I may speak alike to you and my own conscious heart.
I. EVALUATION OF CONSCIOUSNESS
A. Two definitions of consciousness: intuitive and operational
1. Intuitively, we define consciousness as the awareness of self and environment. Or, more introspectively, we define consciousness as the awareness of our sensorium.
2. Operationally, physicians determine consciousness by practical steps.
B. Operations that establish consciousness
Physicians customarily employ inspection, verbal stimuli, and, if necessary, pain to determine the patient’s (Pt’s) awareness of self and environment.
1. Inspection: Does the Pt appear to adapt appropriately to the ongoing visual, auditory, and tactile stimuli of the ordinary environment?
2. Verbal stimuli: Does the Pt respond appropriately to inquiries and requests?
3. Pain: Does the Pt respond appropriately to pain?
4. For proof of conscious awareness, the Pt must have a receptor and its sensory pathway intact enough to receive stimuli and deliver those stimuli to the cerebrum. Then the Pt must have a motor pathway, neuromuscular junction, and an effector intact enough to produce a volitional behavior, verbal or nonverbal, that depends on consciousness. The examiner (Ex) cannot determine consciousness by clinical tests in a curarized Pt on the intensive care unit, a Pt with severe Guillain–Barré syndrome (GBS), or a Pt with bilateral hemiplegia, who lacks an intact effector system. See, however, the locked-in syndrome, pages 500–501.
C. Pathologic alterations in the level of consciousness
1. Disease may alter consciousness by causing various stages of delirium or coma.
2. Delirium (acute confusional state): Delirium means an acute, transient confusional state characterized by global impairment of the sensorium. The Pt shows disorientation, amnesia, misperceptions, hallucinations, (often vivid) delusions, brief attention span, disconnected thoughts, irrational or incoherent mutterings, and abnormally decreased or increased psychomotor activity (agitation). Rating scales enable a quantitative assessment (Trzepacz et al, 2002). The sleep and wake periods fail. Any such state of excessive neuronal activity may eventually cause tremors and convulsions (Diagnostic and Statistical Manual of Mental Disorders, 4th ed., 1994; Lipowski, 1989; Victor and Ropper, 2001). The delirious Pts then return to their previous mental state, or delirium may precede coma. Demented Pts may have periods of superimposed delirium. In aged and demented persons, delirium occurs most commonly at night. The causes of delirium include intracranial hemorrhage, infection, sleep deprivation, various drugs or withdrawal from drugs, toxic or metabolic states, and fever. Withdrawal from alcohol or other depressant drugs causes withdrawal delirium (delirium tremens), the classic example of delirium with excitement and often seizures (Chapter 11).
3. Coma (from the Greek word Koma meaning deep sleep) is sustained pathologic unconsciousness resulting from dysfunction of the ascending reticular activating system (ARAS) in the brainstem tegmentum or both cerebral hemispheres. Comatose patients cannot be aroused and their eyes remain closed (The Multi-Society Task Force on PVS, 1994).
4. Depression of consciousness may evolve through several stages or come on abruptly.
5. Recite the minimum neuroanatomic connections necessary for a valid bedside appraisal of consciousness.
_________
_________
D. The Glasgow Coma Scale (GCS) for tracking the level of depressed consciousness
1. A grading scale forces the Ex to observe systematically and accurately. The necessity to grade a response, as in eliciting muscle stretch reflexes (MSRs), makes you observe better than if you do not face such a reckoning.
Depend on it, sir, when a man knows he is to be hanged in a fortnight, it concentrates his mind wonderfully.
2. For the Glasgow Coma Scale (total score 3-15), the Ex periodically grades eye opening from 1 to 4, the best verbal response from 1 to 5, and the best motor response to pain from 1 to 6 (Teasdale and Jennett, 1974). The trend lines derived from these simple tests readily disclose deterioration or improvement. In Fig. 12-1, the trend lines document a declining level of consciousness in a Pt with cerebral edema. After the Pt receives intravenous mannitol, a hyperosmolar agent that reduces edema, the trend lines document the abrupt improvement.
FIGURE 12-1. The Glasgow Coma Scale (GCS). Notice the declining level of consciousness until after mannitol treatment, which reduces brain edema.
3. Like the Apgar score, the total score for the three categories provides a prognosis (Bates, 1991; Evans, 1976; Teasdale et al, 1978). A GCS score from 3 to 8 implies a poor prognosis (Levin et al, 1991). The GCS should report the total score as well as the individual scores for each of the three items of the scale. Valuable as these scales are, some interexaminer variability occurs (Newton et al, 1995; Wiese, 2003).
4. In some intensive care unit Pts with the eyes swollen shut and who are sedated and intubated, the GCS is difficult to apply. Besides, the GCS is insensitive to brainstem function (lack of assessment of pupillary size and reactivity). Thus, modifications of the GCS and new proposals for more reliable coma scales have since been proposed but not universally accepted. The Full Outline of UnResponsiveness (FOUR) score scale (Table 12-1; Wijdicks et al, 2005), proposed as an alternative to the GCS’s, a new scale for improved assessment of coma. The scale has been validated in different settings (Iyer et al, 2009; Stead et al, 2008), and it consists of four components (Eye Response, Motor Response, Brainstem Reflexes, and Respiratory Pattern). The total score is 0 to 16, and each component has a maximal score of 4.
TABLE 12-1 • Full Outline of UnResponsiveness (FOUR) Scale
Eye Response 4 = eyelids open or opened, tracking, or blinking to command 3 = eyelids open but not tracking 2 = eyelids closed but open to loud voice 1 = eyelids closed but open to pain 0 = eyelids remain closed with pain Motor Response 4 = thumbs-up, fist, or peace sign 3 = localizing to pain 2 = flexion response to pain 1 = extension response to pain 0 = no response to pain or generalized myoclonus status Brainstem Reflexes 4 = pupil and corneal reflexes present 3 = one pupil wide and fixed 2 = pupil or corneal reflexes absent 1 = pupil and corneal reflexes absent 0 = absent pupil, corneal, and cough reflex Respiration 4 = not intubated, regular breathing pattern 3 = not intubated, Cheyne–Stokes breathing pattern 2 = not intubated, irregular breathing 1 = breathes above ventilator rate 0 = breathes at ventilator rate or apnea |
SOURCE: Wijdicks et al., 2005. |
II. ANATOMIC BASIS OF CONSCIOUSNESS
A. Parts of the neuraxis unnecessary for consciousness
1. Operationally, the question of the location of consciousness boils down to whether we can locate neuronal circuitry that must be active for consciousness and that, if destroyed, impairs or abolishes consciousness. To identify the parts of the CNS necessary for consciousness, we will first cut away and discard the parts unnecessary for consciousness. To ensure that you know the gross parts of the CNS, make an enlarged drawing of Fig. 2-1 on a loose sheet of paper. Then use scissors to cut away the parts of your drawing, as described next in the text, and place these parts in a pile.
