Abstract
This chapter provides a systematic review on intestinal electrical stimulation. First, intestinal motility and intestinal myoelectrical activity are introduced. Abnormalities in intestinal motility and intestinal electrophysiology in various clinical conditions are discussed. Different methodologies that have been applied in research and clinical settings are presented, including different patterns for stimulation pulses and delivery time, and different electrode placement. Effects of intestinal electrical stimulation on intestinal myoelectrical activity, contractions, and transit are also discussed, together with possible mechanisms. Finally, potential applications of intestinal electrical stimulation are discussed, including possible therapies for treating various intestinal disorders associated with impaired intestinal motility obesity and diabetes.
Keywords
Diabetes, Gastrointestinal motility, Intestinal electrical stimulation, Obesity, Small intestinal slow waves
Outline
Introduction 1397
Physiology and Pathophysiology of Intestinal Motility 1398
Small Intestinal Motility 1398
Small Intestinal Myoelectrical Activity 1398
Intestinal Motility Disorders 1399
Methodologies 1399
Placement of Stimulation Electrodes 1400
Effects and Mechanisms on Intestinal Functions 1400
Effects of Intestinal Electrical Stimulation (IES) on Intestinal Slow Waves 1401
Effects on Intestinal Slow-Wave Dysrhythmia 1401
Effects and Mechanisms of Intestinal Electrical Stimulation (IES) on Intestinal Motility 1402
Effects and Mechanisms of Intestinal Electrical Stimulation (IES) on Intestinal Transit and Absorption 1402
Effects of Intestinal Electrical Stimulation (IES) on Other Organs 1403
Effects and Mechanism of Intestinal Electrical Stimulation (IES) on Glycemic Control 1403
Potential Applications 1403
Discussion and Conclusions 1404
References 1405
Introduction
Although the earliest study of electrical stimulation of the gut was reported more than 40 years ago, the development of the field is slow when compared to cardiac pacing or other areas of neuromodulation including spinal cord stimulation, deep brain stimulation, and motor cortex stimulation. In the earliest study regarding intestinal stimulation, published in , Bilgutay et al. reported the use of intraluminal electrical stimulation via the tip of a nasogastric tube to induce peristalsis and shorten the recovery period from ileus after laparotomy; an increase in gastric contractions as well as gastric emptying was reported in both dogs and humans. However, subsequent randomized controlled studies failed to produce consistent or promising results ( ). Before the 1960s, there was a lack of understanding of gastrointestinal (GI) electrophysiology, which only became a topic of interest in the later 1960s and early 1970s ( ). From 1970s to early 1990s, Kelly and colleagues made significant contributions to our understanding of the electrical stimulation of the gut; numerous studies in both dogs and humans were reported by this group ( ).
Starting from 1990s, more progress has been made on the methodologies, effects, mechanisms, and clinical applications of GI electrical stimulation. Numerous reports are available in the literature on electrical stimulation of various organs of the GI tract, such as the stomach, small intestine, colon, and rectum for the treatment or therapeutic potentials of various conditions such as gastroparesis, short bowel syndrome, intestinal pseudo-obstruction, and fecal incontinence ( ). This chapter will focus on intestinal electrical stimulation (IES). Topics on gastric electrical stimulation can be found in a separate chapter of this book and a number excellent reviews ( ).
The major difference between electrical stimulation of the gut and neuromodulation lies in the target of stimulation. Neuromodulation typically has its target on nerves or nervous systems to change functions of an organ, whereas electrical stimulation of the gut does not necessarily target on specific nerves or nervous systems and may directly change various functions of an organ in the gut, such as neural, hormonal, and mechanical functions. In addition, the stimulation methodologies of IES may be different from other aspects of neuromodulation, mainly because of the following two reasons: (1) the GI organ is composed of smooth muscles. The response of smooth muscles to electrical stimulation is slow, and therefore long pulses (in the order of msec instead of μs) are typically required to alter the function of the organ being stimulated; (2) the GI organ has intrinsic myoelectrical activity and therefore the electrical stimulation of the gut may be designed to enhance or alter this intrinsic myoelectrical activity.
Physiology and Pathophysiology of Intestinal Motility
Small Intestinal Motility
The main function of the stomach is to accommodate ingested food, grind and fix the food, and finally pump it out of the stomach to the small intestine. The accommodation of the ingested food is achieved by relaxation of the proximal stomach via the release of an inhibitory neurotransmitter called nitric oxide, whereas emptying of the stomach is accomplished by sequentially propagated contractions, called peristalsis, from the corpus to the distal antrum.
The main function of the small intestine is to absorb various nutrients from the ingested food emptied from the stomach. Intestinal motility is organized in such a way that the organ has sufficient time to absorb needed nutrients from the ingested food and then transport the remaining down to the ileum and the colon. Accordingly, intestinal motility after a meal is characterized by segmental contractions with different propagation directions, including antegrade, retrograde, and simultaneous contractile patterns. The force of postprandial intestinal contractions is moderate, about 50%–70% of the maximum strength. The intestinal motility pattern in the fasting state undergoes cycles of periodic fluctuation divided into three phases, called migrating motor complex (MMC): Phase I (no contractions, 40–60 min), Phase II (intermittent contractions, 20–40 min), and Phase III (regular rhythmic contractions, 2–10 min). Typical gastric and intestinal contractions in the fasting state are shown in Fig. 117.1 . The maximum frequency of the stomach is about 3 cycles/min (cpm) and 12 cpm in the most proximal small intestine, duodenum and 8–9 cpm in the most distal intestine, ileum ( ).

