Keywords
Juvenile myasthenia gravis, acetylcholine receptor, thymectomy, neonatal myasthenia gravis, fetal acetylcholine receptor inactivation syndrome
Background
Juvenile myasthenia gravis is a major category of neuromuscular junction disorders that shares many pathophysiologic and clinical features with adult autoimmune myasthenia gravis, yet displays distinct demographic patterns, clinical features, and therapeutic challenges. These considerations underscore the need to discuss this disease separately from its adult counterpart.
Juvenile myasthenia gravis affects a broad range of ages within the pediatric population and has a worldwide geographic distribution. In North American and European populations, juvenile myasthenia gravis tends to be rare compared to adult myasthenia gravis, having been estimated to comprise 10% to 20% of myasthenia cases overall. The incidence of juvenile myasthenia gravis is estimated to be 0.9 to 2.0 new cases per million per year in Canada, and 1.5 new cases per million children per year in the United Kingdom. Although incidence and prevalence studies are rare, the disease appears to be more common in some parts of Asia. One series from China found that 50% of myasthenia gravis patients were less than 15 years old, while another series from Hong Kong found that 39.3% of myasthenia gravis cases were of juvenile onset. Gender ratios in juvenile myasthenia gravis are either balanced or show a slight female predominance prior to puberty, whereas affected females are much more common than males in adolescence, mimicking the gender ratio in adults.
Epidemiological data regarding neonatal myasthenia gravis are hard to come by and mild cases may not be recognized. Overall, it appears to be relatively rare and the incidence may be declining as maternal myasthenia gravis is better controlled. One study found a 30% incidence of neonatal myasthenia gravis among infants born to mothers with known cases of myasthenia gravis. Even rarer are the more severe variants: the fetal akinesia sequence and the fetal acetylcholine inactivation syndrome (see Chapter 7 ).
Pathophysiology
As in the adult form of the disease, juvenile myasthenia gravis is caused by antibodies attacking the postsynaptic membrane at the neuromuscular junction. Neonatal myasthenia gravis is somewhat different in etiology compared to juvenile myasthenia gravis, as the former is caused by transplacental transmission of maternal antibodies. Presynaptic autoimmune injuries typically cause a slightly different disease, Lambert-Eaton myasthenic syndrome, which has been reported in childhood but is exceedingly rare in this population. Genetic mutations that impair the function of the neuromuscular junction lead to congenital myasthenic syndrome (see Chapter 26 ).
The most common pathogenic antibody binds to the acetylcholine receptor (AChR), which is a key component of the synaptic signaling mechanism. Acetylcholine, the primary neurotransmitter at the neuromuscular junction, is released into the synapse in response to depolarization of the presynaptic motor nerve membrane with its accompanying influx of calcium ions. The acetylcholine diffuses rapidly across the synapse and binds to the acetylcholine receptors, triggering a depolarization of the postsynaptic membrane and a cascade of depolarizations that culminate in contraction of the muscle fiber ( Figure 27.1A ). All of this occurs in a blink of an eye.

The acetylcholine receptor is a pentameric complex that exists in two forms: α 2 βδγ in fetal and denervated muscle, and α 2 βδε in adult muscle. Most antibodies bind to the acetylcholine receptor and are detected by a radioimmunoprecipitation assay that is widely available. These antibodies bind mainly to the α and γ subunits, but since the γ subunit is not present in mature muscle, the less common antibodies to ε subunits can be important in some patients. These antibodies activate the complement cascade and can lead to destruction of the postsynaptic membrane and loss of the high density of acetylcholine receptors. The antibodies can also cause internalization of the acetylcholine receptors, without damage, because being divalent they can cross-link adjacent acetylcholine receptors via binding to the two acetylcholine α subunits; however, this process may not be very important in myasthenia gravis as it is accompanied, in experimental animals, by an increased synthesis of acetylcholine receptors. More rarely, blocking antibodies prevent the normal ion channel function of the acetylcholine receptors. The end result of any of these three mechanisms is impairment of neuromuscular transmission ( Figure 27.1B ), leading to myasthenia gravis (the original Greek term translates as “muscle weakness severe”). It should be noted that most antibody tests are designed to help diagnosis and not to make accurate and standardized measurements for evaluation of long-term treatment efficacy.
In the 15% of adult myasthenia gravis patients who do not have elevated acetylcholine receptor antibody titers, other antibody-mediated attacks on the neuromuscular junction are likely to cause the disease. The main known target is muscle specific kinase (MuSK). Antibodies to MuSK tend to be elevated in the absence of elevations of acetylcholine receptor antibodies. Myasthenia gravis associated with MuSK antibodies tends to be less common in northern Europe or Canada. MuSK antibody positive juvenile myasthenia gravis is rare, but has been reported on multiple occasions, in children as early as 14 months. Patients with MuSK antibody-associated myasthenia gravis often do not respond to pyridostigmine, so it is important to identify the immunological basis of individual cases whenever possible as a guide to potential treatments.