2. First excise the entire spinal cord. Next excise the cerebellum. Then excise the medulla and caudal half of the pons. None of these parts is necessary for consciousness. (Actually cut these parts off of your drawing. This seeming busy work will lead to permanent retention.) Complete transection of the neuraxis at any level from the sacral tip of the cord to the midpons spares consciousness, if we artificially maintain respiration and blood pressure. The pile of parts discarded as unnecessary for consciousness now contains the entire spinal cord, medulla, caudal half of the pons, and the cerebellum. Next, however, complete transection of the brainstem in the upper pons will temporarily abolish consciousness, whereas complete midbrain transection will permanently abolish consciousness.
3. Next, instead of complete transections of the pons and midbrain, let us make partial transections to determine what must remain to support consciousness. To appreciate the partial cuts, review the cross-sectional anatomy of the brainstem by drawing the generalized cross section of the brainstem (Figs. 2-13 and 2-14). Actually cut the parts off of your drawing as called for in the text.
4. We find that complete transection or even removal of the entire basis of the midbrain or pons bilaterally does not abolish consciousness. If we start with a completely intact nervous system, bilateral destruction of the basis of the pons or midbrain spares vertical eye movements but causes complete paralysis of all other volitional movements (Chapter 5). The Pt retains full sensation and full consciousness but can communicate that consciousness by the only available effector mechanism, the vertical eye movements (see the locked-in syndrome). Now snip off the brainstem basis bilaterally and place it on the discard heap.
5. After transecting the basis, we can insert the knife blade a little deeper to transect the medial and lateral lemnisci. The Pt loses the sensations mediated by these pathways, but consciousness remains. Next transect the tectum. Consciousness remains. Next, core out the cranial nerve motor nuclei. Consciousness remains. Thus, from your cross-sectional drawing of the brainstem, cut away the tectum, lemnisci, and cranial nerve nuclei and add them to the discard heap.
6. Now transection of the tegmentum bilaterally between the midpons and rostral midbrain abruptly abolishes consciousness. For consciousness, the midbrain and rostral half of the pontine tegmentum must remain intact (Reznick, 1983) and in continuity with the cerebrum and the diencephalon. Except for the rostral half of the pontomesencephalic tegmentum, we have discarded all other parts of the neuraxis caudal to the diencephalon.
7. From your original drawing, you now have left in your hand a cerebrum in continuity with the diencephalon and pontomesencephalic tegmentum. Now we will determine the role of the remaining parts of the CNS in consciousness by transecting the diencephalon and basal ganglia at successively more rostral levels. We must insert the knife from the bottom of the cerebrum to transect these gray masses bilaterally without damaging the surrounding white matter or cortex. Bilateral transection of the diencephalon permanently and irreversibly abolishes consciousness. As we extend more rostrally into the basal ganglia, the evidence becomes a little less secure because of the lack of pure lesions in human disease; however, early in the history of surgery on the basal ganglia and diencephalon to treat involuntary movement syndromes, neurosurgeons learned not to make bilateral lesions because of the impairment of mentation, consciousness, and speech. Tentatively, we can state that acute bilateral destruction of the globus pallidus and striatum abolishes consciousness—at least, if the lesion extends, as it usually does, a little into the neighboring diencephalon or septal region, or into the neighboring medial hemispheric wall (Freemon, 1971). Thus, we find that bilateral lesions at any level, from the pontomesencephalic tegmentum up through the diencephalon and basal ganglia to the medial hemispheric wall, abolish consciousness (Figs. 12-2 and 12-3).
FIGURE 12-2. Sagittal section of the brain. The stippling shows the part of the pontomesencephalic tegmentum, diencephalon, and basal forebrain in which bilateral lesions abolish consciousness.
FIGURE 12-3. Coronal sections of the midbrain (A) and the cerebrum (B) at the level of the caudate nuclei. The shaded area shows the sites and comparative sizes of lesions that abolish consciousness.
8. Notice in Figs. 12-2 and 12-3 that, because the lesions involve structures rostral to the midbrain, in particular the basal ganglia and medial hemispheric wall, they must become increasingly larger than those required in the pontomesencephalic tegmentum and diencephalon.
9. The next anatomic region consists of the deep white matter surrounding the diencephalon and basal ganglia, which conveys axonal circuits between those neuronal masses and the cortical neurons. If we destroy the deep white matter of one hemisphere, scrape off all of its cerebral cortex, or remove the white matter and cortex by a hemispherectomy, the Pt can retain consciousness. (However, large acute lesions of a hemisphere temporarily impair consciousness. Curiously, left hemisphere lesions impair consciousness about twice as often as right hemisphere lesions [Albert et al, 1978]). Actually trace a hemisphere from Fig. 12-2, cut it out, and add it to the discard heap. The discard heap of the gross parts unnecessary for consciousness thus includes:
_________
_________
10. Although one of the two cerebral hemispheres is dispensable, we cannot dispense with both. We can discard any pair of the frontal, parietal, occipital, and temporal lobes. We profoundly alter personality and sensorimotor functions, but consciousness per se remains. But if we remove too much of the cerebrum bilaterally, scrape the cerebral cortex off both hemispheres, or destroy much or all of the cortex by hypoxia or hypoglycemia, we produce an apallic syndrome, an old term for a condition that is now considered equivalent to a persistent vegetative state (Dalle et al, 1977). If we suck out the deep white matter from both hemispheres, or if the Pt has a severe demyelinating disease, thus disconnecting the cortical shell from the brainstem and diencephalon, the Pt permanently loses consciousness. Such severe, bilateral destructive decorticating or demyelinating lesions stand in direct contrast to the tiny, confined, bilateral pontomesencephalic tegmental lesions that exquisitely and selectively abolish consciousness, with little effect on functions mediated through other pathways. Review Fig. 12-3, which shows the location in the midbrain tegmentum of the smallest, most discrete lesion that will permanently abolish consciousness.
