the thalamus has been used as a classic target for ET since 1987 (Miocinovic et al. 2013). However, axial tremor, the action component of distal tremor, and proximal tremor respond poorly to VIM DBS (Benabid et al. 1991, 1996). In addition, VIM DBS manifests a high incidence of dysarthria and disequilibrium (Benabid et al. 1991, 1996; Pahwa et al. 1999; Taha et al. 1999) and tolerance to the stimulation (i.e., habituation) (Benabid et al. 1991, 1996; Hariz et al. 1999). The posterior subthalamic area (PSA), which is composed of the prelemniscal radiation (Raprl) and the caudal zona incerta (cZI), has been a target of early ablation surgery in patients with tremor (Ito 1975; Krauss et al. 1994; Mundinger 1969; Velasco et al. 1972). Recently, this structure has been revived as another option for DBS for ET (Lehman and Augustine 2013). Subthalamic nucleus (STN) DBS has also been considered for ET (Lind et al. 2008).
11.2 Pathophysiology of Essential Tremor
The precise pathogenesis of ET is poorly understood. In the harmaline animal model, abnormally synchronized (4–10 Hz) oscillations have been identified in the inferior olivary nucleus (ION), and these are transmitted to the dentate and interpositus nuclei in the cerebellum (Park et al. 2010; Wilms et al. 1999). The calcium-dependent neuronal synchrony in the ION is sufficient to drive the cerebellothalamocortical circuit to produce tremor (Park et al. 2010), and a functional imaging study has shown the abnormal activation of the ION in ET (Boecker et al. 1996). These results suggest the dysfunction of the olivocerebellar circuit is the primary cause of ET (Deuschl and Bergman 2002). There are no morphological changes in the ION, supporting the idea that ET is due to a functional abnormality within the central nervous system (Deuschl et al. 2001; Louis et al. 2013). The ION adjusts or modulates planned movements during their execution in response to unconditioned afferent information; in other words, it is a movement error detector (Plaha et al. 2004).
The cerebellar nuclei connect to the ventrolateral thalamus through the cerebellothalamic tract and consecutively to the motor cortex. Plaha et al. (2008) have presumed that excessive movement correction in response to limb displacement detection and overcorrection might create abnormal oscillation in the ION in ET. Louis and Vonsattel (2008) have proposed that the cerebellum is integrally involved in the pathophysiology of ET, and some neurodegenerative features in the cerebellum have been shown (Louis et al. 2009). This hypothesis is supported by the findings of a recent neuroimaging study that ET is associated with γ-aminobutyric acid (GABA)ergic neurotransmission dysfunction in the cerebellum (Boecker et al. 2010; Gironell et al. 2012).
Although the olivocerebellar system and the thalamus are key structures, corticomuscular coherence studies have suggested the existence of several central pacemakers in the cerebellum, thalamus, and motor cortex (Govindan et al. 2006; Hua and Lenz 2005; Raethjen and Deuschl 2012; Schelter et al. 2009; Schnitzler et al. 2009). Recent studies using magnetoencephalography (MEG) have revealed that all of the centers simultaneously work and are not fixed and that its constituents may vary over time (Raethjen et al. 2007; Schnitzler et al. 2009; Raethjen and Deuschl 2012). The intermittent cortical involvement may shift the mode of cooperation between all of the constituents of the tremor network (Raethjen et al. 2007; Raethjen and Deuschl 2012). The so-called burst and tonic modes of activity have been observed in thalamic neurons. In the burst mode, the transfer of information has a high signal-to-noise ratio that is conveyed in a nonlinear manner such that new signals are readily identifiable but not readily analyzed. In the tonic mode, there is a linear transfer of the activity of specific afferents, but the signal-to-noise ratio is low (Godwin et al. 1996). These patterns of activity have been seen in the lateral thalamus (Tsoukatos et al. 1997; Zirh et al. 1998). The interaction within the network seems to determine the emerging type of movement (Raethjen and Deuschl 2012), including ET.
The contribution of the basal ganglia loop in generating ET has been controversial (Gerasimou et al. 2012; Lou and Jankovic 1991). The fact that the stimulation of VIM, PSA, and STN could also affect neurons and fibers that are included in the basal ganglia loop has suggested that stimulation of both the cerebellothalamic and pallidothalamic fibers might be responsible for the therapeutic effects on tremor (Deuschl and Bergman 2002).
11.3 Anatomy and Electrophysiology of DBS Surgery
The VIM nucleus, which receives strong cerebellothalamic afferents, is a major target of tremor in functional neurosurgery (Asanuma et al. 1983a, b; Ilinsky and Kultas-Ilinsky 2002; Kultas-Ilinsky and Ilinsky 1991). Vim DBS has demonstrated promising results for the treatment of ET (Rehncrona et al. 2003; Sydow et al. 2003). Recently, many studies have demonstrated that the PSA (Fytagoridis et al. 2012; Kitagawa et al. 2000; Murata et al. 2003; Plaha et al. 2004, 2008, 2011; Velasco et al. 2001) and STN (Blomstedt et al. 2011a, b; Lind et al. 2008; Stover et al. 2005) could be alternative targets. Recent evidence has revealed that the effects of DBS for ET in these subthalamic structures yielded good results.
