Demands from health insurance organizations and hospitals to remain competitive in the health care market, as well as the impact of cost, patient satisfaction, and a quicker return to normal daily function, have pressured physicians and biotechnology manufacturers to address the needs of an evolving work-oriented society. Stemming from the initial experiences of successful early ambulation following surgery and the advantages of outpatient surgery to the patient, hospital, and physician, a trend toward more minimally invasive procedures ensued.1 Thus, to reduce operative time and complications, minimize intraoperative tissue trauma, diminish the length of hospital stays, lessen postoperative use of narcotics, and enhance postoperative outcomes, minimally invasive techniques have been developed to address morbidities associated with more open surgical procedures of the spine. The earliest indication of spine pathology was found in Egyptian mummies dated 2900 BC and further elucidated in the Edwin Smith papyrus written circa 1550 BC. In fact, the first description of the spinal column was offered by the Egyptians in the djet symbol used for the god Osiris to represent stability, duration, and durability.2 Emerging from the age of antiquity, Hippocrates has been credited as the father of spine surgery, as evidenced by his seminal teachings and writings, underlined by his principles of sound reasoning and accurate observation3; however, the first concrete delineation of operative treatment of the spinal column was proposed by Paulus of Aegina in the seventh century.4 He advocated and conducted direct removal of osseous tissue at the site of pathology. Since then, various approaches and techniques for operative treatment of the spine have been developed and evolved to address numerous conditions. In this chapter, we will review the evolution of minimally invasive techniques in the spine. Cervical Spine Endoscopic-Assisted Transoral Surgery Fang and Ong5 published the first series of patients to undergo transoral decompression for irreducible atlantoaxial abnormalities in 1962; however, undue morbidity and mortality caused poor acceptance of this approach. Advancements in imaging techniques and the availability of the operative microscope improved the safety and efficacy of the approach. This approach, though, requires such wide exposures as soft/hard palate splitting and extended maxillotomities for decompressing clival abnormalities, resulting in increased operating time, complexity, prolonged recovery, and patient morbidity.6 Frempong-Boadu et al6 demonstrated the efficacy of endoscopic-assisted transoral surgery in limiting these extended approaches in a series of seven patients. One of the disadvantages of using the microscope is that the optics are located at a distance from the location of the abnormality. With the endoscope, the optics and illumination are in the field of the abnormality. With the use of the 30-degree angled endoscope, one can clearly visualize the clivus abnormality, obviating the need for extensive palatal splitting.6 FIGURE 1–1 The microendoscopic diskectomy system comprising tubular dilators and retractor system can be used for cervical as well as lumbar surgeries. Cervical Microendoscopic Laminectomy/Diskectomy Scoville and Whitcomb7 introduced the concept of posterior cervical disk surgery. Although the anterior approach to the cervical spine has become more popular, the posterior approach is an effective procedure in selected cases. It is indicated in cases with laterally herniated disk fragments, isolated foraminal narrowing, multilevel foraminal narrowing without central stenosis, continued root symptoms after anterior cervical diskectomy and fusion, and patients for whom anterior approaches are contraindicated; however, it is usually necessary to use moderate-size incisions and to perform significant paraspinous muscle dissection in these operations. Postoperative wound pain and muscle spasms can therefore be significant, and postoperative recovery is often relatively slow. The microendoscopic diskectomy (MED) system was developed to minimize the tissue trauma and postoperative discomfort seen with open procedures (Fig. 1–1). It enables posterior cervical and lumbar diskectomy through a tubular retractor, with endoscopic observation. A guidewire is inserted through the posterior cervical musculature, with the tip being fluoroscopically directed to the operative disk space. Dilators are sequentially inserted through the posterior neck musculature, at the junction of the lamina and the lateral mass (Fig. 1–2). A 16 mm tubular retractor is then inserted over the largest dilator and fixed to the flexible arm assembly on the table. The endoscope is fixed inside the tubular retractor. The tubular retractor tip is positioned to expose the lateral portion of the superior and inferior laminae and the medial portion of the corresponding facet joint. After exposure has been achieved, a small curved curette is used to define the edge of the superior lamina and the lateral mass. Bone removal begins on the inferolateral portion of the superior lamina and proceeds to the superolateral portion of the inferior lamina. After palpation of the medial edge of the superior and inferior pedicles with a microprobe, bone removal is extended into the medial facet joint to expose the nerve root. Bone removal is performed with a small Kerrison punch or a high-speed drill as in open procedures. The MED system provides equally good exposure of the involved segment and decompression compared with open microdiskectomy. FIGURE 1–2 Intraoperative lateral fluoroscopic scan confirming the position of the working channel of the microendoscopic diskectomy system for a C4–C5 foraminotomy and diskectomy. In cadaveric studies, Roh et al8 performed posterior cervical foraminotomies using either the MED system or conventional open techniques. Their results demonstrated that the average vertical diameter of decompression and percentage of facet removed were greater for the MED technique. The transverse diameter of the laminotomy area and the average length of decompressed root were not significantly different. Transmuscular dilation with an endoscopic tubular working channel can minimize postsurgical pain and provide rapid postoperative recovery. Thoracic Spine Thoracoscopic Spine Surgery Jacobaeus, a professor of internal medicine in Stockholm, Sweden, is credited with performing