B. Summary of the parts of the neuraxis necessary and unnecessary for consciousness
1. The previous observations show that full consciousness requires the pontomesencephalic tegmentum of at least one-half of the brainstem and the ipsilateral diencephalon and some of the ipsilateral cerebral hemisphere. This may, just may, constitute the minimal amount of brain required to function as a person. Although a necessary condition for consciousness, an intact pontomesencephalic tegmentum alone is not a sufficient condition or at least not sufficient to provide operational evidence of consciousness. That requires, in addition, a cerebral hemisphere or most of a hemisphere with an output pathway to some effector to produce behavioral responses that signify consciousness. What is not expendable bilaterally without profoundly affecting or abolishing consciousness is
a. Medial hemispheric wall down to and including basal forebrain
b. Caudate–putamen (striatum)
c. Diencephalon
d. Midbrain tegmentum: Bilateral tegmental lesions of small size completely and permanently abolish consciousness
e. Rostral pontine tegmentum
2. To justify your having learned the structures unnecessary for consciousness, note this supreme fact: If your Pt has a lesion confined to one of these structures and is or becomes unconscious, something else has caused the unconsciousness. The usual cause is that the lesion has herniated or shifted the brain to compress the structures indispensable for consciousness. Without medical or surgical intervention, the Pt will die. Section III discusses these brain herniations and their clinical recognition.
C. To prove your knowledge of the neuroanatomic basis of consciousness
1. Enumerate the parts of the neuraxis that we can discard without abolishing consciousness.
_________
_________
2. Enumerate the various sites of lesions within the neuraxis that will abolish consciousness. Assume artificial support of breathing and blood pressure to ensure that the neural lesion causes loss of consciousness, not hypoxia or ischemia.
_________
_________
3. State where to put the smallest lesion that will most selectively abolish consciousness.
_________
_________
D. Operational demonstration of the pathways for consciousness
1. Insert a stimulating electrode into the midbrain reticular formation of an animal.
2. Apply recording electrodes over the scalp. The record obtained from the scalp electrodes is called an electroencephalogram (EEG).
3. After the animal goes to sleep, stimulate the reticular formation and observe:
a. That the animal opens its eyes and looks around.
b. That the EEG shows a distinct change in the electrical activity of the brain (Fig. 12-4).
FIGURE 12-4. A sleeping animal with an electrode inserted into the midbrain reticular formation is hooked to an EEG machine. Stimulation of the electrode causes an abrupt awakening. S marks the point of stimulation during the EEG recording. Notice the abrupt change in the entire EEG from the high-amplitude slow waves of sleep to the low-amplitude fast activity of the waking state. EEG = electroencephalography.
4. After having awakened the animal with a stimulus to the reticular formation, the experimenter can greatly increase the current to make an electrolytic lesion around the tip of the electrode. The animal will lapse into unconsciousness.
5. Similar stimulation of the midline and intralaminar nuclei of the thalamus will produce an alerting response. Bilateral destruction abolishes consciousness.
6. Interpretation of the experiment: Demonstration of a nonspecific ascending pathway.
a. The initial stimulation through the electrode was like throwing on a master switch: The entire cortex lit up. Because the entire cortex responded, we say that a diffuse or nonspecific ascending pathway has been stimulated. Because these impulses run through the thalamus, we conclude that the experiment demonstrates an ascending reticulo–thalamocortical pathway. We call this entire pathway the ascending reticular activating system (ARAS). Although the physiologic evidence points to a thalamocortical projection of the ARAS, the actual axons have yet to be fully demonstrated. The axons of some of the chemically specified reticular formation nuclei that belong to the ARAS bypass the thalamic nuclei.
b. Notice that the operational definition of the ARAS depends on two lines of evidence: Stimulation of the system heightens/
decreases consciousness, whereas destruction
heightens/
decreases consciousness. (
heightens;
decreases)
7. Give an operational definition of the ARAS based on the effects of stimulation or destruction._________
_________
E. Demonstration of specific thalamocortical pathways
1. The thalamocortical pathways of the sensory nuclei have a very specific, point-to-point relation to the cerebral cortex, in contrast to the ARAS, which projects diffusely or nonspecifically. To demonstrate this contrasting, specific system, do the following:
a. Insert a stimulating electrode into one of the sensory relay nuclei of the thalamus.
b. Apply recording electrodes over the cortical receptive area of the nucleus. In Fig. 12-5, the stimulating electrode is inserted into the nucleus ventralis posterior, and the recording electrodes are placed over the somesthetic receptive area in the postcentral gyrus. Study Fig. 12-5.
FIGURE 12-5. An electrode stimulated after insertion into one specific site in a thalamic somatosensory relay nucleus causes only one specific point of cortical excitation, in lead 3 of the electroencephalogram, rather than a generalized responses as in Fig. 12-4.
2. The specific systems are so specific that stimulation of minute retinal areas, stimulation of the cochlea by discrete sound frequencies, or stimulation of the skin of the individual digits evokes a response in a tiny, specific cortical sensory area. Review the specific thalamic sensory relay nuclei and their cortical projection areas in Table 12-2.
TABLE 12-2 • Specific Sensory Relay Nuclei of the Thalamus
Modality | Thalamic nucleus | Cortical receptive area |
Vision | Calcarine cortex in _________ | |
Hearing | Superior temporal gyrus in _________ | |
Somatic sensation | Postcentral gyrus in _________ |
(lateral geniculate body; occipital-medial geniculate body; temporalnucleus ventralis posterior; parietal)
3. The specific/
nonspecific pathways of the ARAS mediate the general state of consciousness, whereas the
specific/
nonspecific thalamocortical pathways mediate consciousness of particular sensory events. (
nonspecific;
specific)
BIBLIOGRAPHY · Consciousness
Albert ML, Silverberg R, Reches A, et al. Cerebral dominance for consciousness. Arch Neurol. 1978;33:453–454.
Bates D. Defining prognosis in medical coma. J Neurol Neurosurg Psychiatry. 1991;54:569–571.