11.3.1 The VIM Nucleus
11.3.1.1 Functional Anatomy of VIM
The ventrolateral (VL) thalamus is comprised of ventral oral posterior (Vop) nucleus, ventralis oralis internus (Voi), and the VIM nucleus (Hassler 1959). Anterior and lateral to these nuclei lie the reticular nucleus of the thalamus and the internal capsule (El-Tahawy et al. 2004). The VL thalamus mediates motor control. It receives afferent fibers from the cerebellum, basal ganglia, cerebral cortices, and spinal cord, and it projects efferent fibers to the cerebral cortices and basal ganglia. The VL has two subcortical afferent territories: the pallidothalamic and cerebellothalamic territories (Ilinsky and Kultas-Ilinsky 1987; Kuo and Carpenter 1973; Nakano 2000; Sidibé et al. 1997). The density of the pallidothalamic territory decreases in an anterior to posterior gradient and that of the cerebellothalamic territory decreases in a posterior to anterior gradient in the VL thalamus (Sakai et al. 1996). These two territories are widely interdigitated (Asanuma et al. 1983a, b; Sakai et al. 1996). The Vop preferentially receives inhibitory pallidothalamic inputs from the globus pallidus internus (GPi), and the VIM predominantly receives excitatory inputs from the cerebellum. The efferents from the VIM nucleus preferentially project to the primary motor, premotor, and proper supplementary motor area, which, in turn, provide reciprocal excitatory corticothalamic inputs (Bromberg et al. 1981; Hoover and Strick 1999). The VIM nucleus projects predominantly to the deep cortical areas of the motor cortex (Area 4 and possibly, Area 3a), which respond to the passive motor movements of joints (Anderson and Turner 1991; Butler et al. 1992; Jones 2007; Miyagishima et al. 2007; Vitek et al. 1994). The VIM neurons are somatotopographically organized; the face, forelimb, and hindlimb receptive fields are arranged medially to laterally (Strick 1976; Vitek et al. 1994; Kurata 2005). The Vc nucleus, which is the major termination structure of the medial lemniscus, projects to the primary somatosensory cortex (El-Tahawy et al. 2004). The subthalamic area (STA) is located ventrally to the VL thalamus.
11.3.1.2 Electrophysiological Findings During VIM DBS
As the microelectrode descends toward the thalamic target, the caudate has a very slow rate of spontaneous discharge (0–10 Hz) (Vitek et al. 1998), and the thalamus is relatively quiet in the awake patient but shows occasional slow bursting activity (Starr et al. 1998). Microelectrode entry into the motor thalamus can be indicated by the identification of movement-responsive cells and/or cells discharging at tremor frequency (Lenz et al. 1994). Microelectrode recording can identify the Vc nucleus, which is a tactile relay nucleus, and its anterior border with VIM as well as the ventral border with STA (El-Tahawy et al. 2004). Neurons that respond to voluntary movements (voluntary cells) are predominantly present in the Voa and Vop (El-Tahawy et al. 2004). Voluntary cells do not respond to verbal commands but rather to the voluntary act itself, and they are sometimes difficult to distinguish from kinesthetic cells (El-Tahawy et al. 2004; Raeva et al. 1999). Kinesthetic cells receive afferents from muscle spindles that are located in the tendons and muscle bellies or from stretch receptors that are located in the tendons, joint capsules or deep tissues, and they respond to passive joint movements and proprioceptive afferents. These cells are located just anterior to the tactile receptive field (Ohye and Narabayashi 1979; Ohye et al. 1989). Tactile cells in the Vc nucleus respond to superficial light touch. Stimulation of the Vc nucleus induces paresthesia due to activation of the medial lemniscal axons (El-Tahawy et al. 2004).
11.3.1.3 Surgical Anatomy of VIM for Essential Tremor
Vim DBS reduces abnormal tremor-electromyography coherence in ET postural tremor (Vaillancourt et al. 2003). The ideal target for abolishing the tremor is supposed to be located in the area where kinesthetic and tremor cells coexist (Lenz et al. 1994; Atkinson et al. 2002). The kinesthetic zone is located in the lower and lateral part of the VIM nucleus, which is a region of confluence of cerebellothalamic and spinothalamic tracts and which sends the majority of its axons to the motor cortex (Percheron et al. 1996). Ohye and colleagues have postulated that this area is optimal for radiofrequency lesioning to abolish tremor (Ohye and Narabayashi 1979; Ohye et al. 1989). Kiss et al. (2003) have reported that there is an expansion of the representation of movement-related (kinesthetic and deep-responding) neurons anteriorly in patients with tremor. Although DBS of the lateral portion of the VIM nucleus, where tremor cells might play a predominant role, provides the best control of parkinsonian tremor, as suggested previously (Atkinson et al. 2002; Hariz and Hirabayashi 1997), this is not the case for ET and post-stroke tremor, in which, presumably, the tremor cells are spread out in wide areas (Katayama et al. 2005) and they involve more proximal muscle components, which are represented more anteriorly and dorsally in the VIM nucleus (Ohye et al. 1989). A clinical study that examined the relationship between lead location and clinical outcome of 57 leads in 37 ET patients revealed that the lead locations in the anterior margin of the VIM nucleus corresponded to significant improvements in tremor scores (Papavassiliou et al. 2004). In such cases, thalamic DBS with bipolar stimulation or a low angle of the DBS electrode to the anterior commissure–posterior commissure (AC–PC) line (~45°) is advocated, as this could cover the more anterior (Katayama et al. 2005; Kobayashi et al. 2010; Yamamoto et al. 2004; Kiss et al. 2003) and dorsal areas (Kiss et al. 2003; Nguyen and Degos 1993).