the first thoracoscopic procedure in 1910.9 As an internist, he was involved in the diagnosis and treatment of pulmonary tuberculosis, and he thought that the ability to observe the pleural space was crucial. After his initial diagnostic procedures, Jacobaeus described the technique of lysis of tuberculous pleural adhesions, which was performed with a cystoscope and a heated platinum loop.10 In 1990, with the introduction of video imaging to standard endoscopy, the modern era of thoracoscopy began. Mack et al11 in the United States and Rosenthal et al12 in Europe first reported the technique of video-assisted thoracic surgery (VATS) in 1993. Thoracoscopic spine procedures were initially implemented to treat disk herniations, pathologies of the vertebral body, and drainage of abscesses, as well as for tumor biopsies. In the ensuing years it was used in cases of scoliosis, anterior interbody fusions, osteotomies and bone grafting, corpectomies, and vertebral instrumentation for tumors and fractures. Thoracoscopic access is achieved by temporarily deflating the lung on the ipsilateral side of the spinal exposure using a double-lumen endotracheal tube. Narrow portals are introduced through small incisions in the intercostal spaces. The endoscope is then introduced through one of the portals, with the other portals serving as working channels and being used interchangeably. Thoracoscopy can be used to access the entire thoracic spine and thoracolumbar junction (to L2), providing visualization of the disks, vertebral bodies, and ipsilateral pedicle (Fig. 1–3). It offers several advantages over conventional open thoracotomy to treat spinal disorders. VATS allows visualization of the procedure to the entire operating team, and two surgeons may work side by side. VATS uses small incisions and a minimal amount of rib resection, and there is no spreading of the ribs, thus minimizing postoperative discomfort, reducing incisional pain, creating fewer respiratory and shoulder girdle problems, and decreasing blood loss. It has also shortened hospitalization time and length of rehabilitation after surgery. Like most endoscopic techniques, the successful application of VATS has a steep learning curve that requires the operator to acquire the technical skills needed to use the long working arms of the endoscopic equipment. Bony dissection requires stable and precise manipulation of standard open equipment with modified longer working arms. Thus, it is crucial to use both hands to stabilize and achieve precise control over the drill, curettes, or bone rongeurs used to avoid devastating neural or vascular injuries. Thoracoscopic sympathectomy has also been used with success for treatment of anhydrosis in an ambulatory setting. Furthermore, thoracoscopic sympathectomies have been performed with multiportal and biportal systems; even uniportal approaches are now being used. FIGURE 1–3 Thoracoscopic surgery. The image illustrates thoracoscopic techniques to obtain thoracolumbar decompression and stabilization for a severe TIZ burst fracture. Posterior/Posterolateral Endoscopic Diskectomy In a further attempt to reduce tissue trauma and enhance postoperative outcome, a thoracic microendoscopic diskectomy instrumentation and approach have been developed and employed with preliminary success to treat thoracic disk herniations from a direct posterior or posterolateral endoscopic approach. Jho13 described the technique of endoscopic transpedicular thoracic diskectomy with a 0- and 70-degree 4 mm endoscope requiring relatively small 1.5 to 2 mm incisions and minimal tissue dissection. This approach avoids the need for separate skin incisions in the chest wall (required for thoracoscopic approaches) and the need for postoperative chest drainage. Chiu and Clifford14 demonstrated the safety and efficacy of posterolateral endoscopic thoracic diskectomy followed by low-energy nonablative laser for shrinkage and tightening of the disk (laser thermodiskoplasty) using a 4 mm 0-degree endoscope. Lumbar Spine Chemonucleolysis In 1941, Eugene Jansen and Arnold Balls15 first isolated chymopapain, a proteolytic enzyme extracted from papaya latex. In 1956, Lewis Thomas16 administered intravenous injections of crude papain to rabbits and noticed that their ears drooped. Intrigued by Thomas’s article, Lyman Smith postulated the therapeutic use of papain to treat chondroblastic tumors. In 1963, Smith et al17 were the first to inject chymopapain into a herniated nucleus pulposus for the treatment of sciatica. This process, aptly called chemonucleolysis, alters the characteristics of the nucleus pulposus by liberation of chondroitin sulfate and keratin sulfate by hydrolysis of noncollagenous proteins of mucopolysaccharide involvement and leading to polymerization of the nucleus pulposus. It has been used safely to treat disk disease in the spine for the past three decades. Despite the extensive use of chymopapain, chemonucleolysis still provokes controversy. After more than 30 years of clinical experience, research, double-blind trials, and Food and Drug Administration (FDA) approval, differing views persist. Nordby and Javid18 published the results of a 14-year study of 3000 patients, with an 82% success rate for the first 1000 patients and an overall success rate of 87.2%; however, others have questioned the safety and indications for the use of chymopapain to treat disk disease. Some 75 investigators in the United States and Canada administered injections to nearly 17,000 patients in phase III chemonucleolysis trials, which ended in July 1975. The results of the phase III trials were mixed, with several enthusiastic reports and several others that questioned the safety and efficacy of chymopapain.19,20 To date there is no consensus in the neurosurgical and orthopedic communities regarding the use of chymopapain. Proper patient selection is crucial for any intervention, and this is especially true for percutaneous chemonucleolysis. Chemonucleolysis should be reserved for patients presenting with radicular symptoms, with radiological studies [magnetic resonance imaging (MRI), computed tomographic (CT), and/or myelographic scans] confirming a herniated soft disk, and who have experienced failure of conservative nonsurgical treatment. The size and shape of the disk are not factors for safe chemonucleolysis. The proponents of chymopapain claim that lack of familiarity, training, and indications could explain