Infraslow EEG Activity
Sampsa Vanhatalo
Juha Voipio
Kai Kaila
INTRODUCTION
The conventional way of recording EEG is more than half a century old. The early pioneers were faced with the challenge to design routine EEG techniques that enabled recordings of the most salient features in the human EEG known at that time. A major obstacle was encountered in attempts to faithfully record slow events. Electrode drifts that produced artifactual slow signals tended to contaminate the recordings and to saturate the amplifier’s dynamic range, and they also pushed the polygraph recorder pens out of scale. In order to circumvent these problems, the EEG amplifiers became furnished with an inbuilt high-pass filter. Hence, all kinds of slow signals, whether physiologic or artifactual, were eliminated.
In practice, the technical compromise described above means that the conventional EEG has a poor low-frequency response, which results in attenuation and distortion of signals at <0.5 Hz. This is in sharp contrast with a wealth of data showing that the physiologic frequency range of human EEG signals ranges from infraslow (0.01 Hz or even less; this chapter) to ultrafast (up to several hundreds of hertz; Chapter 37) frequencies. In this chapter, several lines of evidence will be presented to demonstrate that, at the present stage of EEG technology, stability is not an issue that would require any trade-off at the expense of low-frequency response. Notably, filtering off the lower end of the EEG spectrum by the standard high pass at around 0.5 Hz can lead to a situation where the most salient features of the EEG are effectively deleted. This kind of a massive loss of relevant data is clearly evident, for instance, in standard EEG recordings of the immature human brain and during epileptic events.
Because of historical reasons, the terminology related to recording slow EEG events is somewhat confusing. The term direct current EEG (DC-EEG) has been widely used in older literature to imply a (ideal) frequency response of the EEG with a minimum at 0 Hz. However, the term “DC” has at least two connotations, which are misleading. First, it is impossible to have a per definition 0 Hz response, since at 0 Hz the signal level is constant and never changing. Second, the use of the term DC-EEG puts an a priori emphasis on the low-frequency part of the EEG spectrum but, as we will show below, looking at low frequencies does not compromise any simultaneous analyses carried out at fast or even ultrafast frequencies. The results described here show that EEG responses, with a time course of several minutes to achieve their peak levels, can be easily recorded if attention is paid to trivial aspects of EEG electrode and amplifier design and characteristics (1, 2, 3, 4 and 5).
Hence, instead of the old “DC-EEG” nomenclature, we prefer using the term Full-band EEG (FbEEG). This conveys the idea that the full, physiologically and clinically relevant EEG bandwidth can be readily examined without favoring any frequency band at the expense of another. The term DC shift is used here to indicate slow shifts in FbEEG baseline that would go unnoticed in conventional EEG. DC-coupled amplifiers are devices that record electrode signals without elimination of the FbEEG baseline by inbuilt high-pass filters.
EVENTS VERSUS OSCILLATIONS
In the context of infraslow activity, it is especially important to acknowledge the distinction between events and oscillations (6). A variety of EEG phenomena with a duration of seconds are, indeed, not genuine oscillations but discrete events. As will be shown below, spontaneous activity transients (SATs) in preterm babies or arousal shifts in older subjects are very slow transient events nesting complex patterns of high-frequency EEG activity, whereas ictal DC shifts during epileptic seizures are seen as slow deflections in the recording baseline. Although the infraslow activity seen during sleep or cognitive tasks clearly reflects cyclic changes, a well-defined oscillation frequency is not discernible in the spectrograms. Hence it has been called either infraslow oscillation (ISO) (7) or infraslow fluctuation (ISF) (8).
TECHNICAL ASPECTS
Recording of a genuine FbEEG signal sets requirements both to the amplifier and to the electrode-skin interface. It is important to note that these requirements are not only related to the DC stability already mentioned above, but addition of infraslow and ultrafast frequency ranges introduces new sources of noise that are of technical or of biologic origin (e.g., baseline drifts and electrical interference from other devices or skin potential, respectively). Practical solutions to overcome these problems are, however, well established, often trivial, and widely available in several modern higher end installations.