11.3.2 Posterior Subthalamic Area (PSA)
11.3.2.1 Functional Anatomy of the PSA
The PSA and its vicinity comprises the nuclei area, which includes the Zi, the STN, and substance Q of Sano, and the fiber area, which includes the ansa lenticularis, Forel’s fields H, H1, and H2, Raprl, perirubral fibers, and rubrothalamic fibers (Carrillo-Ruiz et al. 2012). There also exist extended nuclei from mesencephalon that correspond to the substantia nigra (SN) and red nucleus (RN). The PSA is situated anterolateral of the red nucleus, posteromedial of the STN, inferior of the ventral thalamic nuclei, superior of the SN, and anteromedial of the posterior limb of the internal capsule (Xie et al. 2012; Fytagoridis et al. 2013a).
The Zi consists of four territories: rostral, dorsal, ventral, and caudal. Rostral Zi, which is attributed to visceral control, is located dorsomedial to the pallidofugal fibers and STN. Dorsal Zi is attributed to the wake and ventral Zi is under the guidance of the eye and head movements (Carrillo-Ruiz et al. 2012). The cZi, which is called the motor part of the Zi, extends posterior to the STN (Carrillo-Ruiz et al. 2012). The cZi is situated ventral to the Va thalamic nucleus, just ventral to the fascicularis thalamicus (Forel’s fields H), and dorsal to the fascicularis lenticularis (Forel’s fields H2) (Morel 2007; Plaha et al. 2008). Medial to the cZi is situated the cerebellothalamic tract and the red nucleus, and, lateral to the cZi, exists the internal capsule. The Zi receives inputs from motor, associative, and limbic cortices, the interpositus nucleus of the cerebellum, the substantia nigra reticulata (SNr), the globus pallidus internus (GPi), and the ascending reticular activating system, and it then connects to the centromedian and parafascicular (CM/Pf) and VL nuclei of the thalamus, SNr, GPi, the parvocellular RN, ION, the medial reticular formation, the pedunculopontine tegmental nucleus, the interpositus nucleus of the cerebellum, hypothalamus, the brainstem, including the pedunculopontine nucleus, the spinal cord, and the cerebral cortices (Carrillo-Ruiz et al. 2012; Heise and Mitrofanis 2004; Mitrofanis et al. 2004; Mitrofanis 2005; Plaha et al. 2008). cZI is an extension of the reticular thalamic nucleus, and it predominantly consists of GABAergic neurons. It affects both the basal ganglia and cerebellar outputs, medial reticular formation, and midbrain reticular formation, which are involved in controlling axial and proximal limb muscles (Blomstedt et al. 2009; Plaha et al. 2008). Current theories suggest that the cZi might play a key role in transmitting GABAergic input from the basal ganglia to the cerebellothalamocortical circuits and that DBS might alter or inhibit abnormal oscillations (Blomstedt et al. 2009; Fytagoridis et al. 2012; Plaha et al. 2008). Plaha et al. (2008) have suggested that Zi is an effective target for various types of tremors.
The Raprl is situated inferior to the Vo and VIM thalamic nuclei, in front of the lemniscus pathway, externally is the thalamic reticular nucleus, Zi, and STN, and the medial side is bordered by the RN (Carrillo-Ruiz et al. 2008, 2012; Lehman and Augustine 2013; Morel 2007). The Raprl contains cerebellothalamic, pallidothalamic, renticulothalamic, and rubrothalamic fibers (Carrillo-Ruiz et al. 2008, 2012).
11.3.2.2 Electrophysiological Findings During PSA DBS
When PSA DBS is performed, the transfrontal trajectory to the target is ~45° to the AC–PC plane, and an abrupt increase in impedance (from 400–500 to 600–700 Ω) is experienced when the electrode is passed beyond the ventral boundary of the thalamus into the subthalamic white matter (Murata et al. 2003; Plaha et al. 2008). Alternatively, single-unit-activity recordings have demonstrated frequencies of at least 15 Hz and/or responses to somatosensory stimulation or joint movements in the thalamus, while single-unit activity is absent or below 5 Hz with low background activity and does not respond to outer stimuli in the subthalamic area (Raprl) (Herzog et al. 2007; Kiss et al. 2003). In awake humans, late components of the somatosensory evoked potential from median nerve stimulation can be recorded from Raprl (Blomstedt et al. 2009). The extracellular action potential characteristics of the anterior thalamus and Zi are similar: low frequency and irregular discharge. The major difference is the neuronal density or the distance traversed before extracellular action potentials are encountered (Baker et al. 2004). In the Zi, the neuronal density is lower than that of the anterior thalamus. When the microelectrode enters into the STN, high frequency irregular discharges and increases in background activity are notably observed (Theodosopoulos et al. 2004). Just outside the bottom of the STN is an electrically quiet zone. When the microelectrode enters into the SNr, high frequency regular discharges with decreased background activity are detected.