some of the complications. Age can be a factor because patients over 60 years of age may lack sufficient mucoprotein for hydrolysis of the herniated disk. For patients younger than 20 years of age, 80 to 90% success rates have been reported.21 FIGURE 1–4 Diagram showing anatomic features of the relevant structures close to the path of the needle. Chemonucleolysis is performed with the patient placed in either a lateral or a prone position; the needle is positioned in the center of the offending disk, with fluoroscopic guidance (Fig. 1–4). The currently recommended dose is 1000 to 2000 units/disk space. With chymopapain sensitivity testing and administration of antihistamine agents before chymopapain injection, anaphylaxis has been reduced to 0.25% of total allergic reactions. The vast majority (87%) of the reported complications occurred before 1984, and no major anaphylactic complications have been reported in the United States since July 1987.22 There is significant cost effectiveness in the use of chemonucleolysis for the treatment of intervertebral disk disease. As noted by Ramirez and David,23 the cost of chemonucleolysis is, on average, 23% lower than that of surgical treatment of disk herniation. Percutaneous Diskectomy In 1975, Hijikata et al24 demonstrated a percutaneous nucleotomy by utilizing arthroscopic techniques for disk removal via intradiscal access for the treatment of posterior or posterolateral lumbar disk herniations under local anesthesia. After diskography using Evans blue dye, specially designed instruments were placed in a 5 mm cannula and inserted against the lateral anulus. A circular incision was made in the anulus, and the blue-stained nucleus pulposus was removed with pituitary forceps. In 1983, Kambin and Gellman25 performed a diskectomy by inserting a Craig cannula and a small forceps into the disk space after an open laminectomy to evacuate the nucleus pulposus. In 1985, Onik et al26 reported the development of a 2 mm blunt-tipped suction cutting probe for automated percutaneous diskectomy at L4–L5 or higher levels. The selection criteria for patients undergoing percutaneous diskectomy are similar to those for patients undergoing chymopapain treatment. Patients are selected on the basis of clinical symptoms and radiological evidence that removal of the nucleus pulposus would decompress the affected nerve root. It is also important to identify the presence or absence of extruded fragments. On T2-weighted MRI scans, extruded disks appear brighter than contained disks, which exhibit dark coloration similar to that of degenerated disks. CT diskograms can demonstrate the presence or absence of extruded disk fragments. The size of the protrusion is an important factor in obtaining successful outcomes with percutaneous diskectomy. If the disk herniation is >50% of the anteroposterior diameter of the thecal sac, then there is a >90% chance that the patient will experience a poor outcome after percutaneous diskectomy. Patients with narrowed disk spaces are also poor candidates for percutaneous diskectomy. Kambin and Sampson27 initiated the use of fluoroscopy before the procedure for confirmation of the appropriate disk level. The anteroposterior view is used to confirm that the patient is in line without rotation. The lateral view should indicate that the end plates are parallel. Preoperative CT or MRI scans of the entire abdomen through the involved disk space should be obtained to avoid damaging crucial structures in a far lateral approach. Suction diskectomy is performed with the patient in either a prone or a lateral position. Flexion of the hips reduces lumbar lordosis and allows for a larger entry zone posteriorly. The procedure is performed using mild sedation and local anesthesia. An 18-gauge, 25 cm, hubless, stainless steel trocar is inserted and advanced obliquely, with fluoroscopic guidance, toward the posterior margin of the intervertebral disk to reach the appropriate level. The starting point on the skin is usually 10 cm from the midline. When the trocar is in the appropriate position, a 2.8 mm cannula with an internal, blunt-end, tapered dilator is placed over the hubless trocar. When the cannula and dilator are in the correct position, the dilator is removed. The cannula is pressed against the anulus, and a 2 mm, open-ended, circular cutting tool is inserted through the cannula to cut a hole in the anulus. The nucleotome is advanced into the disk space. The nucleotome is 20 cm in length, with a rounded tip. The reciprocating action of the cutting tool and aspiration occur simultaneously. Saline solution is delivered to the disk space by flowing between the inner and outer cannulas, producing a suspension of macerated disk material as the inner cannula reciprocates at 180 cycles/min, permitting rapid cutting. The slurry of saline solution and disk material is aspirated through the inner cannula into a collection bottle. After the disk space has been accessed, the diskectomy can be completed in 15 to 20 min. FIGURE 1–5 Result of using an automated percutaneous lumbar diskectomy system for a single-level disk herniation. Minimally invasive lumbar surgery can be performed successfully using percutaneous techniques. The outcomes of more than 4500 automated or manual percutaneous lumbar diskectomies (PLDs) have been reported. The reported outcomes indicate an overall success rate of 75%, with a complication rate of 1%. Automated PLD is appropriate for patients with single-level disk disease (Fig. 1–5). It is not considered an option for patients with a history of previous chymopapain or surgical treatment for disk disease, progressive neurologic deficits, bowel or bladder problems, sequestered disk fragments, or evidence of vertebral disease (e.g., spinal stenosis or spondylolisthesis). The role of PLD remains investigational until prospective, randomized, controlled studies reliably validate outcomes. In the future, spinal endoscopy may facilitate observation of the area of nucleotomy and increase the amount of disk removal. Percutaneous Laser Diskectomy Ascher and Heppner28 were the first to use the technique of percutaneous laser diskectomy to treat lumbar disk disease. Their technique involved measuring the intradiscal pressure before and after laser diskectomy, using a saline manometer. They postulated that the removal of even a small volume of tissue from the disk caused a corresponding decrease in intradiscal pressure.28 