Small Intestinal Myoelectrical Activity
It is important to understand electrophysiology of the small intestine for IES because (1) intestinal motility is regulated by intestinal myoelectrical activity, and (2) to alter the function of the small intestine, electrical stimuli may have to be designed to enhance or inhibit this intrinsic, intestinal myoelectrical activity.
Myoelectric activity of the small intestine is similar to that of the stomach. It consists of two components: pacesetter potentials or slow waves and spike potentials. A typical recording showing intestinal slow waves and spikes are shown in Fig. 117.2 . Small intestinal slow waves originate from a region in the proximal 1 cm of the duodenum and propagate as an annular wave front in an abroad direction ( ). These slow waves determine the frequency and the direction of propagation of intestinal contractions. Spike potentials are superimposed on the slow waves and are electrical counterparts of contractions ( ).

In the dog, the proximal 10%–30% of the small intestine (30–115 cm of duodenum and jejunum) maintains the same slow-wave frequency, 18–20 cpm, in a region called the “frequency plateau” ( ). Aborad 1
1 Aborad: direction away from the mouth.
to this point, there is a diminishing slow-wave frequency gradient along the small bowel to a rate of 14 cpm in the distal ileum ( ). In humans, slow waves in the duodenum and proximal jejunum occur at about 12 cpm with an aborad gradient to about 9 cpm in the terminal ileum ( ). Whether a proximal plateau of identical frequencies is present in the human duodenum and proximal jejunum has not been clearly shown ( ). Transection and reanastomosis of the small bowel decreases the slow-wave frequency in the distal segment in both dogs ( ) and man ( ). In addition, at least in dogs, the propagation of slow waves in the distal segment becomes abnormal, with a high percentage of these slow waves propagating in an orad 22 Orad: direction to the mouth.
, rather than an aborad direction ( ).Studies have indicated that intestinal myoelectrical dysrhythmia is associated with intestinal motor disorders in some clinical settings ( ). It is known that abnormalities in the frequency of intestinal slow waves are associated with intestinal hypomotility and that uncoupled or dysrhythmic intestinal myoelectrical activity leads to a lack of coordinated intestinal contractions or peristalsis.
Intestinal Motility Disorders
Intestinal dysmotility is often seen in patients with irritable bowel syndrome (IBS) and patients with chronic, intestinal pseudo-obstruction 3
3 http://en.wikipedia.org/wiki/Pseudo-obstruction Intestinal pseudo-obstruction is the decreased ability of the intestines to push food through, and often causes dilation of various parts of the bowel. It can be a primary condition (idiopathic or inherited from a parent) or caused by another disease (secondary). The clinical and radiological findings are often similar to true intestinal obstruction. Retrieved from wikipedia on January 29, 2008.
. Intestinal dysmotility is featured with altered MMC patterns in the fasting state and hypotensive or hypertensive postprandial contractions. Altered MMC patterns include a complete loss of the fasting MMC pattern, a loss of Phase III and a prolonged Phase II, and abnormal propagation of Phase III activities. Postprandial contractile abnormalities include a continuation of fasting MMC pattern, absence or decreased amplitude of contractions and abnormal propagation of contractions. Uncoordinated hypertensive contractions may also occur in patients with neuropathy.Methodologies
Methodologies of IES include patterns of stimuli, placement of electrodes, and delivery time of stimuli. In this section, various methods published in the literature are summarized and critically discussed.
Long-pulse stimulation : This method is most frequently reported in the literature because it is able to “pace” or entrain natural slow waves. It is also called electrical pacing or intestinal pacing. In this method, the electrical stimulus is composed of repetitive single pulses with a pulse width in the order of milliseconds rather than microseconds (10–600 ms for IES), and a stimulation frequency in the vicinity of the physiological frequency of the intestinal slow wave (see Fig. 117.3A ). However, it should be noted that currently there are no implantable devices available in the market capable of generating pulses with a width longer than 2 ms.