Titin and ryanodine receptors are intracellular muscle proteins and the antibodies that bind to them serve as biomarkers for the presence of thymoma in adults younger than 60 years. They are often, though not always, accompanied by elevations in acetylcholine receptor antibodies in adults. Children and adolescents with juvenile myasthenia gravis occasionally have isolated elevations of this antibody, but the association with thymoma appears to be reduced or absent in this age group based on a small number of cases. Antibodies to LRP4, another postsynaptic membrane protein, have also been reported recently in myasthenia gravis, but their frequency and clinical associations with the disease have not been characterized in detail to date, especially in children and adolescents.
Thymomas are found in some cases of juvenile myasthenia gravis, just as they are in the adult form of the disease, but are much less common in children and adolescents than in adults. This association with thymoma places myasthenia gravis within the broad category of paraneoplastic diseases. Histologic examinations of thymus tissue obtained from children with juvenile myasthenia gravis who have undergone thymectomy have found a high incidence of lymphoid follicular hyperplasia.
Some evidence suggests a link between Epstein Barr virus (EBV) and some autoimmune diseases. An early hint that this may be the case for myasthenia gravis was the discovery of the presence of Epstein Barr virus genome in thymus tissue derived from a few affected patients. A more recent study found evidence for active Epstein Barr virus infection in all 17 thymuses obtained from myasthenia gravis patients, compared to none of 6 control thymuses. However, 2 subsequent studies found no evidence for such an association, casting doubt on this theory. This proposed association remains controversial.
Clinical Presentation
Nearly all patients with juvenile myasthenia gravis demonstrate ocular involvement, principally the levator palpebrae and/or extraocular muscles, leading to ptosis, diplopia, and sometimes frank ophthalmoparesis or ophthalmoplegia. The ocular symptoms are often the first or primary manifestation of the disease at all ages. In rare cases, ocular symptoms are absent at presentation, but in those cases, bulbar or vocal symptoms are typically present, and ocular symptoms may develop later. Dysphagia, dysarthria, nasal speech, extremity weakness, and respiratory distress may occur in conjunction with the ocular symptoms. It is rare for myasthenia gravis to present with extremity fatigue in the absence of cranial nerve-innervated muscle involvement.
The bimodal anatomical distribution of involvement in most cases of myasthenia gravis has yielded its subcategorization into pure ocular and generalized variants. Both the ocular and generalized forms of myasthenia gravis may occur at any age. Ocular myasthenia gravis is more common in children than in adults and tends to occur more often in prepubertal children, whereas generalized myasthenia gravis is more common in postpubertal adolescents. Most patients who develop generalized myasthenia gravis display symptoms and signs of generalization soon after onset; thus, it is usually possible to assign individual patients to one of these anatomical subcategories early in the course of the disease. However, in rare cases, generalization may occur unexpectedly after years of pure ocular symptoms and/or remission from pure ocular involvement.
The physical examination of a child or adolescent with suspected myasthenia gravis is critical. Ptosis may be unilateral, bilateral, and asymmetric ( Figure 27.2 ), or bilateral and symmetric. When bilateral and symmetric, the ptosis may be difficult to discern on physical examination, as some children may appear to have mild ptosis at baseline. In such situations, it is helpful to examine any older photos of the child that are available for comparison. When unilateral or asymmetric, the ptosis is usually more obvious. One finding that can help verify the presence of abnormal ptosis is the curtain sign. To elicit the curtain sign, the examiner should lift each eyelid in turn. The affected side(s) will drop when the contralateral eyelid is raised. Ophthalmoparesis may be detected by lack of conjugate gaze in one or more directions, and is supported by the patient’s report of subjective diplopia in those directions ( Figure 27.2 ). Quantification of misalignments is most accurately performed by a neuro-ophthalmologist. Ptosis and/or ophthalmoparesis may become more obvious in response to a maneuver to fatigue the eyes, such as sustained upgaze for 1 minute. The ice pack test is used by some practitioners in the clinic or at the bedside; clinical signs of myasthenia gravis should improve in response to cold temperatures. Neck flexion strength is especially useful to assess while examining a patient with suspected myasthenia gravis; however, it is important to remember that children under the age of approximately 10 years tend to have apparent neck flexion weakness at baseline. While examining the extremities, some practitioners attempt to elicit fatigability in the deltoid muscle by asking the patient to abduct and adduct the arms repeatedly. When basic pulmonary function testing is available, measurement of vital capacity and/or negative inspiratory force is useful to assess respiratory function. When such equipment is not readily available, a good bedside assessment of vital capacity can be performed by asking the patient to take a deep breath and count numbers out loud as high as possible on one breath. Adolescents and adults should be able to count beyond 30 on one breath.