This procedure is not indicated for patients with uncontained disk extrusions or disk fragments outside the disk space. The combined results of Ascher,29 Choy et al,30 and others demonstrated 70 to 80% rates of longlasting pain relief for more than 1000 patients. In 1990, Yonezawa et al31 used a neodymium:yttrium-aluminumgarnet (Nd:YAG) laser through a double-lumen needle with a fiberoptic, quartz-fiber tip to measure pressure before and after the procedure. Percutaneous laser diskectomy is an outpatient procedure that requires percutaneous placement of a single needle in the disk space. With fluoroscopic verification of the level and placement of the needle and with coupling via a fiber, laser energy is passed into the disk space. The laser energy is transmitted in short bursts to avoid excessive heating of the adjacent tissue. The consensus is that percutaneous laser diskectomy should demonstrate indications and successes similar to those of other percutaneous modalities. In 1992, Davis32 used a potassium-titanal-phosphate (KTP) laser for lumbar disk ablation, achieving a success rate of 80%. Producing a light that is lime green at 531 nm, the KTP laser can be coupled to a fiberoptic cable and can be easily directed into the intervertebral disk space through a spinal needle. The procedure is appropriate for patients with contained disk herniations associated with radicular symptoms. The KTP laser has also been approved for percutaneous laser diskectomy. Advances have allowed the development of side-firing probes, which provide better directional control and allow better observation. The side-firing laser probe reduces the risk of injury to such anterior structures as the vena cava, aorta, and iliac vessels. Yeung33 recommended injecting the disks with indocyanine green (to act as a chromophore), thus maximizing delivery and minimizing the chance of injury to adjacent structures. The holmium:YAG laser has a 2.1 μm wavelength (in the midinfrared region), which can easily be delivered to the intervertebral disk space. In contrast to the continuous-wave lasers of other systems, the holmium: YAG system has a unique pulsed laser, which enables adjustment of the pulse width and frequency, to reduce damage to adjacent tissue. In bench testing with a pulse width of 250 msec at 10 Hz and 1.6 J/pulse, no temperature increase was noted in adjacent tissues. The disk cavitation caused by the laser results in a reduction in intradiscal pressure, decreasing the force driving the herniated fragments posteriorly. The only reported complication was a case of diskitis in a series of 333 procedures described by Choy et al.34 The possible complications theoretically include perforation of the aorta, vena cava, iliac vessels, or abdominal contents and cauda equina syndrome. The results of percutaneous laser disk decompression in cases involving back and leg pain with disk protrusions are still unclear. No controlled prospective studies have been performed to evaluate the results of percutaneous laser diskectomy. The largest experience in the literature was reported by Choy et al.34 They found excellent results in 87.4% in a study of 333 patients, with a mean follow-up of 26 months. Early experience with the KTP/532 device was reported by Davis,35 who achieved an 85% success rate. Yeung33 reported good to excellent results in 84% with the KTP/532 device. Percutaneous laser diskectomy is one step in the development of safer techniques for disk removal. Various laser wavelengths have been used for this purpose, but no consensus exists regarding success with this form of treatment. Published results demonstrated 70 to 80% success rates for selected groups; unfortunately, laser diskectomy as a stand-alone procedure adds controversy to the field of percutaneous treatment of lumbar disk disease. Current techniques, however, involve manual removal of disk material with laser modulation under endoscopic control. The use of lasers under direct observation can be performed safely and effectively. Arthoscopic Microdiskectomy Ottolenghi and Argentina36 in 1955 and Craig37 in 1956 described posterolateral biopsy of the spine. In 1983 and 1986, Kambin25,27 and co-workers combined open laminectomy with nucleotomy, via Craig instrumentation, to observe the effects of nucleus pulposus removal on the surrounding anatomic features. In 1975, Hijikata et al24 developed mechanical tools for nucleotomy using similar principles. Refinements to the technique involved the use of an automated system. Subsequent developments led to the design of a 2.7 mm glass arthroscope combined with a videodiscoscope with a single working portal.38 This development enabled observation of periannular structures, including the foramina and the spinal nerve. Arthroscopic disk surgery allows removal of herniated disks via a posterolateral approach. This is accomplished with biportal access via triangulation into the intervertebral disk, with adequate irrigation and suction.38 The mechanism of pain relief after arthroscopic microdiskectomy and central nucleotomy is believed to involve reduced intradiscal pressure, removal of inflammatory agents, reductions in intervertebral disk height, and lower tension on the nerve. The dramatic cessation of radicular pain after extraction of a herniated nuclear fragment in the course of an open surgical procedure proves that removing tension and irritation from the nerve root helps provide pain relief. Small rigid arthroscopes allow inspection of the anulus, spinal nerve, and foramina. Intradiscal arthroscopic observation is not required for simple nucleotomy or disk debulking. Localization and removal of posteriorly herniated disk fragments require good arthroscopic illumination and observation. There are currently 0-, 30-, and 70-degree angled arthroscopes and flexible arthroscopes are available. Patients with herniated lumbar disks who are selected for arthroscopic microdiskectomies should demonstrate symptoms of nerve root irritation and present correlative radiological evidence. All intra-annular, subligamentous, and extraligamentous herniations are accessible with arthroscopic microdiskectomy; however, extraligamentous disk fragments that have migrated in caudal or cephalad directions cannot safely be removed using arthroscopic microdiskectomy. The triangular working zone has been defined as a safe zone in the posterolateral anulus, allowing safe passage of instruments with minimal risk