Regarding the amplifier, detection of the infraslow events requires a genuine DC-coupled amplifier with a sufficiently high input impedance and DC stability as well as a wideenough dynamic range (preferably ± 100 mV or higher), whereas detection of the ultrafast frequencies requires a highenough sampling rate and a large data storage capacity. Several clinically suitable, DC-coupled amplifiers of this kind have recently become commercially available.
Regarding the electrode-skin interface, detection of the infraslow events requires a DC-stable electrode-skin interface (Fig. 36.1). This is achieved by using electrode materials that are nonpolarizable (in practice Ag/AgCl) together with a gel containing chloride, which is an absolute requirement for obtaining a stable electrode potential (3). In addition, electrodes need to be firmly fixed on the skin to minimize artifacts caused by gel leakage or drying. Drying of the gel gives rise to an increase in its electrolyte content, and, for example, a gradual 10% increase in the Cl– concentration seen by the Ag/AgCl electrode will generate a negative drift of 2.5 mV. Finally, skin-borne signals (9) caused by electrode movements or galvanic skin reactions (GSR/SSR) can be strongly suppressed or eliminated by short-circuiting the epithelium at the recording site (various techniques are available for this purpose, see also Refs. 10, 11). Sufficient short-circuiting of the skin is painless enough to be performed in even sleeping neonates (12).
Compromises in recording setup are sometimes necessary, especially under clinical conditions (13,14). For instance, if the electrode-skin interface is not optimized as described above (Fig 36.1), most slow events are hard if not impossible to be distinguished from noncerebral artifacts. The situation is, of course, different if the subjects are calm and cooperative (as in research labs) as opposed to recording restless patients (as in intensive care units). Our own experience has shown that a suboptimal electrode-skin interface may still give reasonably good clinical recordings in calm and/or sleeping patients (4,5,15) if the EEG events of interest are phasic with relatively short duration (such as SATs in preterm babies, see section “Slow Activity in the Preterm Human EEG”). However, recordings of longer responses (such as ISOs during sleep) or phasic events accompanied with changes in the autonomic nervous system (such as arousal shifts, which may include GSRs) will no longer be reliable. Movement artifacts and slow baseline drifts will also be very much larger if the transepithelial potential is left intact under the electrode. Another compromise that is sometimes encountered is the use of polarizable (DC unstable) electrodes in combination with high-pass filtering at an extremely low frequency. While such recordings may be used to detect genuine DC shifts of a foreseeable duration, they may equally give spurious results if the evident technical pitfalls are ignored. Unlike reversible electrodes that maintain their electrode potential and provide a primarily resistive coupling of slow signals across the electrode interface, capacitive signal coupling plays a major role in polarizable electrodes. Therefore, it is possible that the low-frequency cutoff is not set by the selected high-pass filter but rather by the circuit formed by the electrode and the amplifier input, resulting in unpredictable distortion of slow signals. Furthermore, in this kind of recordings slow fluctuations of the drifting electrode potential (3) will appear as infraslow noise on an apparently stable baseline.
The challenges of interpretation of FbEEG data are mostly similar to those of conventional EEG data. Lack of high-pass filtering in FbEEG changes the visual appearance of slow artifacts (e.g., eye and tongue movements) (16, 17 and 18), but their visual recognition in FbEEG recordings is easy with little experience. Median filters and/or spatial filters, for near-complete removing of many artifacts, are now readily available in pertinent analysis software packages. Skin-borne potentials (“sweat artifacts”), in turn, do not cause problems in FbEEG recordings if they are excluded by short-circuiting (see above).
SLOW ACTIVITY IN THE PRETERM HUMAN EEG
In FbEEG recordings from preterm neonates the most salient frequency range that dominates the total spectral power is much below the conventional EEG frequency band (Fig. 36.2B) (12,15,19). This is consistent with previous well-known observations that an abundance of slow activity is a major feature of EEG activity in the immature brain (20,21) (see also Chapters 9 and 25). In babies born 2 or more months before term age, the spectral power peaks at frequencies as low as 0.01-0.1 Hz. It is obvious that when recorded using conventional EEG, this kind of activity must be highly attenuated and distorted by filtering. Hence, only faint echoes of the slow neonatal EEG activity can be detected at around the lower edge of the conventional recording bandwidth.
