Figure 43.1. Schematic representation of the ischemic tissue after a major vessel occlusion. In black there is represented the ischemic core, irreversibly damaged. The ischemic penumbra is represented by the “A” area. This is the tissue that can be salvaged after the use of thrombolytic therapy. Surrounding the ischemic penumbra, there is the benign oligaemia area (“B” area), which will survive regardless there is reperfusion or not.
Therefore, ischemic penumbra is the potentially salvageable cerebral ischemic tissue that is present around the ischemic core. This concept arose by clinical observations of fluctuating neurological deficits that suggested different perfusion thresholds for loss of neuronal function and cell death. After the description of these thresholds in experimental models of ischemia, penumbra was defined as the portion of the ischemic zone with reduced cerebral blood flow, absent electrical activity, but preserved ion homeostasis and transmembrane electrical potentials. However, in clinical practice it is more relevant to define ischemic penumbra as the portion of the ischemic tissue that is still potentially viable.
Penumbral tissue is an abnormal tissue with physiological and biochemical characteristics of cellular dysfunction but not cellular death, caused by hypoperfusion. This tissue has to be in the same vascular territory as the infarct core. The main relevance of this tissue is that it can either survive or progress to necrosis, and its salvage is associated with better clinical outcome. Thus, ischemic penumbra can be defined as the ischemic tissue which function is impaired and it is at risk of infarction. The clinical relevance is, therefore, that this tissue can potentially be salvaged if there is reperfusion. If reperfusion is not achieved this tissue at risk will be recruited into the infarct core.
The tissue surrounding the infarct core remains viable, but severely compromised because of metabolic, hemodynamic, and neurochemical alterations characteristic of the ischemic environment. In this region, endogenous protective mechanisms (i.e., local expression of survival factors) and residual collateral blood flow are only able to delay the otherwise inevitable cell death process leading to infarct growth. In normal brain an increase in local glucose metabolism is closely coupled to a reactive increase in local CBF to match the metabolic demands. The dissociation between metabolism and flow is pronounced in focal cerebral ischemia. Maintenance or focal increases in glucose utilization have been observed in brain regions adjacent to the ischemic core. Therefore, the uncoupling of metabolism and flow is an important pathogenetic mechanism that affects the critical energy balance in the penumbra.
Lastly, the ischemic penumbra is a dynamic tissue structure of limited viability surrounding a focus of dense cerebral ischemia. The flow thresholds for electrical and membrane failure are not constant but increase in a time-dependent manner. Therefore, with time increases the viability of the penumbra will decrease and more tissue will be recruited in the core.
43.3 Neuroimaging Tools to Assess Ischemic Penumbra
Just a conventional cranial computed tomography (CT) is enough to select stroke patients to receive alteplase in the 3 hour time window (or 4.5 hours if we take into account the results of ECASS III). However, many authors defend the use of more sophisticated technology to improve the selection of patients and the avoidance of hemorrhagic complications, but there is not enough evidence to recommend further neuroimaging assessment in the approved time window.
While conventional CT can detect hemorrhage and the ischemic core, the new neuroimaging tools are able to characterize the ischemic penumbra by assessing brain perfusion and metabolism. This information can help us to extend the therapeutic window, to exclude patients that do not require thrombolysis because they do not have penumbra, to detect patients who more probably will be harmed by treatment or are unlikely to respond (for example in the malignant pattern in which a very large diffusion weighted image on MR is matched by a similarly sized perfusion image), or to select patients that have not responded to intravenous alteplase to rescue intra-arterial thrombolysis.
There are many neuroimaging tools that have shown efficacy in penumbra assessment. However, there is lack of consensus on the appropriated method to measure penumbra.
43.3.1 Positron Emission Tomography
Positron emission tomography (PET) is a nuclear medicine imaging technique which produces a three-dimensional image of functional processes. It is based on the detection of pairs of gamma rays emitted by a positron-emitting radionuclide (tracer), which is introduced into the body on a biologically active molecule. Multitracer PET using the ligands H215O and 15O2 has been used to quantitatively measure cerebral blood flow (CBF), cerebral metabolism of oxygen (CMRO2) and cerebral oxygen extraction fraction (OEF). The quantitative measurement of CBF, CMRO2, OEF and cerebral blood volume (CBV) allows the assessment of perfusion and energy metabolism. Thus, in ischemic stroke is possible to detect the four tissue subtypes: core, penumbra, oligaemia and unaffected tissue, as well as the thresholds that reliably and efficiently separate them.