to the exiting nerve.25,27 As the nerve exits the foramen, it extends laterally and distally, forming the anterior borders of the triangular working zone. The inferior border of this safe zone is the vertebral end plate of the lower body. It is bordered posteriorly by the articular process and superior articulating facet of the lower vertebrae and medially by the traversing root and dura (Fig. 1–6). FIGURE 1–6 Red shaded area represents the triangular working zone (Kambin’s triangle) defined as the “safe zone” at the posterolateral anulus, allowing safe passage of endoscopic instruments to the disk space with minimal risk to the exiting nerve roots. Uniportal access is achieved with a 5 mm internal diameter cannula, which provides access for central and posterior extraction of herniated intra-annular fragments. The instruments required for arthroscopic microdiskectomy include 18-gauge needles, guidewires, a cannulated obturator, a 6.4 mm (outer diameter) access cannula, a suction irrigation valve and cannula stopper, trephines, flexible-tip forceps, a videodiscoscope, suction forceps, and a variety of powered resecting instruments (Fig. 1–7). With uniportal access, resecting instruments and the videoscope are inserted alternately for observation and safe resection. On the other hand, an access cannula with an outer diameter of 12 to 14 mm can be used, permitting exploration of the exiting nerve and foraminal decompression. The lateral recess can be accessed through this cannula, with partial resection of the pars and facet joints. The cannula is also used to perform a mini-laminotomy and extraction of the free fragments. This device has a side fenestration that permits introduction of the videoscope; simultaneous observation and resection can thus be performed. FIGURE 1–7 The YESS (Yeung Endoscopic Spine Surgery) system used for posterolateral diskectomy consists of multichannel endoscopes working cannulas and specialized instruments. The patient is conscious, minimally sedated, and positioned prone on a radiolucent frame. The C-arm is positioned to obtain a lateral view of the spine. An entry site 10 cm from the midline is made, and the needle is advanced in a horizontal plane. The facet joint is often palpated with the tip of the needle, which is then reinserted in a more vertical direction in the safe triangular working zone. Resistance is felt as the needle makes contact with the anulus. In the anteroposterior plane, the needle is in the midpedicular line. At this point, the stylet is removed and a guidewire is passed through it. A small incision is made, and the cannulated obturator is placed over the guidewire and advanced toward the anulus. The access cannula is passed over the cannulated obturator and advanced until it reaches the anulus. Arthroscopic observation of the periannular structures around the annulotomy is performed, with the adipose tissue and superficial veins often requiring coagulation. After observation of the anulus, cottonoid pads soaked in local anesthetic are placed over the anulus. This helps control the pain because the annular surface has a rich supply of sensory fibers. The anulus is penetrated with a 3 mm trephine using a slow rotary motion. A 4.9 mm trephine is then introduced to enlarge the annulotomy. Use of an upbiting forceps further enlarges the annulotomy. The access cannula is advanced until it is engaged in the annular fibers. A suction punch or trimmer blade is used for posterior nucleotomy. Numerous studies on the efficacy of arthroscopic disk surgery have been published. Kambin and colleagues25,27 reported an 87% successful outcome rate with arthroscopic microdiskectomy, and others have reported similar success. In a prospective randomized study evaluating the efficacy of microscopic disk surgery compared with endoscopic disk extraction, Mayer and Brock39 achieved favorable outcomes, with minimal complications, with the percutaneous arthroscopic technique. The reported complications include diskitis, instrument breakage, and psoas hematomas; no neurovascular complications arising from posterolateral access to the intervertebral disks of the lumbar spine have been encountered. Proper patient selection makes this an attractive option, with same-day surgery, negligible blood loss, avoidance of general anesthesia, and minimization of scar tissue all contributing to satisfactory outcomes. Percutaneous Intradiscal Radio-Frequency Thermocoagulation Percutaneous intradiscal nucleotomy using lasers was first performed by Ascher and co-workers in 1987.28,29 The laser energy causes vaporization of the nucleus pulposus, thus reducing the intradiscal pressure and symptoms related to the herniated disk. Radio-frequency lesioning has gained wider acceptance and is used to treat trigeminal neuralgia and create pallidotomy and thalamotomy lesions. The size of the lesion can be well controlled, and the lesion is usually placed around the tip of the electrode. A high-frequency alternating current flows from the tip of the electrode into the tissue. Most radio-frequency lesions are produced at probe temperatures of 60 to 80°C (Fig. 1–8). Intradiscal electrothermal coagulation is a therapeutic innovation specifically designed to treat internal disk disruption. This is a condition in which the disk is rendered painful because of the development of radial fissures extending into the outer one third of the anulus fibrosus. The diagnosis of this condition is based on history and radiological findings, in the form of diskograms and/or postdiskography CT scans. Intradiscal electrothermal coagulation involves percutaneously threading a flexible heating electrode into the disk so that the electrode passes circumferentially around the inner surface of the disk. The heating of the electrode denatures the collagen of the anulus and coagulates the pain fibers supplying the anulus. Direct application of thermal energy to the intervertebral disk produces pain relief via two different mechanisms, thermal coagulation of nociceptors and increased disk stability due to contraction of collagen type I fibers. FIGURE 1–8 Drawing demonstrating intradiscal electrothermal coagulation, performed by percutaneously threading a flexible heating electrode into the disk. A common method of achieving temperatures of 60 to 80°C involves using a needle powered by a radiofrequency generator in the center of the nucleus. Saal and Saal40 developed a novel resistive heating