Dalle OG, Gerstenbrand F, Lucking CF, et al. The apallic syndrome. Berlin, Germany: Springer-Verlag; 1977.
Evans BM. Patterns of arousal in comatose patients. J Neurol Neurosurg Psychiatry. 1976;39:392–402.
Freemon FR. Akinetic mutism and bilateral anterior cerebral artery occlusion. J Neurol Neurosurg Psychiatry. 1971;34:693–698.
Iyer VN, Mandrekar JN, Danielson RD, et al. Validity of the FOUR score coma scale in the medical intensive care unit. Mayo Clin Proc. 2009;84(8):694–701.
Levin HS, Williams DH, Eisenberg HM. Serial MRI and neurobehavioral findings after mild to moderate closed head injury. J Neurol Neurosurg Psychiatry. 1991;55:255–262.
Lipowski ZJ. Delirium in the elderly patient. N Engl J Med. 1989;52:578–582.
Newton CRJC, Kirkham FJ, Johnston B. Inter-observer agreement of the assessment of coma scales and brainstem signs in non-traumatic coma. Dev Med Child Neurol. 1995;37:807–813.
Posner JB, Saper CB, Schiff ND, et al. Plum and Posner’s Diagnosis of Stupor and Coma. Oxford: Oxford Univ. Press; 2007.
Plum F, Posner J. The Diagnosis of Stupor and Coma. 3rd ed. Philadelphia, PA: FA Davis; 1980.
Reznick M. Neuropathology of seven cases of locked-in syndrome. J Med Sci. 1983;60:67–68.
Stead LG, Wijdicks EFM, Bhagra A, et al. Validation of a new coma scale, the FOUR Score, in the Emergency Department. Neurocritical Care. 2008;10(1);50–54.
Teasdale G. Jennett B. Assessment of coma and impaired consciousness: a practical scale. Lancet. 1974;2:81–84.
Teasdale G, Knill-Jones R, van der Sande J. Observer variability in assessing impaired consciousness and coma. J Neurol Neurosurg Psychiatry. 1978;41:603–610.
The Multi-Society Task Force on PVS. Medical aspects of the persistent vegetative state—first of two parts. N Engl J Med. 1994;330:1499–1508.
Trzepacz PT, Meagher DJ, Wise MG. Neuropsychiatric aspects of delirium. In: Yudofsky SC, Hales RE, eds. Textbook of Neuropsychiatry and Clinical Neuroscience. 4th ed. Washington, DC: American Psychiatric Publishing; 2002, Chap. 14, 525–564.
Victor M, Ropper AM. Adams and Victor’s Principles of Neurology. 7th ed. New York; NY: McGraw-Hill; 2001.
Wiese MF. British Hospitals and different versions of the Glasgow Coma Scale: telephone survey. BMJ. 2003;327(418);782–783.
Wijdicks EFM, Bamlet WR, Maramattom BV, et al. Validation of a new coma scale. The FOUR Score. Ann Neurol. 2005;58:585–593.
III. INTERNAL HERNIATIONS OF THE BRAIN: EFFECT ON CONSCIOUSNESS, NEUROLOGIC FUNCTION, AND VASCULAR SYSTEM
A. Causes and consequences of internal herniations
The most common causes of internal herniations of the brain are cerebral contusions, hematomas, abscesses, neoplasms, and cerebral edema. The mass effect of these lesions increases the intracranial pressure (ICP) and causes internal shifts or herniations of the brain that compress the normal tissue, in particular the diencephalon and brainstem (Cuneo et al, 1979; Davis and Robertson, 1991; Posner et al, 2007; Sunderland, 1958; Walker, 1963). This compression impairs consciousness and the life-sustaining functions of breathing, blood pressure control, and temperature regulation. Herniations also compress cerebral arteries, resulting in infarctions.
B. Anatomy of the intracranial partitions and compartments
To understand how space-occupying lesions act to cause coma or kill Pts, learn Figs. 12-6 to 12-8, and complete the subsequent exercises.
FIGURE 12-6. Basket-handle dissection of the skull and removal of the cerebral hemispheres. Notice that the flax cerebri and tentorium cerebella, folds of the dura mater, partition the intracracterior cerebral arteries course along the ventral edge of the midbrain and extend laterally to cross the free edge of the tentorium to reach the temporo-occipital portions of the cerebrum. Locate the IIIrd nerve issuing from under the posterior cerebral artery. See also Fig. 12-7.
FIGURE 12-7. Base of the skull after removal of the basket handle and its attached falx cerebri. The mesencephalon remains in situ, as in Fig. 12-6. Notice the IIIrd nerve traveling under the PCA = Notice that the cerebellar vermis occupies the apex of the tentorial opening. The posterior fossa is under the tentorium. PCA posterior cerebral artery. (Adapted with permission from Plum F, Posner J. The Diagnosis of Stupor and Coma. 3rd ed. Philadelphia, PA: FA Davis; 1980.)
FIGURE 12-8. Coronal section of the head; ventral view of the brainstem and cerebrum. A large epidural hematoma has shifted the brain from right to left. Notice the herniation of the right uncus over the tentorial edge. Follow along the vertebral and basilar arteries to their terminal branches, the posterior cerebral arteries. Notice how the posterior cerebral artery on the right impinges on the IIIrd nerve. (Adapted with permission from Chapter 8. Head and Neck. In: Hansen, J. T. Netter’s Clinical Anatomy 3E. Philadelphia, PA: Saunders/Elsevier, 2014.)
1. The cranial cavity is divided by tough, dural partitions called the _________
2. The dural partition that divides the supratentorial space into right and left halves is the _________
3. The tentorium cerebelli forms a tent over the cerebellar hemispheres by inserting itself between the cerebellar hemispheres below and the _________
4. The space between the free, medial edges of the tentorial halves is called the tentorial _________
5. The part of the cerebellum that protrudes through the tentorial notch is the superior tip of the _________
6. The tentorial notch surrounds the pons/
mesencephalon/
diencephalon. (
mesencephalon)
7. In Figs. 12-6 and 12-7 notice that:
a. The transected brainstem remains in situ after removal of the cerebral hemispheres and diencephalon. Notice that the posterior cerebral artery passes over the free edge of the tentorial notch to reach the temporo-occipital portions of the cerebrum.
b. CrN III issues from the mesencephalon and passes under the posterior cerebral artery.