Most notably, FbEEG showed that the preterm human EEG exhibits very slow (up to 5 seconds) SATs. Furthermore, most of the faster EEG activity is nested within these slow events, and hence, practically all of the EEG activity of the most immature cortex is associated with SATs (Fig. 36.2A) (12,15). This is an extremely interesting finding in itself and in light of animal experiments that have demonstrated that endogenously driven, spontaneous activity is crucial in shaping neuronal connectivity in the developing brain wiring already at an immature stage where sensory input has little or no role at all (reviewed in Refs. 19, 22, 23). Moreover, it has been shown that sensory input to the as yet poorly wired brain is able to trigger
self-organized network events of this kind (Fig. 36.2C) (reviewed in Ref. 24; see also Ref. 25).
self-organized network events of this kind (Fig. 36.2C) (reviewed in Ref. 24; see also Ref. 25).
![]() Figure 36.2. Slow activity in the perinatal human cortex. Specimen recording of FbEEG activity (at O1 vs. Cz) of a premature neonate at 33 weeks of conceptional age shows prominent spontaneous activity transients. FbEEG data (A, lower trace) was high-pass filtered off-line at 0.5 Hz (upper trace) in order to demonstrate the pronounced loss (attenuation, distortion) of slow activity. This is also clearly seen in the power spectrum of FbEEG data from the same experiment (B). Note the small fraction of the total EEG power above the conventional cutoff frequency of 0.5 Hz (dashed line). FFT was obtained from three 3-minute segments, using 60-second Hanning window. (C) Tactile stimulation (asterisk) to palm area in a preterm baby (30 weeks conceptional age) results in very prominent EEG events in the contralateral electrode (C3) above somatosensory cortex. These events are characterized by a slow-wave nesting faster activity, and they have striking similarities to some SAT events generated spontaneously (i.e., without apparent stimuli) in the same cortical area (19). |
FbEEG recordings do not only unravel a fully novel type of activity in the immature human cortex in the low-frequency bandwidth. In addition, it is obvious that these data call for a revised nomenclature for the perinatal EEG (19,22). Future studies will show whether work of this kind might set the stage for the identification and diagnostics of activity-dependent diseases and malfunctions of the immature brain as proposed by Shatz et al. (26).
INFRASLOW EEG OSCILLATIONS AND VOLTAGE SHIFTS DURING SLEEP
Using FbEEG, ISOs that take place at a wide range of frequencies (0.02-0.2 Hz) with an amplitude of up to several tens of microvolts are readily observed during non-REM sleep (7). Interestingly, the phase of ISO shows a robust correlation with higher frequency EEG activities (Fig. 36.3) as well as with phasic brain events, such as the K-complex or interictal epileptiform activity. ISOs recorded by FbEEG in humans may thus reflect slow cyclic modulation of cortical excitability under both physiologic and pathophysiologic conditions. This idea fits well with results obtained in in vivo experiments on rats (27), rabbits (28), and monkeys (29).