In all suspected cases of myasthenia gravis, serum thyroid studies are indicated, as symptoms of thyroid disorders may mimic those of myasthenia gravis, and thyroid disorders are sometimes present concurrently with myasthenia gravis.
A differential diagnosis should be considered in the appropriate settings. For pure ocular presentations, alternative diagnoses may include ptosis due to hypoplasia of the levator palpebrae muscles. The Marcus Gunn jaw winking phenomenon also occurs at times in children, and should be suspected when the ptosis occurs during chewing movements. When generalized symptoms are present, the possibility of Lambert-Eaton myasthenic syndrome should be considered, though this diagnosis is exceedingly rare in children compared to adults. Botulism may sometimes be a diagnostic consideration. A typical history for infant botulism includes living in an endemic geographic area (Pennsylvania, Utah, and California have high rates of infant botulism in the United States), a family member who works in the construction industry (spores are often stirred during construction projects), and constipation in addition to weakness. In older children and adolescents, rare cases of botulism may occur primarily as a result of ingesting botulinum toxin in improperly canned foods. Clues on physical examination that may indicate the presence of botulism include internal as well as external ophthalmoplegia (sluggish pupillary reactions in addition to eye movement abnormalities) and flaccid paralysis; tendon reflexes may be preserved. When generalized weakness appears to be chronic, the diagnosis of congenital myasthenic syndrome is a consideration (see Chapter 26 ), as well as other inherited disorders associated with ptosis including myotonic dystrophy (see Chapter 37 ) and congenital myopathies (see Chapter 28 ). Serum creatine kinase levels and electromyography may help sort out this differential diagnosis.
Diagnostic Testing
Juvenile myasthenia gravis, like adult myasthenia gravis, is ultimately a clinical diagnosis. A strongly suggestive history and physical examination may be sufficient to make the diagnosis; however, in a number of cases the clinical presentation is not clear-cut, and it is usually helpful to have positive results on one or more standard diagnostic tests. It is important to remember that some of these diagnostic tests do not always have high sensitivities, especially in cases of ocular myasthenia gravis.
Antibody Testing
Antibody testing is usually highly specific for myasthenia gravis. In the appropriate clinical setting, positive antibody titers confirm the diagnosis, and further testing may only be indicated if the clinical presentation is atypical. Antibody testing only involves serum testing and, especially in the case of acetylcholine receptor antibodies, is relatively inexpensive and straightforward. Thus, this test is easy to obtain in children. Some laboratories distinguish between binding, blocking, and modulating antibodies, but the binding antibody titer is usually sufficient and the other two are not universally available.
As in adults, children with generalized juvenile myasthenia gravis are more likely to have elevated acetylcholine receptor antibodies than children with pure ocular juvenile myasthenia gravis. Because the acetylcholine receptor antibody was the first and most common antibody to be associated with myasthenia gravis, patients who have elevated acetylcholine receptor antibody titers have traditionally been termed “seropositive,” with the remainder categorized as “seronegative,” even if they are found to have elevated titers of other antibodies. Seropositive adult patients tend to have more severe clinical courses, though the antibody titers do not correlate with clinical status or history of thymectomy. In pediatrics, seronegative myasthenia gravis is more common before puberty. In adults, a significant proportion of seronegative myasthenia patients have been shown to have elevated antibodies to muscle-specific kinase (MuSK), ranging from a majority in the first report to a lower proportion in other reports from the Netherlands, Thailand, and Sri Lanka. In the United States, MuSK antibody testing is expensive and elevated MuSK antibody titers are rare in seronegative juvenile myasthenia gravis, but can be identified in some generalized cases. A prepubertal child with MuSK antibody positive juvenile myasthenia gravis has been reported to present with ocular symptoms, only developing a brief period of generalized symptoms 2 years after onset. There are rare reports of patients, including an adolescent, found to have elevated titers of both acetylcholine receptor antibodies and MuSK antibodies. It is important to identify the occasional patients with elevations in both types of antibodies, as the acetylcholine receptor antibody elevation is typically low and less relevant than the elevation in the MuSK antibody.
Among the rare instances when striated muscle antibody titers are elevated in juvenile myasthenia gravis, they are sometimes associated with acetylcholine receptor antibody elevations and sometimes are found in isolation. When any antibody titer is borderline or mildly elevated, or if the clinical presentation is discordant with the antibody titers, repeat testing may help clarify the situation, though any intervening immunotherapies may reduce the sensitivity of further rounds of testing. Antibody titers do not generally correlate with the clinical status of affected individuals, though some reports suggest that a correlation may exist for MuSK antibody positive cases.