11.3.3 Surgical Anatomy of the Subthalamic Area (STA) and STN
Herzog et al. (2007) have reported that most contacts that disrupt tremor-electromyography synchronization are located within the PSA, although they are still present in the VL thalamus. Stimulation of the PSA significantly increases the tremor frequency, which reflects a reduced contribution of the pathological central tremor oscillator (Deuschl and Bergman 2002; Herzog et al. 2007). The cerebellothalamic fibers pass through the lateral portion of the red nucleus and the bulk of fibers that continue in the rostrolateral direction pass through the field H of Forel and Raprl and enter the thalamus (Ilinsky and Kultas-Ilinsky 1984; Herzog et al. 2007). The fasciculus thalamicus (pallidothalamic tract) includes the fasciculus and ansa lenticularis (Forel H1 and H2, respectively). These two fibers merge and form the fasciculus thalamicus (Forel H) (Morel 2007). Therefore, the PSA is largely comprised of these two fibers: the cerebellothalamic fibers and Forel H. These fibers, especially the cerebellothalamic fibers, pass through the Zi and the Raprl (Morel 2007). Therefore, the effects of cZi stimulation on tremor might, in part, be due to the stimulation of cerebellothalamic and/or pallidothalamic fibers. In patients suffering from ET over the long term, the tremor and kinesthetic cells spread into Vo segments (Katayama et al. 2005; Kiss et al. 2003). The intention component of ET is supposed to be intensively affected by cerebellothalamic afferents to the VIM nucleus (Pedrosa et al. 2013). These facts suggest that it might be better to stimulate cerebellothalamic fibers themselves in order to alleviate ET. The most effective electrode might be situated in the border zone of the PSA and VIM for ET. This situation has been shown to be true for Parkinson’s disease (PD); the border zone of the cZi and the STN was most effective for DBS (Deuschl and Bergman 2002).
According to the stereotactic target of the STN proposed by Blomstedt et al. (2011a), the stimulation site is located at the border of the STN and fasciculus lenticularis from Morel’s atlas, and this location is close to the Zi and the fasciculus thalamicus. The effect of STN DBS might affect the tonic activation of adjacent tracts, as well as the cZi, which might lead to a tonic activation of VIM neurons and disrupt the tremor synchronous activity (Stover et al. 2005; Miocinovic et al. 2013). Stover et al. (2005) have postulated that this hypothesis is compatible with the results that the short-latency excitation of thalamic neurons begins just after STN stimulation in MPTP PD model monkeys. Lind et al. (2008) have reported that the most effective contacts are situated in the mid (56.3 %) and ventral (31.3 %) side of the STN.
11.4 Tentative Stereotactic Coordinates for DBS in Patients with ET
The current surgical targets for ET are the VIM (Fig. 11.1), STA (Fig. 11.2), and STN (Fig. 11.3). The tentative stereotactic coordinate for VIM DBS is 15 mm lateral to the midline at the level of the intercommissural line (ICL) and 6 mm anterior to the PC (Benabid et al. 1996). The Guiot approach that is widely used in France is the following: (11.0 or 11.5 + 1/2 or 1/3 of the third ventricular width) mm lateral to the midline at the level of the AC–PC plane and one-fourth of the AC–PC length anterior to the PC (Bardinet et al. 2011).