C. Responses of the fluid pools of the intracranial space to increased pressure
1. Water comprises 75% of the brain. Water is physically incompressible and biologically relatively immobile, although in cerebral edema it may increase the brain volume by 20% to 30%. This relatively immobile brain water contrasts with the two rapidly mobile liquid pools of the intracranial space, the intravascular blood and the cerebrospinal fluid (CSF). If brain tissue swells, what will happen to the lumen of the veins and capillaries? _________
2. After studying Figs. 12-8 and 12-9, describe what pressure does to the ventricles, sulci, and subarachnoid spaces of a swollen hemisphere. _________
FIGURE 12-9. Coronal section through the falx and adjacent portions of the cerebrum along the interhemispheric fissure. Notice that the swollen hemisphere (reader’s left) has collapsed the subarachnoid space and that the cingulate gyrus has begun to herniate under the free, inferior edge of the falx, impinging on the anterior cerebral artery.
3. Thus, the first compensation for increased pressure is a reduction in the two rapidly mobile intracranial fluid pools, the _________
4. The infant has additional ways to compensate for increased intracranial pressure, manifested by the following physical signs:
_________
_________
5. As the skull matures, it no longer yields to increased intracranial pressure. If you find split sutures on a skull radiograph of an 18-year-old Pt, the increased pressure must have started before the age of _________
6. If the hemispheric swelling exceeds the compensatory mechanisms in an infant or adult, the affected tissue can only respond by herniating. Study Fig. 12-8 and state the only two places the shifting hemisphere can go _________
_________
7. Because part of the swollen hemisphere has shifted under the falx cerebri, this shift is called _________
D. Anatomy of transfalcine herniation
1. The part of the hemisphere that shifts under the falx is called the _________
2. The anterior cerebral arteries run parallel to the free edge of the falx. When the cingulate gyrus herniates, it may compress the artery against the free edge of the falx, causing infarction of the medial hemispheric wall dorsal to the corpus callosum (Fig. 12-9).
3. In view of the representation of body parts in the motor cortex on the medial aspect of a hemisphere (Fig. 2-2C), this infarction would cause upper motoneuron (UMN) paralysis of the leg/
arm/
face/
leg, arm, and face. (
leg)
E. Anatomy of transtentorial herniation of the cerebrum
1. With a space-occupying lesion in one hemicranium, the bony wall of the calvarium prevents herniation or decompression outward. The cerebral hemisphere can herniate only medially or downward (Fig. 12-8).
a. The dural membrane that opposes medial shift of the cerebrum is the _________
b. The dural membrane that opposes downward shift of the cerebrum is the _________
2. Trans means over or across, hence, trans continental. The shifting of a swollen hemisphere across the free edge of the tentorium cerebelli is called _________
3. Hence, the two internal herniations of a swollen hemisphere are _________
4. The parts that undergo transtentorial herniation are the medial parts of the temporal lobe, namely the _________
5. Figure 12-10 shows a brain removed at autopsy. Fill in the labels and, in the blanks below, describe what is wrong.
_________
FIGURE 12-10. Ventral view of the cerebrum with the tentorium cerebelli reflected on the left side. Complete the blanks on the right side. (Redrawn from Peele T. The Neuroanatomic Basis for Clinical Neurology, 2nd ed. New York, McGraw-Hill, 1961.)
6. In coursing from the basilar artery to the cerebrum, the posterior cerebral artery crosses the free edge of the falx, where the herniation may compress it (Figs. 12-6 and 12-7 and Table 12-5). This artery irrigates the medial part of the temporo-occipital region and the occipital pole. Infarction of this region, particularly on the right, would cause two ophthalmologic disorders, _________
F. Effect of transtentorial and subfalcine herniation on consciousness
Because subfalcine and transtentorial herniations compress the medial hemispheric wall, diencephalon, and mesencephalon, they interfere with the ARAS and alter the Pt’s level of consciousness. Medial shift of the brain alone may impair consciousness (Fisher, 1995; Inao et al, 2001; Pullicino et al, 1997; Ropper, 1993) but, if combined with transtentorial herniation, compresses the brainstem and may kill the Pt.
G. Effect of transtentorial herniation on cranial nerve III
1. As transtentorial herniation increases, the uncus displaces the posterior cerebral artery and CrN III. CrN III contains parasympathetic fibers that, when stimulated, cause the pupil to _________
2. After compression of CrN III, what happens to the pupillary size? Explain.
_________
_________
3. Because the pupilloconstrictor fibers occupy the superomedial part of CrN III, the caudally displaced posterior cerebral artery impinges on them first when the temporal lobe herniates (Fig. 12-11).
FIGURE 12-11. Relation of pupilloconstrictor fibers of cranial nerve III to the posterior cerebral artery. To orient this illustration, see Figs. 12-6 and 12-8.
4. In a Pt suspected of having a space-occupying intracranial lesion, what would an increasing pupillary size and a decreasing level of consciousness imply?
_________
_________
5. As transtentorial herniation advances, both IIIrd nerves may cease to function. Both pupils become dilated and fixed, no longer responding to light. Before dilating, the opposite pupil may go through a phase of mild constriction (Forbes et al, 1965; Ropper, 1990). Whereas a unilateral dilated and fixed pupil announces danger requiring prompt or even heroic intervention, bilateral dilated and fixed pupils from brainstem compression are almost synonymous with death without prompt neurosurgical intervention (Clusmann et al, 2001).
H. Effect of transtentorial herniation on motor function
1. Consider a Pt with a large acute right hemisphere lesion. It would cause a left-sided/
right-sided hemiplegia (Video 12-1). (
left-sided)
Video 12-1. Recovery from right malignant middle cerebral artery (MCA) stroke; status posthemicraniectomy.
2. As the herniating right hemisphere compresses the mesencephalon, the left basis gets pushed against the opposite free edge of the tentorium on the left side, indenting the basis (Kernohan–Woltman notch). Notice in Fig. 12-7 the close relation of the basis mesencephali to the tentorial edges, affording virtually no safety factor.