The Ice Test
The ice test is a traditional bedside test in which ice is placed on the eyes of a patient with ptosis who is suspected of having myasthenia gravis. A positive result is determined when cooling for approximately 2 minutes results in a 2 mm or greater improvement in the ptosis. The ice test has been determined in numerous adult studies to have a reasonably high sensitivity and specificity, but should not be relied on alone for diagnostic confirmation. The ice test has not been studied extensively in juvenile myasthenia gravis, but its use has been documented in children, and one study that included adults and children over the age of 9 years found that the ice test had reasonably good sensitivity but low specificity.
The Edrophonium Test
Edrophonium is a short-acting acetylcholinesterase inhibitor that is used only for diagnostic purposes; the half-life is so short that this medication has no therapeutic value. Tensilon is an old brand name for edrophonium, and the diagnostic test that makes use of this medication is often still called the Tensilon test, but given the multiple brands currently in use, it would be more accurate to call it the edrophonium test. The test is most useful when there is a clear physical sign such as unilateral ptosis that can be objectively observed for a response to this medication. It is best if this testing is performed in a monitored setting (i.e. with cardiac telemetry) where advanced cardiorespiratory support is available in case of bradycardia or asystole, which in rare instances occurs during this testing, especially when higher doses are used. An intravenous line should be placed, with a 3-way stopcock attached close to the catheter. One port should be attached to a syringe of normal saline flush, and the other to a syringe of edrophonium. At its typical 10 mg/mL concentration, edrophonium should either be drawn up in a 1 mL tuberculin syringe or diluted for use in a larger syringe. There is some conflict in the literature regarding the optimal dose of edrophonium in infants, with one report recommending 0.1 mg only, and another documenting 0.1 mg/kg. A pharmacokinetic study of edrophonium that included infant data suggests that 0.1 mg/kg is needed to counteract neuromuscular blockade. It may be reasonable to start with a dose of 0.1 mg as a test dose, and then administer 0.1 mg/kg (with a maximum of 0.5 mg) once or twice. For children older than a year who are below 35 kg in weight, the test dose should be 0.5 mg, followed by subsequent 1 mg doses several minutes apart to a maximum total dose of 5 mg. For children and adolescents who are above 35 kg, adult dosing ranges may be used, i.e. a test dose of 1 mg and subsequent doses of 1 to 2 mg each administered several minutes apart to a maximum total dose of 10 mg. The physical sign in question (e.g. ptosis, dramatic ophthalmoparesis, nasal speech) should be monitored before and after each dose to determine if there is a transient improvement. The heart rate should be tracked throughout, and dosing paused if there is a substantial dip. If bradycardia ensues, atropine is an effective antidote, and should be administered intravenously immediately. When the parents consent, photos taken before and after the test may be useful to document any possible response ( Figure 27.3 ). There are many instances when the results of this testing are equivocal, so the parents should be cautioned ahead of time that the findings may not yield a clear diagnosis.

Repetitive Stimulation
Repetitive stimulation testing is an adaptation of a motor nerve conduction study and is a useful diagnostic test, especially when the results of other diagnostic tests are negative, equivocal, or unavailable. As the term implies, the electromyography machine is programmed to deliver a series of electrical stimuli to a motor nerve at fixed intervals, and the compound motor action potential is recorded at a muscle innervated by that nerve. Common sites of stimulation include the ulnar nerve, spinal accessory nerve, and facial nerve, recording at the abductor digiti minimi, trapezius, and nasalis muscles, respectively, though any nerve and corresponding muscle that are amenable to motor nerve conduction studies may potentially be tested. Low frequency repetitive stimulation is administered at a frequency lower than 10 Hz, typically 2 or 3 Hz, whereas high frequency repetitive stimulation is administered at a higher frequency than 10 Hz, typically 20 to 50 Hz. The 10 Hz threshold corresponds to the 100 milliseconds that are needed after each stimulation for calcium ions to be effluxed from the nerve terminal to their original extracellular space, repolarizing the presynaptic membrane.
On low frequency repetitive stimulation, a decrement of 10% or greater in the compound motor action potential amplitude by the fourth stimulus is abnormal, indicating the presence of a defect in neuromuscular junction transmission. It is important to remember that decrements on low frequency stimulation cannot distinguish among different disorders of the neuromuscular junction. Low frequency testing is first obtained at baseline. When testing adults, it is customary to ask the patient to contract the muscle under study for a full minute, then perform further rounds of low frequency repetitive stimulation. In some instances, fatiguing the muscle in this manner brings out or accentuates the decrement. However, a study in adults indicates that post-exercise low frequency repetitive stimulation only produces an incremental increase in the diagnostic yield of this testing overall ; this finding, combined with the tenuous nature of children’s cooperation with these studies, suggests that post-exercise testing should be considered on a case-by-case basis in children and adolescents.