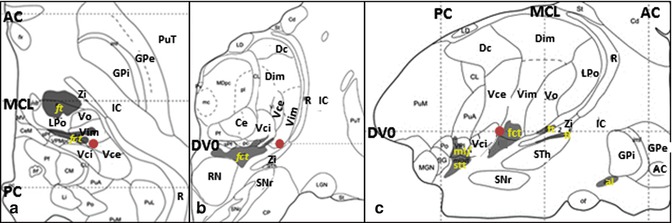
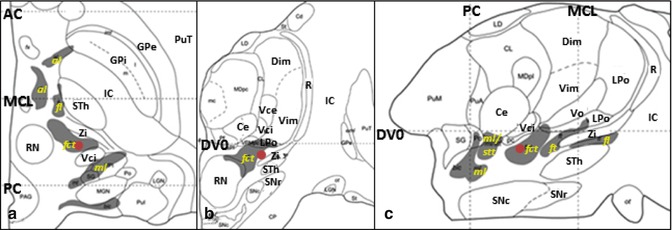
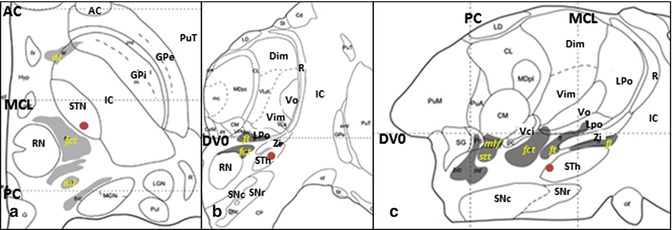
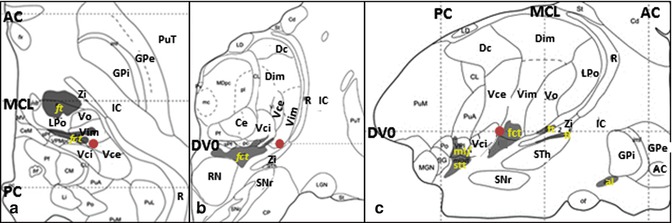
Fig. 11.1
Stereotactic coordinate modified from Morel’s atlas (2007) illustrating the location of the Vim nucleus (
). (a) Axial plane, on the AC–PC plane. (b) Coronal plane, 6 mm anterior to the PC. (c) Sagittal plane, 14.5 mm lateral to the median plane (From Morel (2007) with permission.) Abbreviations: AC anterior commissure, al ansa lenticularis, Ce nucleus centralis externus, Dc nucleus dorsocaudalis, Dim nucleus dorsointermedius, DVO AC-PC plane, fct fasciculus cerebello-thalamicus, fl fasciculus lenticularis, ft fasciculus thalamicus, GPi globus pallidus, internal segment, GPe globus pallidus, external segment, LPo nucleus lateropolaris thalami, stt spinothalamic tract, IC internal capsule, MCL midcommissural line, ml medial lemniscus, PC posterior commissure, PuT putamen, R reticular thalamic nucleus, RN red nucleus, SNr substantia nigra, pars reticulata, STh Subthalamic nucleus, Vce & Vci nucleus ventrocaudalis externus & internus, Vim nucleus ventrointermedius, Vo nucleus ventrooralis, Zi zona incerta

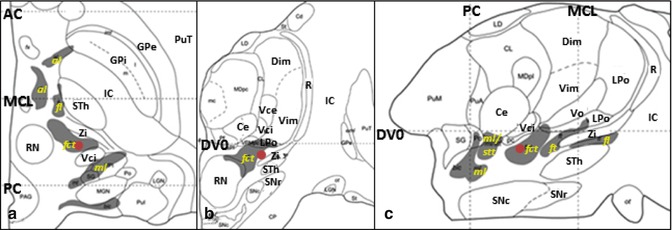
Fig. 11.2
Stereotactic coordinate modified from Morel’s atlas (2007) illustrating the location of the STA (
). (a) Axial plane, 2.7 mm ventral to the AC-PC plane. (b) Coronal plane, 7 mm anterior to the PC. (c) Sagittal plane, 11.8 mm lateral to the median plane (From Morel (2007) with permission.) Abbreviations: AC anterior commissure, al ansa lenticularis, Ce nucleus centralis externus, Dim nucleus dorsointermedius, DVO AC-PC plane, fct fasciculus cerebello-thalamicus, fl fasciculus lenticularis, ft fasciculus thalamicus, GPi globus pallidus, internal segment, GPe globus pallidus, external segment, LPo nucleus lateropolaris thalami, stt spinothalamic tract, IC internal capsule, MCL midcommissural line, ml medial lemniscus, PC posterior commissure, PuT putamen, R reticular thalamic nucleus, RN red nucleus, SNc substantia nigra, pars compacta, SNr substantia nigra, pars reticulata, STh Subthalamic nucleus, Vce & Vci nucleus ventrocaudalis externus & internus, Vim nucleus ventrointermedius, Vo nucleus ventrooralis, Zi zona incerta

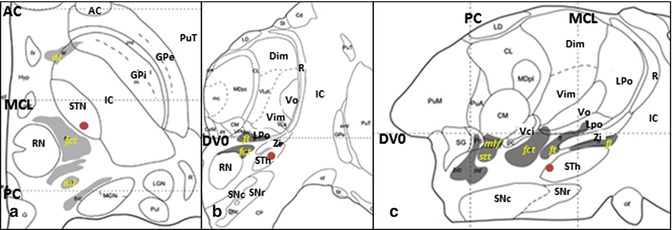
Fig. 11.3
Stereotactic coordinate modified from Morel’s atlas (2007) illustrating the location of the STh (
). (a) Axial plane, 3.6 mm ventral to the AC-PC plane. (b) Coronal plane, 9 mm anterior to the PC. (c) Sagittal plane, 11.8 mm lateral to the median plane (From Morel (2007) with permission.) Abbreviations: AC anterior commissure, al ansa lenticularis, Dim nucleus dorsointermedius, DVO AC-PC plane, fct fasciculus cerebello-thalamicus, fl fasciculus lenticularis, ft fasciculus thalamicus, GPi globus pallidus, internal segment, GPe globus pallidus, external segment, LPo nucleus lateropolaris thalami, stt spinothalamic tract, IC internal capsule, MCL midcommissural line, ml medial lemniscus, PC posterior commissure, PuT putamen, R reticular thalamic nucleus, RN red nucleus, SNc substantia nigra, pars compacta, SNr substantia nigra, pars reticulata, STh Subthalamic nucleus, Vci nucleus ventrocaudalis internus, Vim nucleus ventrointermedius, Vo nucleus ventrooralis, Zi zona incerta

In most studies, the PSA has been targeted directly (Murata et al. 2003; Plaha et al. 2008; Blomstedt et al. 2010). Murata et al. (2003) have reported the PSA coordinates as approximately 10 mm lateral and 3–4 mm behind the posterior border of the STN on the axial slice with the greatest STN diameter. Plaha et al. (2004) have targeted the point medial to the posterior dorsal third of the STN. Blomstedt et al. (2010) have targeted the cZi with thin-slice T2-weighted magnetic resonance images: slightly posteromedial to the tail of the STN at the level of the maximal diameter of the red nucleus. The stereotactic locations of the STA that best alleviated tremor (active contact) were 10.9–12.7 mm lateral to the midline, 6.1–7.0 mm posterior of the midcommissural line, and 1.5–3.0 mm below ICL (Murata et al. 2003; Hamel et al. 2007; Fytagoridis and Blomstedt 2010; Barbe et al. 2011). These targets are supposed to be located in the Raprl. Plaha et al. (2008) have targeted the cZi, and they reported that the stereotactic targets of the cZi were 14.2 mm lateral to the midline, 5.7 mm posterior to the mid-commissural line, and 2.1 mm below the ICL.