11C-flumazenil is a PET radioligand that binds to the benzodiazepine site on the neuronal [gamma]-aminobutyric acid receptor and has been applied with 15O2 tracers in acute stroke patients. This ligand it is able to distinguish ischemic core from penumbral tissue early after acute stroke. 11C-flumazenil binding below 3.4-times the mean of contralateral white matter predicts irreversible tissue damage, and a CBF of 14.1 ml/100 g per minute is the penumbral threshold.
Another marker, 18F-fluoromisonidazole, provides a direct image of the penumbra, but not of the ischemic core. Studies with 18F-fluoromisonidazole have shown that penumbra may last for long periods in some patients, and that penumbral salvage is time-independent, with at least 50% tissue survival even beyond 12 h.
PET is considered the gold standard to study ischemic penumbra, because it provides quantitative mapping of many physiological parameters. However, its utilization is not practical in clinical studies, because of it complexity, cost, and its limited availability.
43.3.2 Magnetic Resonance Imaging
The ischemic penumbra can be evaluated with magnetic resonance imaging (MRI), using diffusion-weighted (DWI) and perfusion-weighted (PWI) sequences. Currently, DWI and PWI MRI studies are the most widely used clinical imaging modalities to detect the presence of penumbra.
DWI measures alterations in the diffusion of water molecules. The degree of free diffusion of water molecules can be quantified with the apparent diffusion coefficient (ADC). The changes detected with DWI are due to metabolic failure leading to disruption of ion homeostasis and cytotoxic edema. The DWI image visualizes acute ischemic lesions within minutes, and identifies acute infarcts within 6 hours after onset with high sensitivity and specificity. The hyperintense lesions represent the irreversible damaged brain tissue, so the altered region detected with DWI is a reasonable representation of the infarct core.
PWI detects the signal loss of flow that occurs during the dynamic tracking with the use of gadolinium, an intravenous paramagnetic contrast agent. With this technique it is possible to delineate ischemic areas as an abnormal perfusion area, which is commonly defined by time domain parameters on dynamic contrast-enhanced imaging, including mean transit time (MTT), time to peak (TTP), or Tmax. MTT is the time between arterial inflow and venous outflow. TTP represents the time from the beginning of gadolinium injection to the maximum enhancement within an intracerebral region of interest. Tmax is the time to peak of the residue function, indicating bolus delay between the site of selection of the arterial input function and the tissue. Therefore, Tmax represents bolus arrival time, without the confounding effects of bolus administration.
The mismatch between a PWI lesion and a DWI lesion is the MRI signature of the ischemic penumbra. The PWI-DWI mismatch region is defined as the difference in volume of tissue between the smaller diffusion lesion and the larger perfusion lesion. The mismatch provides an estimation of the extent of penumbra and can be used to monitor infarct growth over time. Ischemic penumbra is defined when there is at least a 20% discrepancy between the two volumes. It is observed in over 50% of middle cerebral artery territory stroke patients when studied up to 6 hours after the symptoms onset of stroke, and it may persist up to 24 hours. In the absence of reperfusion the DWI lesion typically expands into the PWI volume. This expansion is not only dependent on time, as it might be influenced by blood glucose concentrations, temperature, hematocrit, systemic hypoxia, and age.
However, using MRI in the acute stroke setting has some disadvantages. The time taken to acquire the data is longer than in conventional CT. Moreover, the procedure is not applicable in some patients because of claustrophobia or the presence of pacemakers or metal objects. There is also a small risk of nephrogenic systemic fibrosis in patients with severe renal impairment who receive gadolinium, but the use of contrast agents could be avoided in the future by the use of new techniques such as arterial spin labelling. Another limiting factor is the need for automated, online image analysis.