3. After compression of the left basis, the UMN fibers cannot transmit impulses. The Pt now shows a _________ ipsilateral/
contralateral to the right hemisphere lesion. (right;
ipsilateral)
4. Such an ipsilateral hemiplegia is called a paradoxical hemiplegia because it is on the same/
opposite side as the hemispheric lesion. Sometimes the paradoxical hemiplegia appears first, constituting a false localizing sign of the side of brain herniation. (
same)
5. Hence, if a Pt started out with a large right hemispheric lesion and left hemiplegia and then had a right hemiplegia, the Pt has double hemiplegia. If the double hemiplegia evolves very rapidly, the Pt might display cerebral shock. What would happen to the MSRs and tone? _________
6. Thus, depending on the rapidity of evolution of the lesion, the MSRs and tone may change from time to time and from side to side.
7. Recite the neurologic findings in transtentorial herniation with respect to the IIIrd nerve, the ARAS, and the pyramidal tracts in the basic mesencephali. Check the preceding frames to be sure that you have recited the effects correctly.
8. The Kernohan-Woltman notch/paradoxical hemiplegia sequence can be regarded as a contrecoup condition because it is on the opposite side of the inciting hemispheric lesion; but in acute head injury a coup type of displacement may similarly nick the midbrain ipsilaterally (Saeki et al, 2000).
I. Bilateral transtentorial herniation
1. Many pathologic conditions cause bilateral transtentorial herniation. The lesion may consist of cerebral edema from trauma, encephalitis, or metabolic disorders such as uremia and hepatic coma. Of the structural lesions, head injuries, subdural hematomas, hydrocephalus, multiple metastatic neoplasms or abscesses, and intracranial hemorrhage lead the list. If both hemispheres swell, the unci and parahippocampal gyri of both sides try to squeeze down through the tentorial notch. The ring of swollen tissue acts exactly like a ligature around the midbrain.
2. The coup de grace to the Pt with transtentorial herniation, bilateral or unilateral, is mesencephalic and pontine hemorrhages secondary to the herniation (Friede and Roessmann, 1966; Hassler, 1967). The stretching and compression of the brainstem vessels stops the blood flow. The vessel walls rupture. The consequent brainstem hemorrhage is usually the terminal event that causes the Pt to die (Fig. 12-12).
FIGURE 12-12. Transverse section of the mesencephalon showing hemorrhages secondary to transtentorial herniation. The IIIrd nerves are seen exiting ventrally. The caudal displacement of the brainstem by the herniating cerebrum stretches and compresses the brainstem vessels until they rupture, killing the patient.
J. Decerebrate rigidity, a postural syndrome of midbrain lesions
1. Description of the decerebrate posture: Extensive midbrain lesions, intrinsic or from unilateral or bilateral transtentorial herniation, disconnect the cerebrum and diencephalon from the rest of the brainstem, that is, decerebrate the individual. The “decerebration” can, in fact, be toxic–metabolic rather than anatomic. Along with the IIIrd nerve signs, double hemiplegia, and loss of consciousness, the Pt may show a diagnostic postural syndrome called decerebrate rigidity (gamma rigidity). Study Fig. 12-13 and assume the decerebrate posture yourself. Can you maintain it easily?
FIGURE 12-13. Decerebrate posture in an unconscious Pt. (A) Patient placed on his side. (B) Patient placed with his head over the edge of the table to show the rigid extension of his neck. (Adapted with permission from Penfield W, Jasper H. Epilepsy and the Functional Anatomy of the Human Brain. Boston, MA: Little, Brown & Co.; 1954).
2. After carefully inspecting the head, jaw, trunk, and limb postures of the Pt, cover Fig. 12-13 and test your powers of observation by completing Table 12-3.
TABLE 12-3 • Posture in Decerebrate Rigidity
The mouth is |
The head is |
The trunk is |
The arms are |
The wrists are |
The fingers are |
The legs are |
The feet are |
The toes are |
3. Muscle tone in decerebrate rigidity: Testing of the muscle tone of the Pt shown in Fig. 12-13 would disclose rigid extension of the extremities and spine, but if you once bend the part, it will yield like a clasp knife. Hence, the tonic disturbance seems to combine features of spasticity and rigidity.
4. Descriptive definition of decerebrate rigidity: The Pt displays an involuntary posture with the:
a. Proximal joints (spine, shoulders, hips, elbows, and knees) rigidly extended/
flexed. (
extended)
b. Distal joints (wrists/ankles, fingers/toes) rigidly extended/
flexed. (
flexed)
c. Forearms and legs internally/
externally rotated. (
internally)
5. Pathophysiologic explanation of the decerebrate posture: As a prelude to interpret the decerebrate posture, review the strength of the muscle actions listed in Table 12-4. Actually test a normal subject to complete the table.
TABLE 12-4 • Comparative Strength of Postural Deviation in Decerebrate Rigidity
Action | Stronger than | Weaker than | Opposing action |
Jaw closure is | Jaw opening | ||
Head extension is | Head flexion | ||
Trunk extension is | Trunk flexion | ||
Arm extension is | Arm flexion | ||
Forearm pronation, arm extended is | Supination | ||
Wrist flexion is | Wrist extension | ||
Finger flexion is | Finger extension | ||
Leg extension is | Leg flexion | ||
Inversion of foot is | Eversion | ||
Ankle flexion is | Ankle extension | ||
Toe flexion is | Toe extension |
a. After completing Table 12-4, you can conclude that the direction of pull of the strongest muscles determines the posture of the spine and extremities assumed by the decerebrate Pt.
b. Figure 12-14 shows the posture of a quadruped with decerebrate rigidity. A quadruped with decerebrate rigidity will stand, if set on its feet. Review also Fig. 7-2.
c. The extended head, tail, and extremities and the closed jaw of the quadruped indicate that the muscles that acting to cause the decerebrate posture support a quadruped animal’s posture against collapse due to the pull of gravity. These same muscles cause it to leap and locomote in defiance of gravity. Hence, we interpret decerebrate rigidity as that posture maintained by excessive contraction of the antigravity muscles of the quadruped. In general, the strongest muscles of humans are the true antigravity muscles of a quadruped/
biped. (
quadruped (Review Table 12-3 and Fig. 7-2, if you missed.))
d. Describe how you could produce the decerebrate posture in an experimental animal._________
FIGURE 12-14. Decerebrate posture in the cat. (Reproduced with permission from Pollock L, Davis L. The reflex activities of a decerebrate animal. J Comp Neurol. 1930;50:377–411.)