Blomstedt et al. (2011a, b) have targeted the STN with thin-slice T2-weighted images at the level of the maximal diameter of the red nucleus, at a line joining the anterior borders of these nuclei, and around 1.5 mm lateral from the visualized medial border of the STN. Lind et al. (2008) have reported that the position of active contact is 12 mm lateral to the midline, 16 mm posterior to the AC, and 4 mm below the ICL.
11.5 Definition of the Active Target During DBS Surgery
Intraoperative macrostimulation under local anesthesia is a reliable method to evaluate the effects of the optimal locations for each patient. The tremor stops immediately after the stimulation. Adverse events, such as dysesthesia and limb ataxia, which diminish within 10 s during the stimulation (200–300 Hz, 1 s trains of 0.1- or 0.2-ms pulse widths, the stimulation current is usually limited to a maximum of ~100 μA with high-impedance microelectrodes), are acceptable (El-Tahawy et al. 2004; Krack et al. 2002; Morigaki et al. 2010). Electrode model 3389 (Medtronic, Inc., Minneapolis, MN, USA) is usually used for VIM and STN, and model 3387 or 3389 is used for STA as it enabled stimulation of the VL thalamus alternatively (Plaha et al. 2004).
11.6 Stimulation Parameters
The mean parameters used in VIM, PSA, and STN DBS for ET are the following:
VIM DBS: 145–185 Hz (at least 100 Hz), 60–117 μs, 2.0–3.7 V (Koller et al. 1997, 1999, 2001; Pilitsis et al. 2008; Rehncrona et al. 2003; Tröster et al. 1999).
PSA DBS: 130–170 Hz (usually 130 Hz), 60–112.5 μs (usually 60 μs), 1.9–2.5 V (Blomstedt et al. 2010, 2011a; Fytagoridis et al. 2013a; Herzog et al. 2007; Murata et al. 2003; Plaha et al. 2004, 2011).
11.7 The Possible Mechanisms by Which DBS Alleviates Tremor
Several hypotheses have been proposed with regard to the mechanisms of high-frequency DBS. Conduction or depolarization block is the simplest hypothesis, and it is supported by the findings that ablation surgery has similar effects as DBS (Benabid et al. 1996). Activation of inhibitory presynaptic afferents and inhibition of projection neurons (Wu et al. 2001; Anderson et al. 2006), inhibition of neuronal activity near the stimulation site and activation of axonal elements that leave the target structure (Vitek 2002), activation of adjacent fiber tracts surrounding or running through the stimulated site (Johnson et al. 2009), and the superposition of continuous stimuli onto rhythmically oscillating subcortical–cortical loops (Montgomery and Baker 2000) are other hypotheses. Some authors have hypothesized that high-frequency stimulation in the VIM increases glutamate release from glial cells and excites local interneurons, thereby increasing the production of inhibitory neurotransmitters (e.g., GABA and glycine) and resulting in a decrease in the firing rates of projection neurons (Kang et al. 1998; Tawfik et al. 2010; Tian et al. 2005). When we consider the effect of VIM, as well as PSA (especially Rarpl) stimulation, the conduction or depolarization block might be a plausible mechanism. However, positron emission tomography analyses have demonstrated that VIM DBS increases regional blood flow in the supplemental motor area (Ceballos-Baumann et al. 2001; Perlmutter et al. 2002). Taking into consideration that the cerebellothalamic and thalamocortical fibers are both glutamatergic (excitatory), a different mechanism might be at work. DBS might modulate abnormal oscillation coupling by superposing continuous stimuli onto rhythmically oscillating loops (Montgomery & Baker’s theory) or activate adjacent or surrounding fiber components (Vitek’s or Johnson’s theory).