catheter that can be introduced into the anulus and navigated through the nucleus and around the inner wall of the anulus. Oratec Interventions Inc. (Menlo Park, CA) has conducted several clinical trials of intradiscal electrothermal treatment for internal disk disruption. Their study included 140 patients who presented with chronic lower back pain between February 1998 and October 1998. To be eligible for that study, patients were required to satisfy the International Association for the Study of Pain criteria for internal disk disruption: reproducibility of pain with stimulation of the affected disk and CT evidence of a grade III annular tear. Fifty-three patients satisfied the diagnostic criteria and were invited to participate in the study. Of those patients, 36 consented to undergoing intradiscal electrothermal coagulation. The control treatment consisted of an established physical therapy and rehabilitation program. The results of that study indicated that 60% of the selected patients experienced profound reductions in pain.40 All of these studies indicate that, for carefully selected patients, intradiscal electrothermal annuloplasty seems promising, both as a technique to reduce chronic pain of discogenic origin and as an alternative to invasive spine surgery. Spinal Endoscopy In 1931, Burman41 introduced the concept of myeloscopy for direct spinal cord observation. In 1934, Mixter and Barr42 reported an open hemilaminectomy with intraoperative discotomy for treatment of intervertebral disk rupture into the spinal canal. In 1938, Pool43 expanded on Burman’s work and reported myeloscopic inspection of the dorsal nerve roots of the cauda equina. In 1942, Pool44 introduced the concept of intrathecal endoscopy and reported the results of more than 400 myeloscopic procedures. Myeloscopy fell out of favor for a time because of the morbidity associated with insertion of a large-bore scope into the neural cavity. The state of spinal endoscopy remained essentially the same until Ooi et al45 used an endoscope to examine the intrathecal space before surgery. Using improved technology, Ooi et al46 were able to describe pathological features in greater detail, including chronic arachnoiditis and nerve root excursion during claudication associated with lumbar spinal stenosis. Current FDA-approved indications for spinal endoscopy are documentation of pathological features and decompression of structures, direct nerve inspection, inspection of internal fixation, and delivery of therapeutic agents. The current uses of spinal endoscopy have been expanded to include closed decompression of spinal roots, use with lasers, epidural biopsies, percutaneous interbody fusion, and decompression of thoracic disk herniations. Spinal endoscopy has been used to perform thoracoscopy-assisted diskectomies, correction of kyphosis, biopsies, drainage of epidural abscesses, and disk space fusion. In addition, the uses of spinal endoscopy have been expanded to include laparoscopic techniques to treat such lumbar spine pathological conditions as lumbar diskectomy and fusion (anterior vs. posterior). Lumbar Microendoscopic Diskectomy MED to treat nerve root compression is a relatively new procedure that provides minimally invasive access to the spinal column. Medtronic Sofamor Danek (Memphis, TN) developed the instruments and technology (Figs. 1–9 and 1–10). MED combines standard lumbar microsurgical techniques with endoscopy, enabling surgeons successfully to address free-fragment disk pathological factors and lateral recess stenosis. The endoscopic approach allows smaller incisions and less tissue trauma, compared with standard open microdiskectomy. Routine outpatient application and the avoidance of general anesthesia reduce hospital stays and costs. FIGURE 1–9 Photograph of the microendoscopic diskectomy (MED) system: the set of dilators of incremental sizes, and the working channel used for MED. FIGURE 1–10 Photograph of the articulated clamp used to secure the working channel and the endoscope of the microendoscopic diskectomy system. Muramatsu et al47 reported on their series of 70 patients who underwent MED and 15 patients for whom Love’s method was used to treat lumbar disk disease. MED resulted in less blood loss (mean = 12.1 ml) than Love’s method (mean = 59.1 ml); analgesics (suppositories) were required by 52.0% of the MED-treated patients after surgery, whereas all of the patients treated with Love’s procedure required analgesics; and MED reduced the mean number of days before the patients became ambulatory (MED, 1.0 day; Love’s method, 4.9 days). MED has the same indications as open microdiskectomy procedures. Muramatsu et al47 did not use MED to treat patients with herniation associated with segmental instability and lower back pain, patients with combined lumbar canal stenosis and herniation, or patients who had previously undergone back surgery. Guiot et al48 have shown the technical feasibility of percutaneous microendoscopic bilateral decompression of lumbar stenosis via a unilateral approach in a human cadaveric study. Endoscopic Pedicle Screw Fixation of Lumbar Spine The video-assisted posterolateral approach was developed by Boden and associates.49 Posterior keyhole approaches for endoscopic placement of pedicle screws has been done using multiple portals or a single portal between the two pedicles. Muller et al50 described the use of multiple portals in a cadaveric study to identify bony landmarks and to test the feasibility of performing endoscopic pedicle screw fixation. Endius (Plainville, MA) developed a system for a single portal approach for posterolateral transpedicular screw fixation and posterolateral lumbar arthrodesis (Fig. 1–11). In both techniques, a transmuscular approach is performed to place the endoscope over the working area. A needle probe is positioned at the desired level, with biplanar fluoroscopic confirmation. Making a small skin incision around the probe, dilators of incremental size are passed over the probe until the desired blunt-tipped obdurator can be placed. FIGURE 1–11 (A) The Endius system used in percutaneous transpedicular screw insertion. (B) Lateral and axial schematic representation of the Endius system used in transpedicular screw placement. The retractor system can be repositioned using the same entry point to obtain exposure for the adjacent pedicle. Muller et al50 performed a transmuscular insertion of a pedicle screw-rod fixation