Although it has shown to be a good tool to assess acute ischemic stroke patients, there are some uncertainties regarding MRI to assess penumbral tissue. In animal models of ischemia and some clinical studies, it is possible to observe a very early reversibility of the DWI lesions. Therefore, the acute DWI lesion may not only represent tissue destined for infarct, but also severely ischemic tissue that may still be saved from necrosis. Even severely reduced ADC values, very early after stroke, are reversible in some patients. Also, DWI lesions can reappear after an initial disappearance following treatment. This is probably due to inflammatory tissue injury, delayed mitochondrial dysfunction, and apoptosis. Moreover, there can be false negatives of DWI in patients with ischemia in the posterior circulation.
On the other hand, PWI can detect areas of hypoperfusion that are not really penumbral tissue, but benign oligaemia. Thus, PWI techniques may overestimate the amount of tissue that is critically hypoperfused. This is important in penumbra assessment, as these areas of benign oligaemia will survive regardless of reperfusion. It is possible to reduce the volume of benign oligaemia, and therefore to improve the precision of PWI for estimating the volume of penumbral tissue in acute stroke patients and improve the accuracy of early prediction of final infarct volume, by using a threshold to exclude areas with minimal delay in contrast arrival times. The use of a strict Tmax threshold (>4 or >6 seconds) might reduce the percentage of patients considered to have a mismatch, but identifying mismatch patients who are more likely to have salvageable tissue. TTP maps are easiest to generate and to interpret visually. However, MTT or TTP can be increased even in areas without hypoperfusion if the perfusion pressure is reduced, as happens in autoregulation.
There are alternative methods to improve the definition of mismatch, such as a larger mismatch ratio than the typically selected 1.2. The original concept of PWI-DWI mismatch introduced an arbitrary ratio of 20%, but there is no evidence to support it. This volume ratio is very conservative and the increases are more modest because of the cubed root relation of radius with volume.
Despite these uncertainties, MRI remains the most practical tool for identifying tissue at risk of infarction in the early phase of acute ischemic stroke. Moreover, there are new MRI techniques than can help to avoid the uncertainties in infarct core and penumbra assessment. Arterial spin labelling, a non-invasive perfusion MRI technique, uses electromagnetically labelled arterial blood water to measure CBF. A quantitative estimate of the OEF can be obtained using MRI with the results in good agreement with values reported in the PET literature. By combining MRI-measured OEF and CBF via quantitative perfusion studies it might be possible to obtain a measure of the CMRO2 using MRI in acute stroke patients.
43.3.3 CT Perfusion
Conventional cranial CT is the most important imaging technique for acute stroke because of its easy availability and accessibility. It is helpful to discard intracranial hemorrhage and to detect the appearance and extent of early infarct signs. However, it gives little information about the presence and extension of ischemic penumbra. Although hypodensity on CT within the first 6 h may represent irreversibly infarcted tissue, tissue at further risk of damage cannot be clearly distinguished with conventional CT.
However, the combination of CT perfusion and CT angiography allows the evaluation of ischemic penumbra. The baseline CT perfusion should have 3 components: unenhanced CT, vertex-to-arch CT angiography (CTA), and dynamic first-pass CT perfusion.
CT perfusion involves the dynamic acquisition of sequential CT slices during the administration of contrast material and an analysis of time-concentration curves for each pixel. Brain tissue flow can be described by several parameters including CBF, CBV, and MTT. CBV is the total volume of flowing blood in a given volume in the brain, with units of millilitres of blood per 100 g of brain tissue. CBF is the volume of blood moving through a given volume of brain per unit time, with units of millilitres of blood per 100 grams of brain tissue per minute. MTT is the average transit time of blood through a given brain region, measured in seconds. In CT perfusion, the ischemic core is defined as the CBV lesion volume, i.e. the equivalent to the DWI lesion in MRI. The mismatch between the MTT or CBF lesion and the CBV lesion represents the ischemic penumbra.
Ischemic penumbra is defined by a CBF below 34% of the non affected hemisphere, with a CBV above 2.5 ml/100 g, and a MTT of be more than 145% of the contralateral side. The infarcted tissue is defined by a CBV below 2.5 ml/100 g.