11.8 Patient Selection
Factors to consider in DBS include tremor severity, refractoriness to medication, tremor type, patient characteristics, including age, comorbid conditions, surgical risk, patient preference, social and employment factors, and social support (Cooper and Bowes 2012). One of the most important aspects is cognitive function. This is especially important for bilateral procedures, particularly bilateral STN DBS (Hariz 2002). Patients with borderline mental conditions have experienced irreversible and unadjustable dementia after bilateral STN DBS. Although most cases involve unilateral surgery (left side), VIM, Raprl, and cZi DBS have not been shown to affect higher cognitive processes as long as in 6-year follow-up periods (Heber et al. 2013).
Unilateral thalamotomy could be an option when a patient does not want to implant devices, a patient has difficulty in consulting medical care frequently, extensive lesioning is predicted due to a proximal inclusion, a patient has an increased risk of infection (immune-compromised patients), and habituation or tolerance occurs after DBS (Bahgat et al. 2013).
With regard to VIM DBS, tremor-suppressing medications could be discontinued after bilateral VIM DBS, but they should be continued after unilateral DBS. Unilateral VIM DBS is insufficient to eliminate the necessity for tremor-suppressing medications, and discontinuation has been shown to worsen the tremor (Favilla et al. 2013).
11.9 Therapeutic Impact of DBS for ET
In general, resting tremors are better controlled than action tremors, distal limb tremors are better than proximal limb tremors, and upper limb tremors are better than lower limb tremors (Benabid et al. 1996, 1998; Lozano 2000). The short- and long-term efficacy of VIM DBS is promising. However, tolerance to the stimulation and insufficient effects on axial and proximal tremor are the biggest problems. The PSA is an effective target for tremor suppression in early ablation surgery (Spiegel et al. 1963). Although there are not enough results concerning long-term efficacy, PSA DBS seems promising in a number of recent publications. Moreover, PSA stimulation showed a powerful effect, even for axial and proximal tremor, and several short-term and a few long-term results have described that ET patients with PSA DBS did not manifest tolerance to the stimulation. Here, we review the short- and long-term outcomes that are related to VIM, PSA (Raprl & cZi), and STN DBS for patients with ET.
11.9.1 Therapeutic Impact of VIM DBS
Only one randomized controlled trial with a direct comparison of thalamotomy with DBS is available (Schuurman et al. 2000, 2008). The results demonstrated that VIM DBS was equally effective as VIM thalamotomy. However, half of the ET patients experienced diminished effects of the stimulation over the 5-year follow-up (Schuurman et al. 2008).
11.9.1.1 Short-Term Efficacy
Tremor Reduction (Assessors Blind)
Numerous case series are available, and the short- and long-term effects of VIM DBS are usually favorable (Morigaki et al. 2010). Among them, blinded assessments have shown a marked reduction in tremor scores and significant improvements in disability scores (Koller et al. 1997, 1999, 2001; Lyons et al. 1998; Pahwa et al. 1999; Tröster et al. 1999; Schuurman et al. 2000; Hariz 2002; Rehncrona et al. 2003; Fields et al. 2003).
Schuurman et al. (2000) have reported that the contralateral tremors of 13 ET patients were completely suppressed at 6 months postoperatively. In Koller’s series (Koller et al. 1997), VIM stimulation resulted in 31 % of the ET patients obtaining contralateral tremor remission, and 79 % of the ET patients experienced marked tremor improvement (50–100 %) at the 3-month follow-up. Hariz et al. (2002) have reported that unilateral VIM DBS improved the total Fahn-Tolosa-Marin (FTM)-tremor rating scale (TRS) tremor score by 47 % from baseline at the 12-month follow-up. Unilateral VIM DBS improved the FTM-TRS tremor scores by 50.8–78.0, 53–78.7, and 20.6 % from baseline at the 3-, 12-, and 24-month follow-ups, respectively (Fields et al. 2003; Hariz 2002; Rehncrona et al. 2003; Tröster et al. 1999). The improvement in upper limb tremors contralateral to the DBS improved 79 % at the 12-month follow-up (Hariz 2002). Both the contralateral upper postural and activity/intention components improved 66.7 % at the 24-month follow-up (Rehncrona et al. 2003). Bilateral VIM DBS improved the FTM-TRS motor scores (items 1–10) and the postural + intention tremor by 64.9 and 66.7 %, respectively, at the 3-month follow-up (Pahwa et al. 1999). Head tremor was improved in 71 % of patients (Koller et al. 1999).