device using a rigid operating sheath. This sheath (Thoracoport, AutoSuture Co., Norwalk, CT) measured 1.5 cm in diameter and 5 cm in length, and was used with a disposable trocar system consisting of a blunt-tipped obdurator, a threaded sleeve, and a shroud to secure the system. The surgical field was illuminated by an endoscope (Hopkins 11, Karl Storz GmbH & Co., Tuttlingen, Germany) with a diameter of 4 mm, a length of 18 cm, and angles of 0, 30, and 70 degrees. The Diapason pedicle probe (Stryker Instruments, Kalamazoo, MI) with a blunt tip was used to discover whether the pedicle walls were intact. The system has pedicle screws that have a U-shaped screw head.50 The connecting rods are fixed in the screw heads via a ball-ring interface and are locked with locking screws. The pedicle screws are placed using an inclinometer, which provides the surgeon with instant feedback during the placement of pedicle screws. The Endius system consists of similar dilators and probes. In addition, the Endius system has a fan apparatus that allows the surgeon to use blunt dissection techniques to visualize both pedicles and the interspace between them. Thus, this system accomplishes pedicle screw fixation of one motion segment with a single portal in each side. The visualization afforded is large enough to place pedicle screw constructs using rods/plates at the desired levels (Fig. 1–11). Laparoscopic Lumbar Spine Surgery The modern era of laparoscopy began in the 1980s, when Kurt Semm performed the first appendectomy in Germany. Semm, a physician and an engineer, developed many tools that are still in use. The first human laparoscopic cholecystectomy was performed in 1987 by DuBois et al.51 With the advantages of laparoscopic exposures being championed by urological, gynecological, and general surgeons, it is natural that spine surgeons would consider extending the advantages of laparoscopic exposures to the anterior lumbar spine. The significant advantages of transperitoneal laparoscopic surgical treatment include improved observation of surgical anatomic features, marked reductions in postoperative pain, early hospital discharges, and reduced incidence of postoperative ileus. In 1991, Obenchain52 reported the first use of a laparoscopic approach to the lumbar spine for a diskectomy (Fig. 1–12). Regan et al53 described the technique and reported preliminary results for laparoscopic anterior lumbar fusion. Gaur54 was the first to describe an endoscopic retroperitoneal approach for urological procedures, which was later applied to treatment of the lumbar spine. Retroperitoneal, minimally invasive, endoscopic spine surgery has the advantage of not requiring carbon dioxide insufflation or entrance into the peritoneal cavity, and it avoids dissection near the large vessels and the hypogastric plexus. Advances in interbody fusion cage technology have generated a great deal of interest in laparoscopic techniques. Spinal fusion systems inserted with laparoscopic techniques maintain posterior loadbearing elements and reduce postoperative pain. FIGURE 1–12 (A) Lateral radiographs showing fluoroscopic guidance of L5-S1 disk space with positioning of working channel/dilator for performing diskectomy. (B) Preparation of the disk space with drill and tap. (C) Lateral radiographs after laparoscopic insertion of the BAK cage. Additional Minimally Invasive Developments Application of Image-Guidance Systems in the Spine Since its introduction, transpedicular screw fixation has significantly changed the scope of spine surgery.55 Several complex spinal abnormalities, including neoplasms, degenerative diseases, trauma, and infections, are commonly treated with instrumentation to promote fusion via stabilization. The anatomic features of the diseased motion segments and the adjacent neural structures may be distorted and are often difficult to appreciate. Serious complications resulting from screw misplacement or pedicle cortex perforation can lead to devastating neurologic or vascular damage. Pedicle screw fixation provides the most stable construct. To increase the safety of the procedure, various methods have been used to target the pedicle better with respect to the trajectory and depth of screw placement. Most surgeons use assessments of anatomical landmarks, supplemented by fluoroscopy, to identify the anatomical features of the pedicles before placement of the pedicle screws. Weinstein et al56 reported pedicle cortex violation in nearly 20% of cases. Image guidance systems are widely used in intracranial surgery and have been adapted to assist with screw placement since the mid-1990s. The use of image guidance systems for pedicle screw placement has improved the accuracy of placement. The system relies on precise localization of the pedicles with CT. In addition, the transverse width, longitudinal depth, and trajectory angle can be measured (Fig. 1–13). FIGURE 1–13 The virtual fluoroscopy system used for image-guided spinal surgery. Nolte et al57 described the principles of computer-assisted pedicle screw fixation. The overall accuracy of their system was 1.74 mm, using CT scans with 2 mm image increments. Intraoperative surgical exposure of the posterior vertebral elements was performed using standard surgical techniques. An infrared camera (Optotrak; Northern Digital, Waterloo, Ontario, Canada) tracked specific instruments (i.e., pedicle probe, awl, and space pointer) equipped with light-emitting diodes. The dynamic reference was fixed to the spinous process of the vertebra to be instrumented. Normal bony landmarks and their correlations with the images confirmed the calibration accuracy. Using that computerized system, they reported a pedicle screw misplacement rate of 4.3% under clinical conditions. Choi et al58 reported the use of computer-assisted fluoroscopic targeting for pedicle screw fixation. They described a system in which the pedicle entry site and the depth of insertion were determined by intraoperative anteroposterior and lateral fluoroscopic scans. Those authors compared the accuracy of placement with the fluoroscopy-guided system versus the image guidance system and observed no significant differences. Improved accuracy and ease of use with image guidance spine systems will facilitate localization of anatomical bony landmarks and expedite fixation for cervical, thoracic, and lumbar stabilization. With improvements in endoscopic and image-based guidance, the use of a