The general principles underlying the computation of perfusion parameters such as CBV, CBF, and MTT are the same for both MR imaging and CT. The most important advantage of CT perfusion is the linear relationship between contrast concentration and attenuation in CT, which facilitates quantitative measurement of CBF and CBV. The main disadvantage of CT perfusion is the relative limited coverage, as most machines are only able to cover 4 brain slices, so the volumetric assessment of the core and penumbra might be incomplete. Nevertheless, newer CT equipment is able to cover the whole brain with a single bolus injection, similar to PWI-MRI.
The advantages of this technique are their wider availability, as it can be performed in any centre that has spiral CT. Also, the assessment is quicker than with MRI, and there are no concerns about claustrophobia, pacemakers, or metallic objects. Studies can immediately follow plain CT and be completed within about 5 minutes. However, the risk of contrast-induced nephropathy is more important than with MRI.
43.4 Clinical Relevance of Ischemic Penumbra: Using Penumbra as a Proof-of-concept
The aims of thrombolytic therapy are the recanalization of the occluded artery and salvage of the ischemic penumbra. Imaging penumbra might allow selection of patients for thrombolysis beyond 3 hours. Two phase II trials of thrombolysis with alteplase – the DEFUSE and the EPITHET trials – incorporated penumbral imaging and are worthy of further discussion.
The Diffusion and Perfusion Imaging Evaluation for Understanding Stroke Evolution (DEFUSE) trial was a prospective, open label, non randomized, multicenter trial aimed at confirming eligibility for intravenous thrombolysis with alteplase within 3 to 6 hours after onset. It included 74 patients, who received open-label alteplase and were analyzed using MRI and MRA within 3-6 hours of stroke onset and at 24 hours. The main hypothesis was that prespecified MRI profiles could be used to identify patients who have a robust clinical response to reperfusion when treated with alteplase 3-6 hours after stroke onset. The presence of mismatch was assessed if the PWI lesion was more than 20% larger than the DWI lesion. This study showed that it is safe to use MRI to define mismatch, and that thrombolytic treatment is effective in patients with mismatch. However, patients were not selected on the basis of MRI findings. In DEFUSE, early reperfusion in patients with a mismatch was associated with favourable outcome. These results were better after the exclusion of patients with malignant mismatch (i.e., a baseline DWI ≥100 ml, and/or PWI ≥100 ml with a Tmax ≥8 seconds). This positive effect of alteplase was not observed in patients without mismatch.
The Echoplanar Imaging Thrombolytic Evaluation Trial (EPITHET) was a phase II trial aimed at analyzing the effect of intravenous alteplase on ischemic lesion growth, reperfusion, and clinical outcome in patients with ischemic penumbra 3 to 6 hours after stroke onset. It was a randomized, double-blinded, placebo-controlled, multinational trial. Patients were treated with either alteplase or placebo, and were imaged with MRI before treatment and repeated at day 3-5. Mismatch was defined as a PWI/DWI volume >1.2 ml, and PWI-DWI volume ≥10 ml, and hypoperfusion volumes were defined using a Tmax delay of 2 seconds or more. MRI was not used to select patients, but to determine the effect of alteplase on lesion growth, reperfusion, and clinical outcomes. The primary endpoint was a greater attenuation of infarct growth in patients with an imaging mismatch who received alteplase than in those who received placebo. The study enrolled 101 patients, 52 allocated to alteplase and 49 allocated to placebo. The prevalence of mismatch was 86%, and arterial occlusion on MRA was seen in 62% of patients. The geometric mean growth of the ischemic lesion was about two-thirds in the alteplase group compared to the placebo group, although this difference was non significant. The incidence of reperfusion was significantly higher in patients with mismatch who received alteplase. Recanalization was associated with lower infarct growth and good neurological outcome in patients with mismatch. Although EPITHET was not powered to test differences in clinical outcomes, there was a better functional recovery at 3 months in the treatment group. The primary outcome measure of this study was negative, but other measures supported the hypothesis of attenuated infarct growth with alteplase beyond 3 hours.