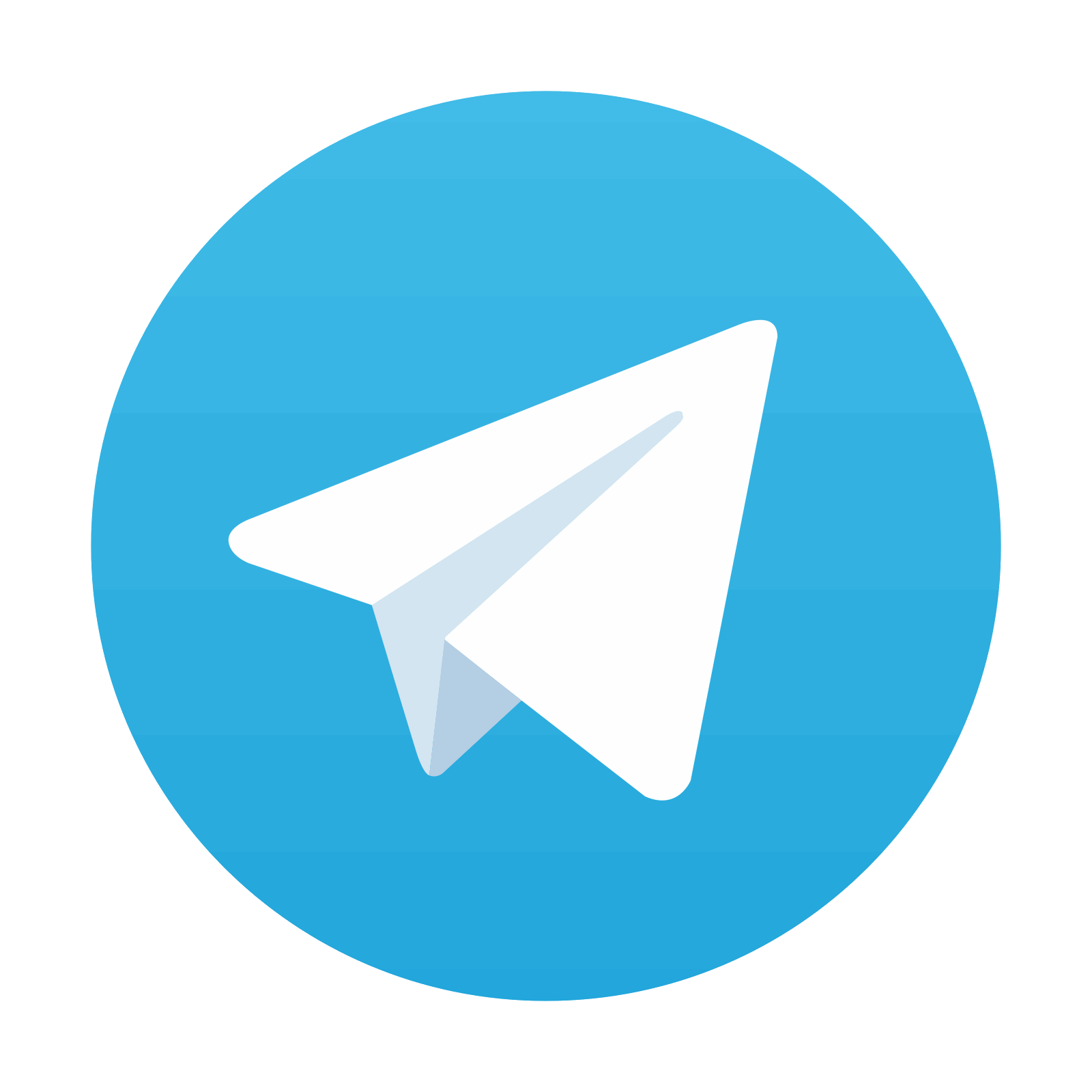
Stay updated, free articles. Join our Telegram channel

Full access? Get Clinical Tree
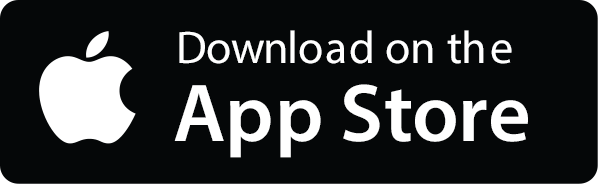
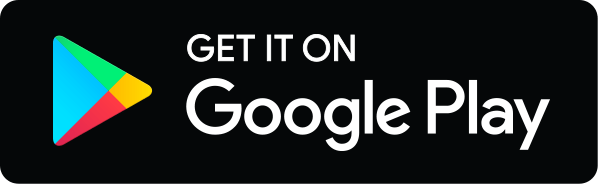