combination of endoscopic and image guidance systems for thoracoscopic or laparoscopic stabilization procedures can be envisioned. Vertebroplasty The spine is composed of a rich trabecular lattice of cancellous bone encased in a hard cortical shell. Moreover, the spine is exposed to degrees of compressive loads and tensile stresses that are in symbiotic biomechanical play with the inner and outer matrixes of the vertebral bodies. Osteoporotic and neoplastic invasion of the vertebral bodies results in erosion of the cancellous network and development of vertebral compression fractures (VCFs), which can contribute to neurologic deficit, gross spinal instability, and ensuing deformity. Surgical management involves significant risks because all of these patients have significant comorbid conditions. It involves internal fixation using screws, plates, wires, cages, or rods and requires extensive surgical exposure. The time required for recuperation from open fixation procedures can be long. Obtaining satisfactory fixation in osteoporotic bone can be technically difficult, and the failure rate for spinal arthrodesis is significant. Open procedures with the implementation of instrumentation were utilized in an attempt to establish pain control and address neurologic compromise. In an attempt to reduce such invasive operative treatment, percutaneous vertebroplasty (PVP) was developed in 1984 by Galibert and Deramond59 in France as a minimally invasive outpatient procedure to offer immediate pain relief by the injection of polymethylmethacrylate (PMMA) bone cement into the vertebral body through a transpedicular percutaneous approach. Although a popular procedure in Europe, PVP was not performed in the United States until 1994.60 This is currently used in the treatment of vertebral fractures involving osteoporosis, primary or secondary tumors of the spine, and spinal trauma. Kyphoplasty In an effort to reduce the high incidence of cement extravasation and detrimental sequelae, infection, cement toxicity, and adjacent fracture development due to an altered sagittal balance, kyphoplasty was developed in the mid-1990s by Dr. Mark Reiley. Kyphoplasty implements inflatable bone tamps inserted by a bilateral transpedicular approach that decreases intravertebral pressure and inserts PMMA into the cavity to elevate the vertebral end plates and restore vertebral height.61 The injection of highly viscous PMMA under lower pressures into a preformed cavity formed by the bone tamps greatly reduces the risk of extravertebral extravasation of the PMMA. Furthermore, kyphoplasty offers immediate pain relief, restores sagittal balance, enhances biomechanical regional milieu, improves vital capacity, thereby increasing mobility, and eradicates the detrimental psychological cascade that often affects patients with VCFs and an altered spinal alignment. In addition, the risk of subsequent vertebral fractures is decreased due to the restoration of the sagittal balance. Although PMMA has been successful in a slue of orthopedic and spine-related procedures, various bioactive substrates possessing osteoconductive and osteoinductive potential have been under investigation as alternatives to PMMA. Endoscopic/Percutaneous Applications of Biological Agents One of the many bioactive substrates under heated investigation are bone morphogenetic proteins (BMPs). These proteins, initially identified in 1965 by Urist,62 are multifunctional cytokines that function as osteoinductive agents in the formation of visceral development, cell proliferation and differentiation, and chondroblast and osteoblast formation. Recombinant BMPs promote and enhance solid fusion, obviate the need for autograft harvest and its associated morbidities, diminish the need for internal instrumentation, avoid reoperation rates associated with nonunions or implant failure, decrease hospital costs, and improve postoperative outcome. Early results, especially with BMP-2 and BMP-7, in animal models and in human subjects have been promising.63,64 Furthermore, heated investigation of the vast potential of gene therapy is ongoing and motivated by the ability of direct application as well as prolonged and desired osteoinduction signaling.65,66 Preliminary results have indicated the promotion of bone and intervertebral disk formation, as well as the encoding of various desired factors. The availability of these bone growth factors and gene therapy opens newer avenues for bone fusion by endoscopic or percutaneous methods to avoid the morbidity and risks associated with fusion by open methods. The field of spine surgery has been witness to immeasurable growth in the past four decades. The evolution of surgical techniques and treatment methodologies is testament to the optimal quality of care each physician seeks to offer the patient. Although there has been an inclination toward more minimally invasive techniques in spine surgery, indications and proper patient selection are essential pragmatic dogmas that must be employed in consideration of an operative treatment modality to obtain optimal clinical outcome and patient satisfaction. REFERENCES 3. Marketos SG, Skiadas P. Hippocrates: the father of spine surgery. Spine. 1999;24:1381–1387. 4. Knoeller SM, Seifried C. History of spine surgery. Spine. 2000;25: 2838–2843. 28. Ascher PW, Heppner F. CO2-laser in neurosurgery. Neurosurg Rev. 1984;7:123–133. 32. Davis JK. Percutaneous discectomy improved with KTP laser. Clin Laser Mon. 1990;8:105–106. 34. Choy DS, Ascher PW, Saddekni S. Percutaneous laser disc decompression. Spine. 1992;17:949–956. 37. Craig FS. Vertebral body biopsy. J Bone Joint Surg Am. 1956;38A: 93–102. 38. Kambin P. Arthroscopic microdiscectomy. Arthroscopy. 1992;8: 287–295. 44. Pool JL. Myeloscopy: intraspinal endoscopy. Surgery. 1942;11: 169–182. 45. Ooi Y Sato Y, Mikanagi K, Morisaki N. [Myeloscopy.] No To Shinkei. 1977;29:569–574. 50. Muller A, Gall C, Marz U, Reulen HJ. A keyhole approach for endoscopically assisted pedicle screw fixation in lumbar spine instability. Neurosurgery. 2000;47:85–96. 52. Obenchain TG. Laparoscopic lumbar discectomy. J Laparoendosc Surg. 1991;1:145–149. 62. Urist M. Bone formation by autoinduction. Science. 1965;150: 893–899. 65. Cha CW, Boden SD. Gene therapy applications for spine fusion. Spine. 2003;28(suppl):S74–84
1
Historical Background of Minimally Invasive Spine Surgery
< div class='tao-gold-member'>
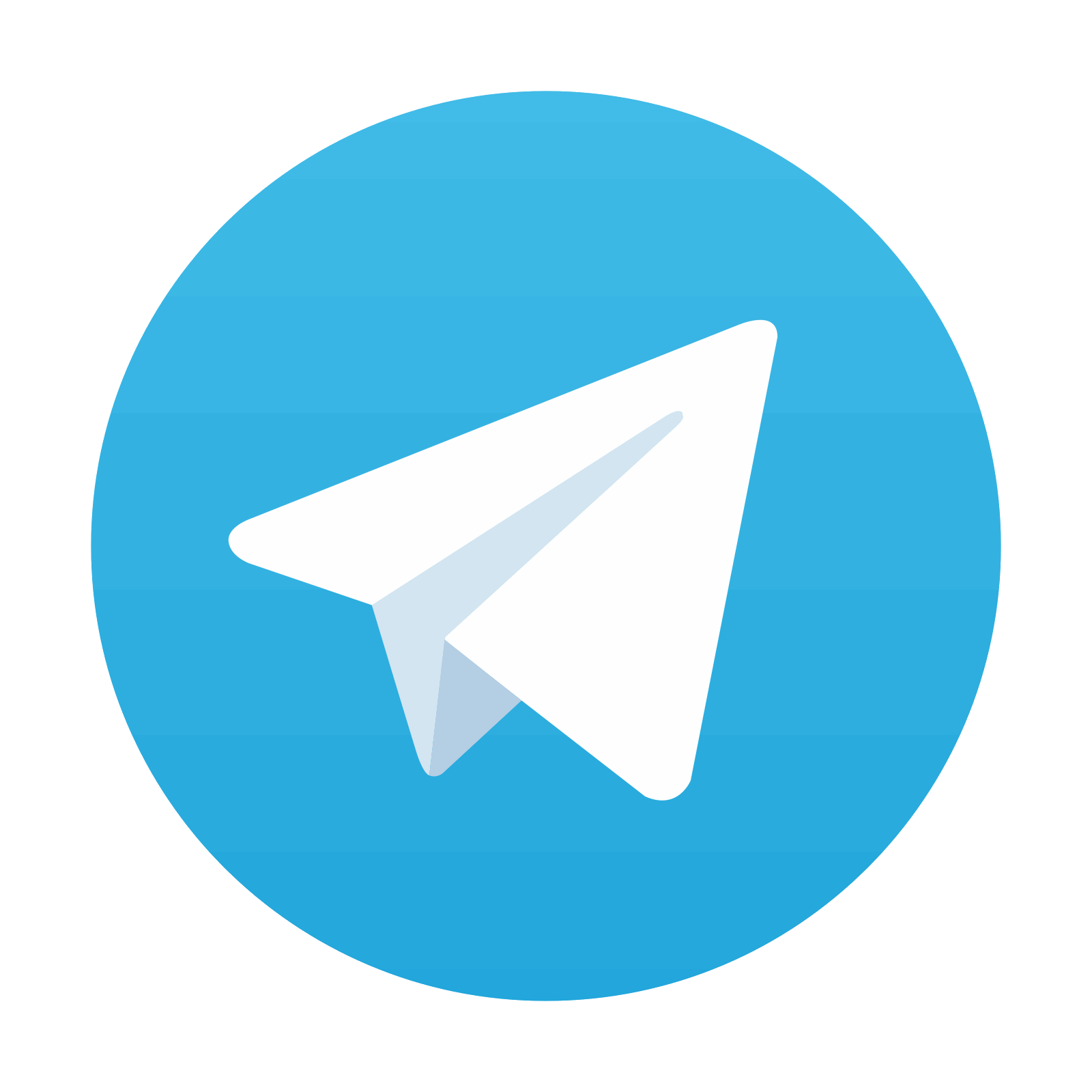