Both studies provide strong biological support for the relation between infarct growth, reperfusion, and clinical outcome in the 3-6 hours time window after onset of stroke. However, these trials had some limitations. The analysis of the imaging data might have led to an underestimation of the true mismatch volumes, and neither study was powered to test the mismatch hypothesis.
There are other studies testing demosteplase, another thrombolytic agent which has some theoretical advantages over alteplase. In the DIAS trial there was a dose-response effect on reperfusion rates seen on PWI with greatest benefits with either 90 μg/kg or 125 μg/kg desmoteplase in the 3-9 hour time window. These results were replicated in the US Dose Escalation of Desmoteplase for Acute Ischemic Stroke (DEDAS) trial. With this encouraging data, the phase III DIAS-2 study, the largest penumbral selection trial with 186 patients, was undertaken. The primary outcome measure was clinical recovery at 3 moths. Good outcome was similar in the placebo and in the 90 μg/kg desmoteplase groups. However, patients who received 125 μg/kg desmoteplase had lower recovery and higher mortality. Most deaths in the latter group were non-neurological and occurred late in the trial, and were more likely due to chance.
There are other trials in acute ischemic stroke that have used the principle of penumbral selection (GAIN MRI substudy, Citicoline study, Normobaric oxygen study, COOL AID hypothermia). These are phase II trials that have tested the hypothesis that improving reperfusion or recanalization is safe, feasible, and might provide infarct growth attenuation on imaging. For example, in the GAIN trial, patients treated with gavestinel showed attenuation on infarct growth on DWI.
43.5 Future Analysis of Ischemic Penumbra
The DEFUSE and EPITHET studies have provided biological support for the therapeutic responsiveness of patients with mismatch. However, there is still no evidence that these patients would have better clinical outcomes when given alteplase. Therefore, new studies are needed to provide proof that mismatch tissue is a viable therapeutic target in human beings. Using larger sample sizes, such as combining the DEFUSE and EPITHET databases, could provide further indirect evidence.
The evidence given in the EPITHET study has encourage the investigators to plan a phase III trial (the EXTEND trial) which will randomize patients with mismatch detected by MRI to receive alteplase or placebo in the 4.5-9 hour time window.
Also, an ongoing trial, Magnetic Resonance and Recanalization of Stroke Clots Using Embolectomy (MR RESCUE) is assessing the benefits of reperfusion interventions, comparing patients with and without penumbral mismatch.
Other stroke groups are conducting observational studies assessing penumbra with CT perfusion to select patients. A conventional CT is used before starting treatment with alteplase, and after treatment a CT perfusion is performed to detect patients with a large mismatch or a persistent arterial occlusion. After that, selected patients receive rescue intra-arterial thrombolysis.
To conclude, we have robust data which suggests that it is possible to extend the therapeutic window of thrombolysis using the mismatch (i.e. the ischemic penumbra) as a proof of concept. However, more evidence is needed to use it in routine clinical practice. This information will become available in the next years, once the ongoing phase III trials are published.
Figure 43.2. Perfusion CT in acute stroke performed after intravenous treatment with alteplase. A-D: The CBV maps showed a moderate area of volume alteration in the deep territory of the left middle cerebral artery (MCA). E-H: The TTP maps disclosed a larger area of hypoperfusion that includes most of the left MCA territory. K-L: Angio-CT detects and occlusion of the left MCA in the M1 segment. This patient received rescue treatment with intra-arterial alteplase, and the conventional CT performed 3 days after stroke onset showed a moderate infarction in the deep territory of the MCA, closely resembling the initial CBV lesion. Thus, this case illustrates how neuroimaging assesses ischemic penumbra, and how thrombolytic treatment can limit lesion growth.