6. Role of the vestibular system in decerebrate rigidity: After decerebration by midbrain transection interrupts the impulses descending from the cerebrum, the vestibular system becomes overactive, causing it to overstimulate or drive the powerful antigravity muscles. (If you tested carefully, you should have checked the stronger than column every time.)
a. Explain whether you should classify the decerebrate posture as a deficit or a release phenomenon._________
b. Describe a surgical experiment or series of experiments to prove that the drive for the decerebrate posture comes from the vestibular system. Think carefully.
_________
_________
c. Like any release phenomenon, decerebrate rigidity not only requires a lesion to release it but also an intact neural mechanism to produce, drive, and perpetuate it. The term “decerebrate rigidity” was first used by Sherrington (1898) after an intercollicular transection (between the red nuclei and the vestibular nuclei) in animal studies. Sherrington showed that destruction of the vestibular system abolishes the decerebrate posture. Apparently the activity that drives the decerebrate posture arises in the vestibular system and is conveyed to the spinal cord by the vestibulospinal tracts. Dorsal or ventral root transection also abolishes it.
d. For decerebrate rigidity to appear, the critical neural structure transected or compressed is the _________
7. Give a succinct clinical description of the decerebrate posture. Be sure to separate observation from interpretation._________
_________
8. Give the pathophysiologic interpretation of decerebrate rigidity.
_________
_________
9. Some unconscious Pts show decerebrate posturing only in response to pain. To elicit the posture, press the ball of your thumb or a knuckle hard for several seconds against the Pt’s sternum.
10. Despite the relatively poor prognosis, rarely Pts with decerebrate rigidity remain conscious (Halsey and Downie, 1966) and rarely recover (Conomy and Swash, 1968; Brendler and Selverstone, 1970; Damasceno, 1991; Kao et al, 2006).
K. Decorticate versus decerebrate posture
1. The decerebrate posture differs from chronic hemiplegia (after the state of cerebral shock or diaschisis in acute hemiplegia has passed). Compare Figs. 12-13 and 12-15.
FIGURE 12-15. Posture in an adult with chronic hemiplegia. Compare the arm position with that shown in Fig. 12-13.
2. Describe the major difference in the posture of the arm in decerebrate rigidity versus hemiplegia.
_________
_________
L. Respiratory and autonomic effects of brainstem lesions and transtentorial herniation
1. The unconscious Pt may display strong inspiratory stridor because of collapse of the oropharyngeal muscles. Simply place your fingers behind the ramus of the mandible and pull the mandible forward. The Pt will immediately breathe more freely. Positioning the head to the side or placing an oropharyngeal airway then solves the problem.
2. Lesions at various levels from the cerebrum to the upper cervical cord cause characteristic respiratory dysrhythmias. Figure 12-16 depicts some of the dysrhythmias, but it depicts their localizations more schematically than the data warrant.
FIGURE 12-16. Correlation of intra-axial brainstem lesions at successive levels, with the type of respiratory dysrhythmia caused. (A) Cheyne–Stokes respiration. (B) Central neurogenic hyperventilation. (C) Apneustic breathing. (D) Cluster breathing. (E) Ataxic breathing. (Redrawn from Plum F, Posner J. The Diagnosis of Stupor and Coma. 3rd ed. Philadelphia, PA: FA Davis; 1980.)
3. Transtentorial herniation, by external compression, caudal displacement, and kinking of the brainstem, causes a “wave of failure” to pass caudally from diencephalon to medulla. The Pt passes in succession through the various respiratory dysrhythmias shown in Fig. 12-16 that reflect the effects of lesion at various rostrocaudal levels. One type of dysrhythmia seen with intrinsic brainstem lesions but not with transtentorial herniation is apneustic breathing (Fig. 12-16C; Plum and Posner, 1980).
4. Among many other dysrhythmias not specific as to lesion site or cause are Biot and Kussmaul.
a. Kussmaul breathing is characterized by “air hunger,” with deep regular sighing respiration (increase tidal volume), whether the rate is slow, normal, or fast. Kussmaul respiration suggests the presence of metabolic acidosis, and is the result of stimulation of the brainstem respiratory centers by the low blood pH. It occurs with metabolic disorders, such as diabetic ketoacidosis and uremia, exogenous acids (eg, intoxication with salicylate methanol, or ethylene glycol), and other severe systemic illnesses.
b. Biot breathing resembles Cheyne–Stokes breathing (Fig. 12-16A) by consisting of periods of apnea that alternate irregularly with series of breaths, but the four to five breaths have equal length and terminate abruptly rather than fading in and out.
5. The effect of transtentorial herniation or other brainstem lesions on blood pressure and pulse varies. Although hypertension or hypotension and tachycardia or bradycardia may occur, typically increased ICP causes a slowing of the pulse rate and an increasing blood pressure (Cushing phenomenon). Many factors, such as the ventilatory efficiency, may alter this formula. Thus, the Ex must expect changes in the vital signs and monitor them carefully.
M. Transforaminal herniation
1. Anatomy of transforaminal herniation: The diffusely swollen, herniating brain may impose the death penalty by a mechanism other than transtentorial herniation. As increased pressure pushes the intracranial contents caudally, the cerebellum and medulla herniate into the foramen magnum. This aperture is designed to accommodate only the cervicomedullary junction.
a. Because the caudalmost part of the cerebellum, the cerebellar tonsils, is the part of the cerebellum to herniate, this lesion is called tonsillar or transforaminal herniation. See the herniated right cerebellar tonsil shown in Fig. 12-8.
b. Transforaminal herniation may result from expanding supra tentorial lesions or from expanding infratentorial lesions, such as a cerebellar hemorrhage or neoplasm. Chapter 13 describes the danger of transforaminal herniation after lumbar puncture (LP).
c. Infratentorial masses also may cause upward transtentorial herniation, causing midbrain compression and midbrain signs (Cuneo et al, 1979).
2. The clinical syndrome of transforaminal herniation: The clinical syndrome mimics transection of the neuraxis at the medullocervical junction level. The Pt becomes quadriplegic and totally apneic (Table 12-5). The total apnea results from the quadriplegia that stops volitional breathing and compression of the reticulospinal tracts that stops automatic breathing. No respiratory drive reaches the lower motoneurons (LMNs). Review Fig. 6-17.