Short-pulse stimulation : In contrast to long-pulse stimulation, the pulse width in this method is substantially shorter and is in the order of a few hundred microseconds (μsec). The stimulation frequency is usually a few times higher than the physiological frequency of the intestinal slow wave (see Fig. 117.3B ). Most of commercially available cardiac pacemakers or nerve stimulators are capable of generating short pulses.
Pulse train : In this method, the stimulus is composed of repetitive trains of pulses and is derived from the combination of two signals: (1) continuous short pulses with a high frequency (in the order of 5–100 Hz); (2) a control signal to turn the pulses on and off, such as x seconds “on” and y seconds “off.” The addition of x and y then determines the frequency of the pulse train ( Fig. 117.3C ). This kind of stimulation has been frequently used for nerve stimulation. Commercially available stimulators are capable of generating trains of pulses with a pulse width of below 2 ms.
Dual pulses : A novel method of IES, called dual-pulse IES, has recently been proposed by combining short and long pulses ( ). In this method, the stimulus is composed of a short pulse (in the order of a few hundred μs) followed with a long pulse (in the order of a few hundred ms) (see Fig. 117.4 ). A canine study has shown that dual-pulse IES is capable of both normalizing intestinal dysrhythmia and improving symptoms suggestive of nausea and vomiting induced by infusion of vasopressin ( ). Apparently, the proposed method of dual pulse IES is more attractive than the conventional method of electrical stimulation in which only short pulses or long pulses but not both are utilized.

Synchronized stimulation : Conventionally, electrical stimulation is performed at a fixed frequency delivered at random without consideration of the occurrence of the intrinsic, intestinal slow waves. A novel method has been proposed: synchronized IES ( ). Synchronized IES requires the implantation of two pairs of electrodes, one for the detection of intestinal slow waves and the other for stimulation. In this proposed method, each electrical stimulus is delivered upon the detection of an intrinsic, slow-wave peak, that is, IES is performed at the occurrence of cyclic, physiologic, electrical events of the small intestine ( Fig. 117.5 ). By synchronizing each electrical stimulus with the intrinsic, physiologic electrical activity, it is hypothesized that it is capable of enhancing intestinal contractions. A recent canine study showed that synchronized IES in the fed state significantly accelerated intestinal transit ( ).

Placement of Stimulation Electrodes
- 1.
Serosal electrodes . Most commonly, stimulation electrodes are placed on the serosal surface of the small intestine. The advantage of this method is the guaranteed contact and direct effect on the targeted organ. The disadvantage is its invasiveness. Laparotomy or laparoscopy under general anesthesia is required.
- 2.
Intraluminal or mucosal electrodes . Alternatively, stimulation electrodes may be placed on the mucosal surface of the small intestine via a nasojejunal tube. Typically, ring electrodes are attached to the tip of a catheter that is placed into the small intestine via the nose ( ). The advantage of this method is that surgery is not a requisite. The intubation of the catheter can be accomplished with or without the aid of endoscopy. The disadvantage is that this method may not be adequate for chronic stimulation.
Effects and Mechanisms on Intestinal Functions
Effects of IES on intestinal motility and possible mechanisms related to IES have been investigated in a number of studies. IES has been shown to pace or entrain intestinal slow waves and normalize intestinal, slow-wave dysrhythmia. Intestinal contractions can be inhibited with IES of high stimulation energy but may be enhanced with synchronized IES. Similarly, intestinal transit may be inhibited or enhanced with IES of appropriate parameters, resulting in an alteration in absorption. Moreover, the effects of IES on organs along the gut have also been explored.
Effects of Intestinal Electrical Stimulation (IES) on Intestinal Slow Waves
The effect of IES or intestinal pacing on intestinal slow waves was investigated systematically in a canine model ( ). Long pulses with a pulse width of 50–150 ms are required to entrain or pace the intrinsic intestinal slow waves. It has been reported that intestinal slow waves can only be entrained when IES is delivered at a frequency higher than the frequency of the intrinsic slow waves; a complete entrainment of intestinal slow waves can be achieved only when the stimulation frequency is equal to or below 110% of the intrinsic frequency. When the stimulation frequency is higher than 110% of the intrinsic frequency, only partial entrainment is possible as shown in Fig. 117.6 . Similarly, entrainment of intestinal slow waves can also be accomplished with IES using intraluminal ring electrodes ( ).