Although the assessors were not blind, several publications have indicated the efficacy of bilateral VIM DBS for axial tremors (Koller et al. 1999; Limousin et al. 1999; Obwegeser et al. 2000; Ondo et al. 1998; Putzke et al. 2005; Sydow et al. 2003; Taha et al. 1999). However, its therapeutic efficacy has been reported to vary. With respect to voice tremor, some studies have reported a significant and marked (more than 80 %) improvement (Obwegeser et al. 2000; Taha et al. 1999), whereas others have reported minimal changes in either short- or long-term follow-up studies (Limousin et al. 1999; Putzke et al. 2005; Sydow et al. 2003).
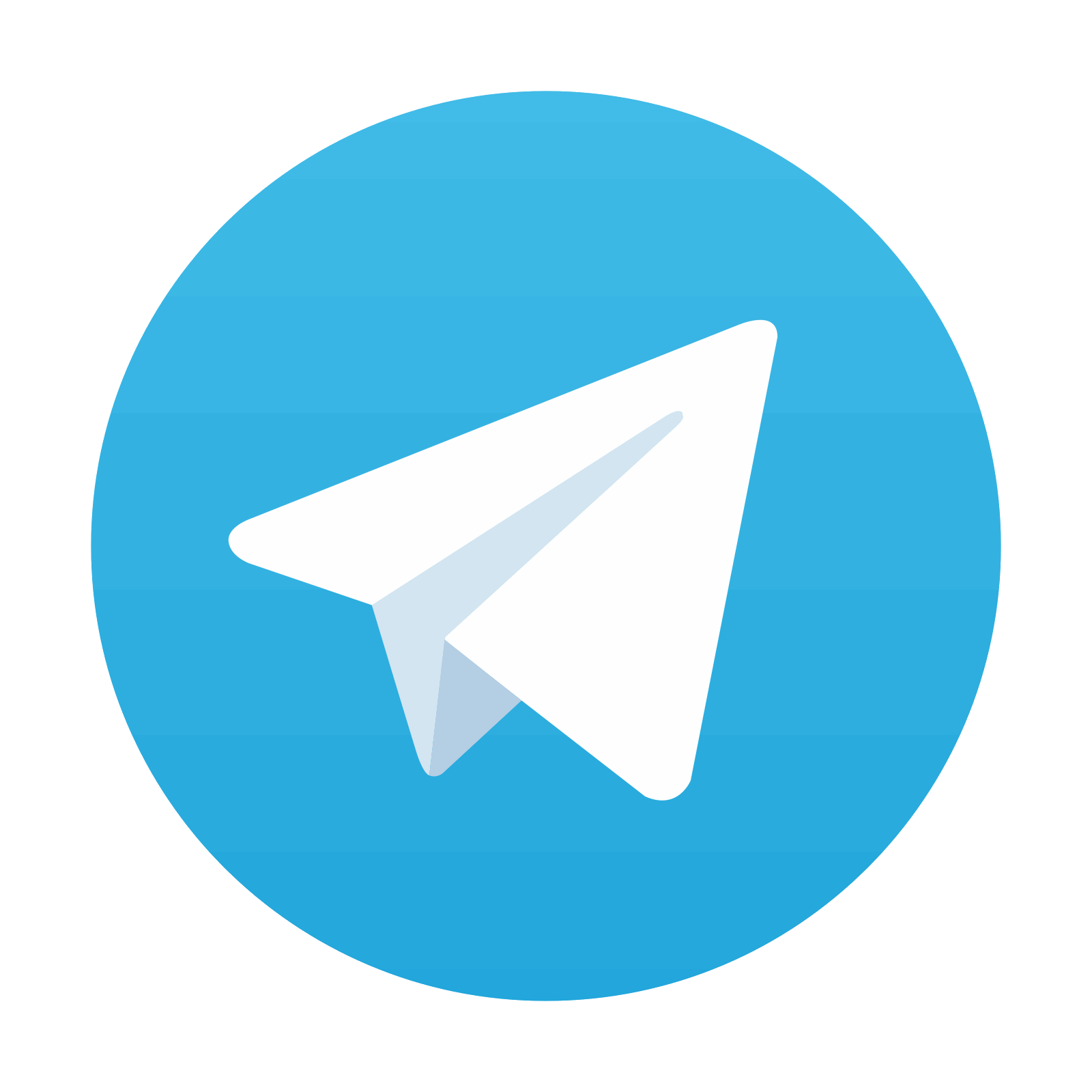
Stay updated, free articles. Join our Telegram channel

Full access? Get Clinical Tree
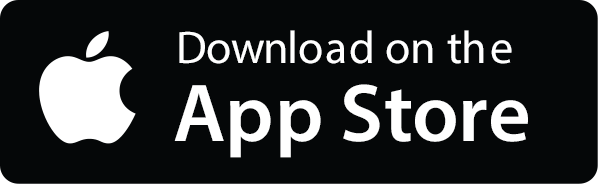
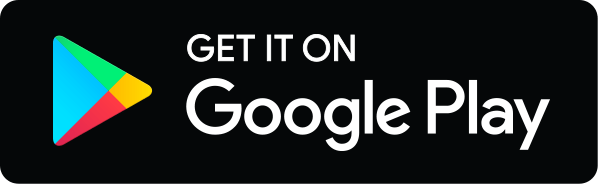