If a presynaptic disorder such as Lambert Eaton myasthenic syndrome or botulism is suspected, high frequency stimulation testing should be considered, as an increment in the compound motor action potential amplitude during prolonged high frequency stimulation indicates the presence of a presynaptic disorder. However, high frequency repetitive stimulation is painful for the child or adolescent, and needs to be performed with adequate analgesia, especially in younger children. Opioid analgesia is recommended, as milder analgesics such as acetaminophen and ibuprofen are inadequate, and topical lidocaine preparations are similarly ineffective under these circumstances. When adequate analgesia is not available, other clues on routine testing may suggest the presence of a presynaptic disorder. For example, a low baseline compound motor action potential amplitude with normal sensory nerve action potentials may be found in presynaptic disorders, as well as abnormal spontaneous activity and small, myopathic motor units on needle electromyography. This pattern of findings may also be consistent with a myopathic disorder; the results of low frequency repetitive stimulation would help distinguish between the two possibilities, though a low baseline compound motor action potential amplitude may make it difficult to interpret the repetitive stimulation testing in some patients.
As a general rule, repetitive stimulation at any frequency is more technique-dependent than routine motor nerve conduction studies, and artifactual findings are common, especially in some children who have difficulty holding still for this testing. Thus, it is important to attempt to replicate abnormalities in multiple nerves whenever possible before concluding that there is electrophysiologic evidence for a disorder of neuromuscular transmission.
Single Fiber Electromyography
Single fiber electromyography is a sophisticated adaptation of needle electromyography that determines the jitter for a set of individual muscle fibers within a motor unit. The jitter is the variability in the latency of neuromuscular transmission for individual muscle fibers; increased variability, or jitter, may be consistent with a range of neuromuscular disorders, including disorders of neuromuscular junction transmission. Single fiber electromyography is highly sensitive for myasthenia gravis, but like repetitive stimulation is not entirely specific for this disorder; thus, other evidence should be assembled in addition to abnormal jitter to make the diagnosis of myasthenia gravis. For single fiber electromyography to work, the patient needs to be cooperative and to be able to contract individual motor units voluntarily. The needle electrodes used are the same as for routine needle electromyography. Muscles that are commonly tested include the extensor digitorum brevis, frontalis, and orbicularis oculi. Traditional single fiber electromyography is not practical for most children, especially as it cannot be performed under sedation or general anesthesia, though some adolescents may be able to cooperate enough to produce a technically adequate study.
A variant of single fiber electromyography, stimulated single fiber electromyography, is much more useful in children and adolescents than traditional single fiber electromyography. In this technique, a monopolar needle electrode is inserted close to the nerve innervating the muscle under study, and the recording electrode may be a standard concentric bipolar needle inserted into the muscle itself. The monopolar needle acts as a stimulating electrode, and at low stimulus currents, activates individual motor units. Thus, aside from holding still during the study, this technique requires no further cooperation on the part of the patient, so stimulated single fiber electromyography may be performed in an awake or sedated patient.
Regardless of whether traditional single fiber or stimulated single fiber electromyography is employed, the data generated are similar. The motor unit potentials of a pair of individual muscle fibers within a motor unit are identified on the screen, and a set of these potentials (typically 100) are collected in rapid succession. The differences in latencies for the potentials representing the pair of muscle fibers is then averaged, yielding a measure of the variability of these differences, called the jitter. Increased jitter indicates the presence of a neuromuscular disorder, in some cases myasthenia gravis, and in some cases other diseases. Normal values for jitter on stimulated single fiber electromyography have been published.
In selected cases, when all or most of the diagnostic testing noted above is negative or unavailable, a therapeutic trial of pyridostigmine may be considered. However, the use of such a trial for diagnostic purposes may be unreliable, and it is important for expectations to be discussed clearly with the family in advance, and for the family to express an understanding of the side effects. One particular concern revolves around the possibility of a placebo effect. Thus, in assessing the response to such a trial, monitoring of objective physical examination findings should be performed whenever possible.
Imaging
An imaging study of the thorax to assess for thymoma is important to consider in cases of confirmed juvenile myasthenia gravis. Though rare in childhood and adolescence, thymomas have been reported on multiple occasions in this age group. Thorax imaging is critical for any child or adolescent with generalized juvenile myasthenia gravis. For ocular juvenile myasthenia gravis, thorax imaging should also be considered, although the yield is much lower in this situation. When choosing an imaging modality to use, three considerations are important. One is diagnostic yield. Both CT and MRI scans have good resolution and diagnostic yield, whereas chest X-rays are not sensitive enough to rely on alone. A second consideration is the need for sedation. MRIs may require sedation, especially in younger children, adding to its already considerable cost. CT scans typically do not require sedation, though exceptions do arise. A third consideration is radiation exposure. CT scans generate significant radiation exposure, whereas MRI scans do not generate any. The radiation exposure issue becomes especially important when a thymoma is identified and a patient will need serial imaging after a thymectomy to monitor for a recurrence of thymoma.