General References
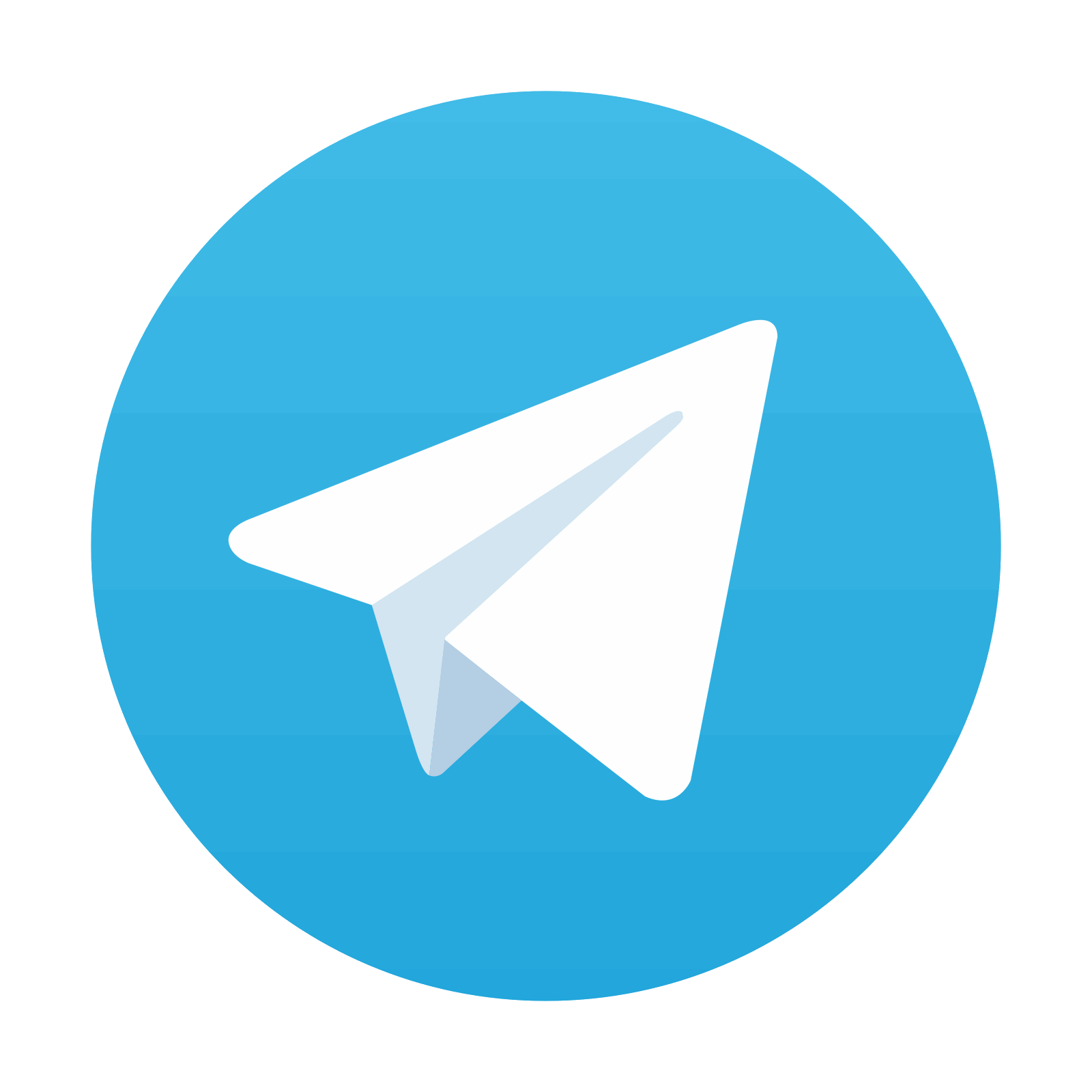
Stay updated, free articles. Join our Telegram channel

Full access? Get Clinical Tree
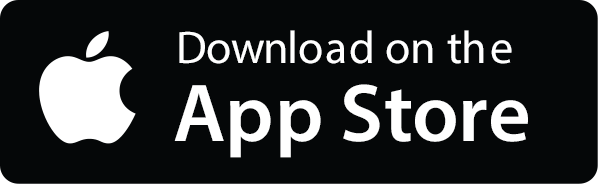
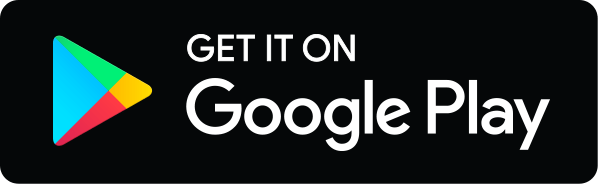