TABLE 12-5 • Summary of Vascular Complications and Clinical Signs of Internal Herniations of the Brain
Type of herniation | Vascular complications | Clinical signs |
Subfalcine herniation medially of the cingulate gyrus | Compression of anterior cerebral artery under free edge of the falx, causing infarction of medial hemispheric wall and motor area for the leg (Figs. 2-2C and 12-9) | Obtundation, disorientation, monoparesis of contralateral lower extremity |
Transtentorial herniation downward and medially of the uncus and parahippocampal gyrus | Compression of posterior cerebral artery against free edge of tentorium (Figs. 12-6 and 12-7); brainstem hemorrhages (Fig. 12-12). The anterior choroidal and posterior communicating arteries may be displaced inferomedially in severe cases | Increasing loss of consciousness, pupillodilation; and IIIrd nerve palsy; hemianopia |
Transtentorial herniation upward of the cerebellar vermis and midbrain | Compression of superior cerebellar artery by free edge of tentorium and compression of midbrain and brainstem vessels | Increasing obtundation, pretectal/rostral midbrain syndrome (Table 5-2), IIIrd nerve palsy |
Transforaminal herniation downward of the cerebellar tonsils and medulla oblongata | Infarction of the cervicomedullary junction and rostral 1-2 segments of spinal cord from compression of ventral and posterior spinal arteries that arise inside the foramen magnum from the vertebral and posterior inferior cerebellar arteries; hemorrhagic necrosis of the cerebellar tonsils | Neck stiffness or head tilt. Apnea and quadriparesis from compression of the medullocervical junction |
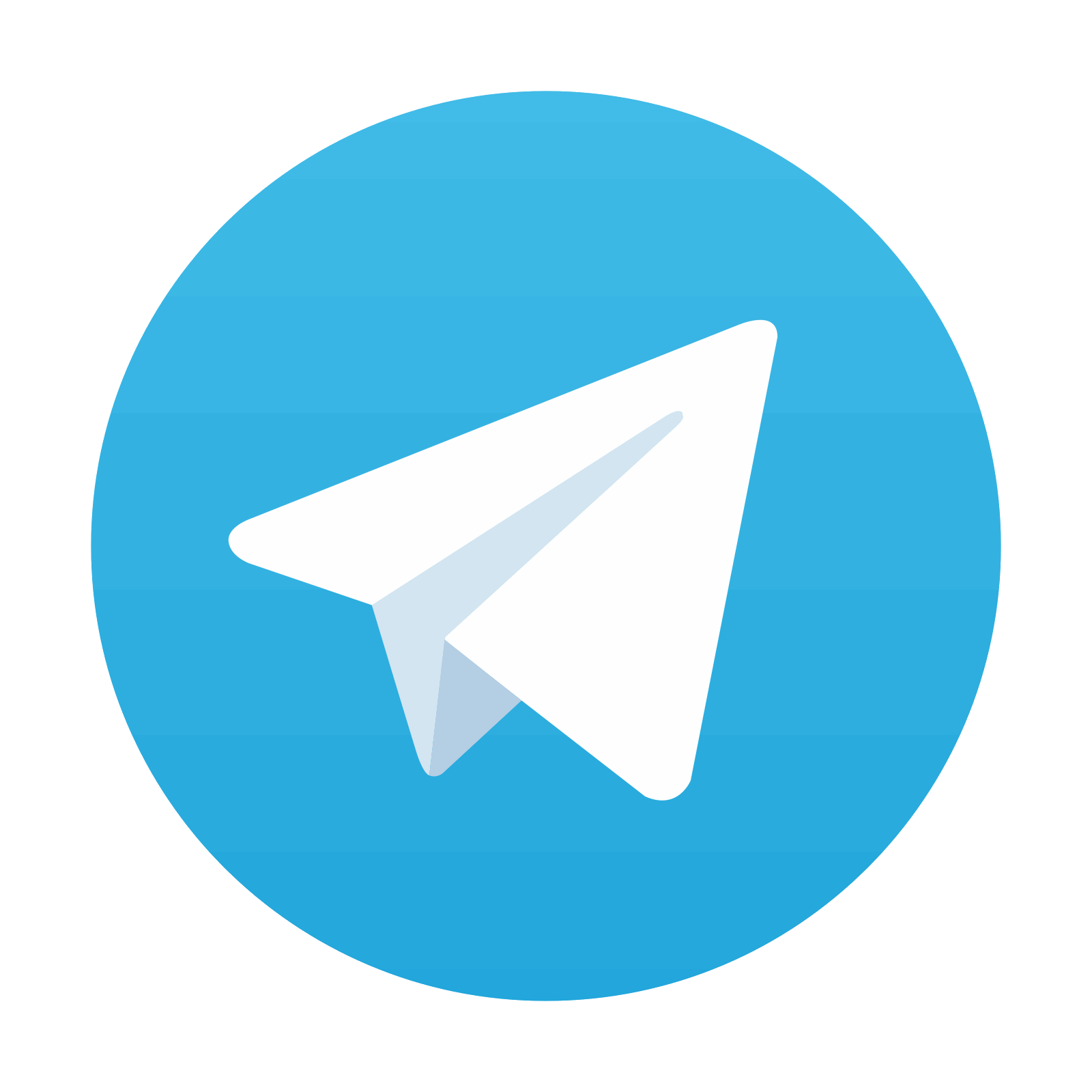
Stay updated, free articles. Join our Telegram channel

Full access? Get Clinical Tree
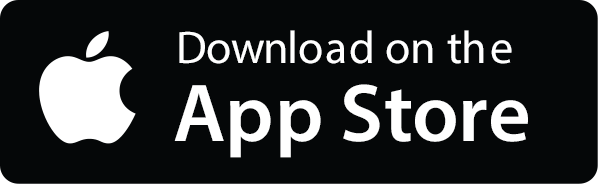
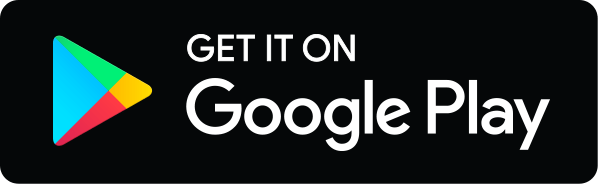