Medical Therapies
General Approach to Treating Juvenile Myasthenia Gravis
Medical therapies for juvenile myasthenia gravis include drugs that increase the availability of the acetylcholine neurotransmitter, as well as immunomodulatory and immunosuppressive drugs. Immunomodulation and immunosuppression are more powerful approaches to medical therapy. However, immunomodulation and immunosuppression are typically more invasive and/or carry greater side effect burdens. As in adults, pediatric patients and their families should be counseled regarding medications that may exacerbate myasthenia gravis.
Among the standard medical therapies for juvenile myasthenia gravis, it is reasonable to start with pyridostigmine except in the rare cases where a child presents in myasthenic crisis. But for those children who do not respond adequately to pyridostigmine, a complicated decision awaits the family and neurologist. Plasmapheresis, intravenous immunoglobulins, and corticosteroids are all efficacious in the treatment of myasthenia gravis, and this has been documented in the adult literature. Corticosteroids are easy to administer and are low in cost compared to plasmapheresis and intravenous immunoglobulins. However, chronic corticosteroid therapy is significantly more toxic in children and adolescents than in adults, as noted previously. It is thus worth considering plasmapheresis and/or intravenous immunoglobulins before corticosteroids.
Acetylcholinesterase Inhibition
Pyridostigmine is a synthetic small molecule (C 9 H 13 N 2 O 2 ) that was patented in 1951, and is often known by the brand name Mestinon. It is an acetylcholinesterase inhibitor, thus reducing the natural enzymatic metabolism of acetylcholine at the neuromuscular junction. The end effect is an increased amount of acetylcholine available to bind to postsynaptic receptors. Its potency is typically mild, especially in comparison with certain forms of immunomodulation. However, most patients tolerate the medication well, and some patients with mild symptoms may be adequately controlled on pyridostigmine monotherapy, so it is usually the first therapy used in juvenile myasthenia gravis. An exception to this approach involves MuSK antibody positive cases, which often do not respond well to this medication. Many patients experience no side effects at all. Some will develop diarrhea and less commonly other cholinergic side effects. Occasionally, somnolence will occur. A typical starting dose for children and adolescents is 0.5 to 1.0 mg/kg/dose, given 3 times a day, with a maximum starting dose of 60 mg/dose. The usual maximum for children and adolescents is 7 mg/kg/day, though some patients may need higher doses, such as confirmed cases of certain subtypes of congenital myasthenic syndrome. The 7 mg/kg/day maximum rarely needs to be exceeded in juvenile myasthenia gravis, and the risk of cholinergic side effects increases as the dose escalates. For adolescents and adults, some recommend a maximum absolute dose of 300 mg/day, though patients do exceed that level in certain cases.
Plasmapheresis
Plasmapheresis (also known as plasma exchange) is a therapeutic procedure in which whole blood is removed from the circulation, proteins such as antibodies and clotting factors are filtered from the plasma, and then the whole filtered blood is returned to the circulation. In most cases, the therapeutic targets are the antibodies rather than the clotting factors. It is effective in children and adolescents with juvenile myasthenia gravis, just as it is in adults with autoimmune myasthenia gravis. Traditionally, plasmapheresis has been used primarily to optimize a patient’s clinical status prior to thymectomy and also to treat myasthenic crisis. However, it can also be used as maintenance therapy for ambulatory children and adolescents, with one report indicating a more consistent response to this intervention compared to intravenous immunoglobulin. Some practical considerations in the implementation of this therapy are specific to children and adolescents. Chief among them is the issue of venous access. Some adolescents and older children may be able to accommodate the two large intravenous lines needed for peripheral plasmapheresis. However, many cannot, and thus the risks and benefits of having central venous access for this therapy will need to be considered and discussed with the families in those cases. Traditional plasmapheresis catheters and Broviac lines have double lumens and can easily accommodate plasmapheresis. Pheresis catheters are only meant to stay in for a brief period of time, whereas a Broviac may stay in longer. These lines carry a risk of infection, especially in situations where they remain in place after the patient is discharged (more likely with Broviac lines than pheresis catheters) and proper line care does not occur at home. The filtered antibodies are typically regenerated by the immune system in a matter of weeks, so plasmapheresis is either used in acute settings, such as myasthenic crisis, or repeated at regular intervals for those on maintenance therapy. Regimens vary widely depending on the individual patient’s response.