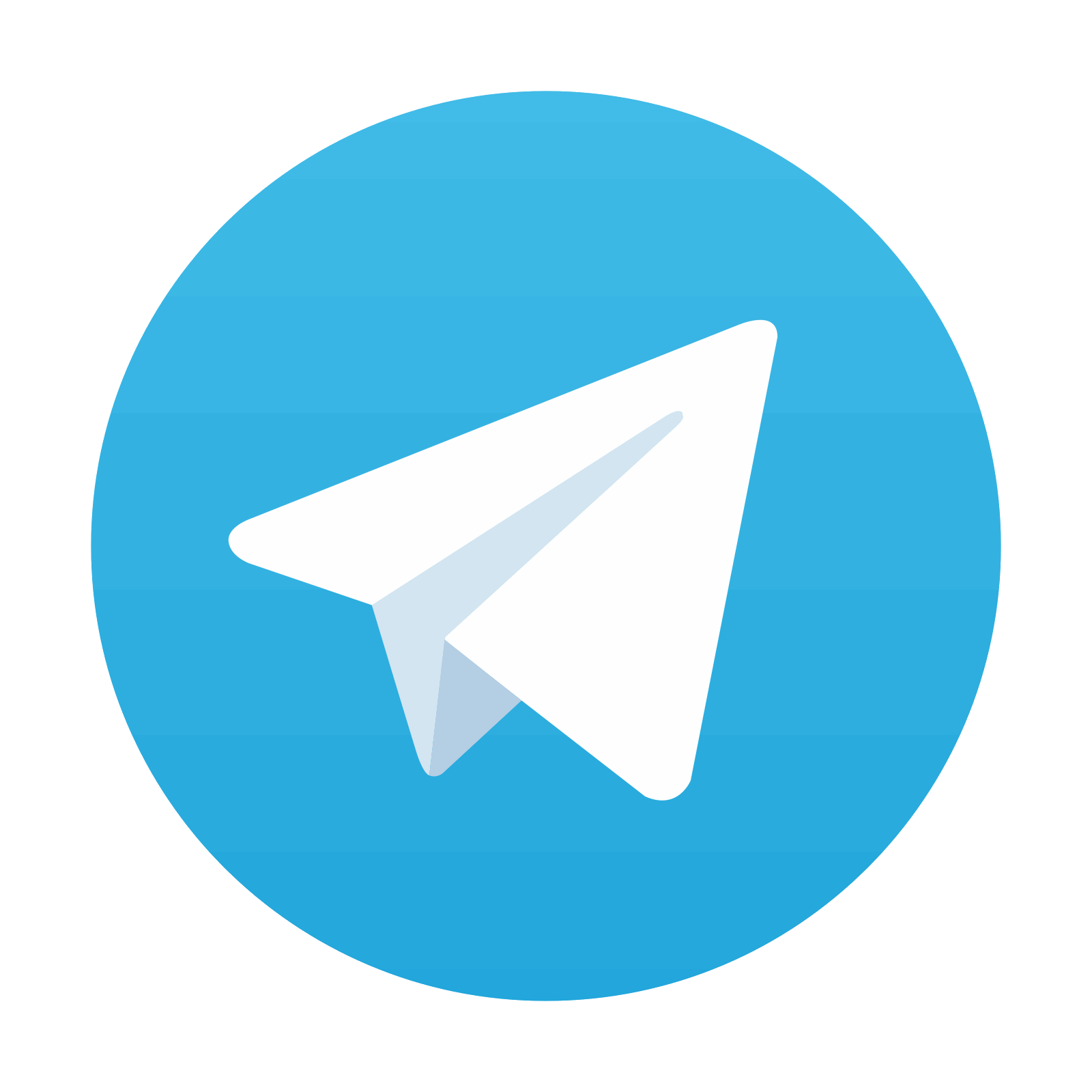
Stay updated, free articles. Join our Telegram channel

Full access? Get Clinical Tree
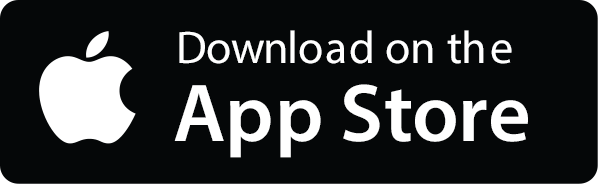
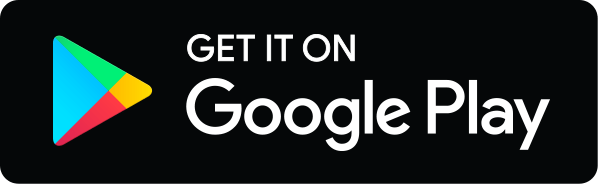