Intravenous Immunoglobulins
Intravenous immunoglobulin (IVIg) therapy consists of infusions of immunoglobulins pooled from multiple blood donors. Only standard venous access is required for these infusions, so most pediatric patients, including infants, are able to receive this treatment via peripheral venous access. IVIg has been reported to be effective in juvenile myasthenia gravis. In the past, allergic reactions could be severe and sometimes life-threatening, but the more severe reactions were found to be due to IgA deficiency; thus, many current IVIg formulations are depleted of IgA during processing. The most common side effects include headache, nausea, and vomiting. Some patients develop an aseptic meningitis, in which these common symptoms become more severe than usual, often persisting several days after treatment. As these meningitides resolve spontaneously with supportive treatment, it is usually not necessary to diagnose them with lumbar punctures, especially as the occasional post-lumbar puncture headache would only make matters worse. Rare cases of renal failure have been associated with IVIg therapy, but those have generally occurred in adult patients. The standard initial IVIg treatment regimen consists of 1 gram/kg infused intravenously×2 consecutive days. IVIg is eliminated from the circulation over a period of several weeks, with a half-life estimated to be 21 days. Thus, patients with chronic diseases such as myasthenia gravis may need a regular schedule of recurring treatments. Factors that may limit access to IVIg for certain patients include cost and the availability of sufficient numbers of blood donors. Shortages of IVIg have occurred in recent years, and the cost is extremely expensive, depending on the actual dose ordered.
Corticosteroids
Corticosteroids have traditionally played a central role in the treatment of autoimmune disorders in children, including juvenile myasthenia gravis. They are inexpensive, convenient to take in either oral or intravenous form, and have a long track record, so the side effect profile is well known. Corticosteroids are known to be effective in myasthenia gravis. The problem with their use revolves around the extensive list of side effects, including several that have significant long-term implications. The long-term toxicities are more consequential for children and adolescents than in adults, so steroids should be used more judiciously in the pediatric population than in adults. For example, the linear growth deceleration that occurs in some patients who are receiving chronic corticosteroid therapy is deeply disturbing to many patients and families, but is only relevant to children and adolescents who have not reached their full adult height. The weight gain and Cushingoid facies that may occur with corticosteroid therapy are troubling to both children and adults, but adolescents in particular who are often already sensitive about their weight are typically very worried about the prospect of embarking on a course of corticosteroids that may last months or even several years. The bone demineralization that occurs in many patients receiving corticosteroids is more consequential in children and adolescents as they are at an age when bone growth is typically increasing. There are numerous other side effects of corticosteroids that are well documented elsewhere, and are as concerning in children and adolescents as in adults.
Steroid-sparing Agents
Azathioprine is a commonly used steroid-sparing immunosuppressive agent for adults with myasthenia gravis. Its mechanism of action is inhibition of purine metabolism, resulting in impairment of DNA and RNA replication. The traditional indication for its use is to facilitate weaning of steroids. It has also been used in children and adolescents with juvenile myasthenia gravis, with good outcomes noted in combination with other therapies. Azathioprine is generally well tolerated, though side effects may include myelosuppression and some liver toxicity. There is a slight risk of malignancy reported, though this seems to be a negligible concern for relatively short courses of therapy. It has become increasingly recognized that certain variants of TPMT , the gene encoding thiopurine methyltransferase, can lead to deficient activity of that enzyme, leading to a higher risk of severe myelosuppression in patients with those variants who receive azathioprine. Thus, it is important to test patients, including children and adolescents, for thiopurine methyltransferase activity and/or genetic variants in the TPMT gene prior to initiation of azathioprine therapy so that dosing can be calibrated appropriately. Baseline and ongoing monitoring of blood counts and liver function tests should also be routine in patients receiving azathioprine.
Cyclosporine is another steroid-sparing immunosuppressant medication that is a standard option for the treatment of adults with myasthenia gravis, though it is not used frequently in some countries such as the United Kingdom. It inhibits T-cell activation by dampening the production and release of interleukin 2. Its track record in juvenile myasthenia gravis is somewhat limited, but it has been used in that setting as well.
Tacrolimus is a macrolide that inhibits T-cell activation by blocking the dephosphorylation of nuclear factor of activated T cells (NF-AT). It is another immunosuppressive medication that has the potential to help wean steroids in the setting of myasthenia gravis. Tacrolimus seems to be beneficial in some cases of adult myasthenia gravis, and it is also used for various indications in children, but not much is known about its potential as a therapy for juvenile myasthenia gravis. One recent case report suggests that it may be helpful in some children with ocular juvenile myasthenia gravis.
Mycophenolate mofetil inhibits purine synthesis and is another steroid-sparing immunosuppressant that is used for the treatment of myasthenia gravis. Its history in this setting is brief but somewhat turbulent. Mycophenolate mofetil began to be used for myasthenia gravis based on case reports, small open label studies, and retrospective case series suggesting that it was quite effective in some adults and adolescents. However, when two major randomized, controlled trials were performed, they showed no statistically significant treatment effect, causing confusion among many neurologists and patients. It is possible that these trials did not show a clear treatment effect due to the concomitant use of steroids in both arms of the studies. A subsequent retrospective study detected another possible explanation for the discrepancy, finding that the benefits of mycophenolate mofetil may only begin to emerge in a quantifiable manner after 6 months. Mycophenolate mofetil continues to be used in some affected children and adolescents based on retrospective data, but has not been systematically studied for this age group.
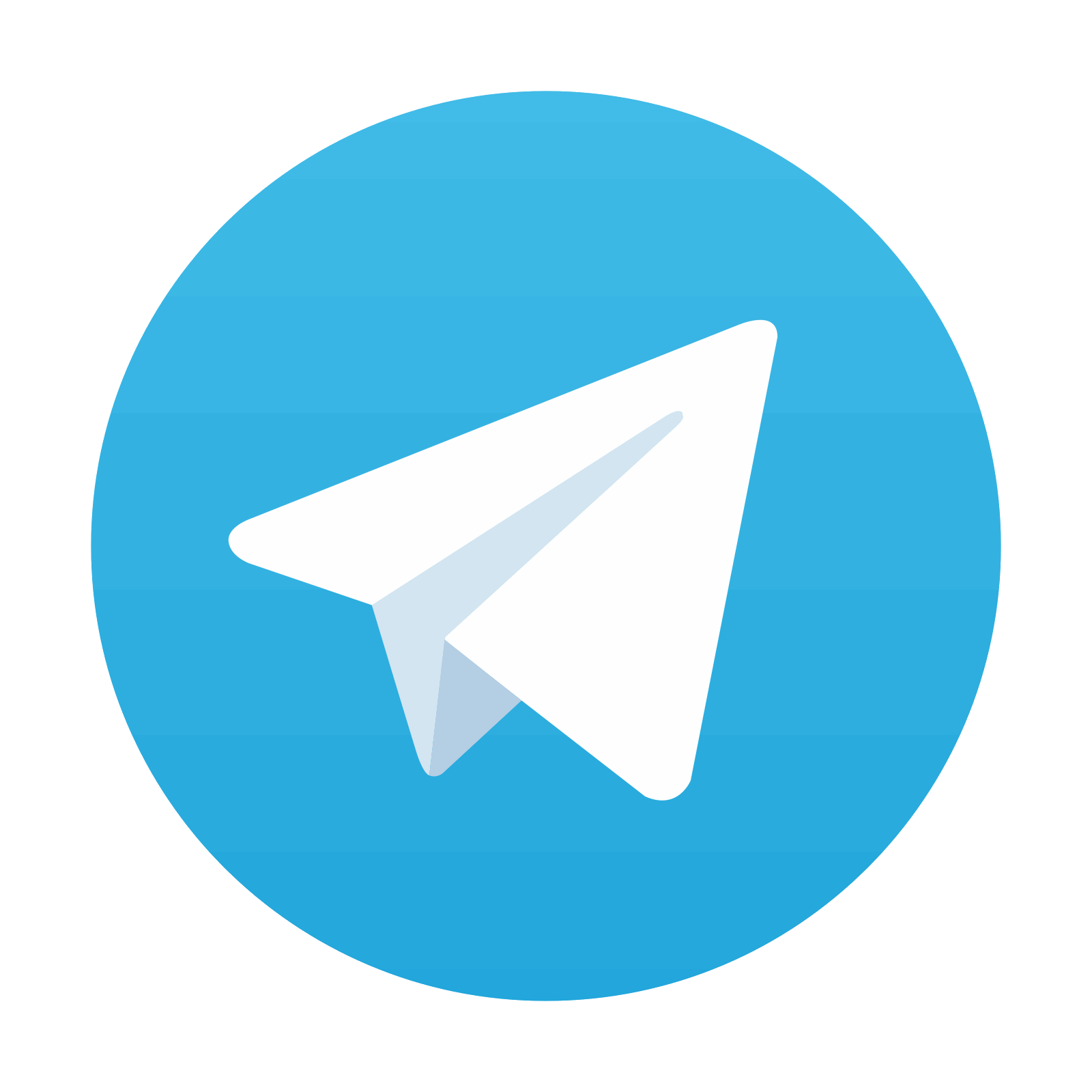
Stay updated, free articles. Join our Telegram channel

Full access? Get Clinical Tree
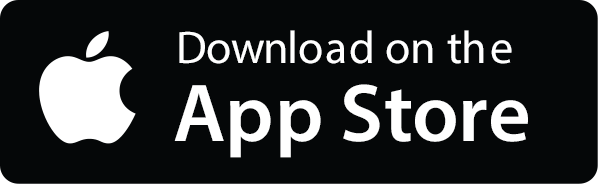
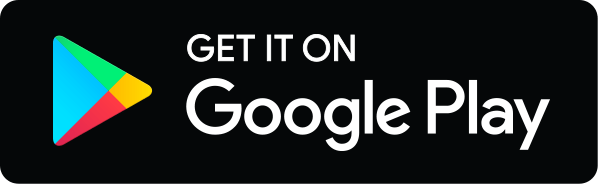