Syndrome
Affected gene
Prevalence in PanNETs (%)
Multiple endocrine neoplasia type 1
MEN1
20–70
Von Hippel-Lindau
VHL
<20
Neurofibromatosis type 1
NF1
<10
Tuberous sclerosis complex
TSC2
Rare (~1)
MEN1 is a rare autosomal dominant disorder characterized by a greatly elevated risk of a variety of endocrine tumors involving the pituitary, parathyroid, endocrine pancreas, and duodenum. Hereditary PanNETs occur in 20–70 % of MEN1-affected patients [1], and they are frequently small, nonfunctioning, multiple, and benign. Moreover, they have an earlier age of onset and a much higher rate of postoperative recurrences compared with their sporadic counterparts. MEN1 patients typically harbor multiple small pancreatic neuroendocrine microadenomas, thought to be precursor lesions to malignant PanNETs. A genetic locus associated with MEN1 syndrome was localized to the long arm of chromosome 11 (11q13), and the gene responsible (MEN1) was identified by positional cloning techniques [2, 3]. MEN1 spans 9.8 kb with ten exons and encodes a 610-amino-acid protein named menin [4] which is a ubiquitously expressed nuclear protein. Heterozygous germline mutations scattered throughout the MEN1 coding region have been identified in over 80 % of probands/families with patients sharing at least three major lesions of the syndrome and first-degree relatives affected by one (or more) MEN1-related lesions. More than 1,300 mutations in MEN1 have been identified to date, with the majority of mutations resulting in the loss of function of the protein [5].
Menin is critical to normal pancreatic β cell homeostasis and glucose sensing. High glucose levels activate PI3K/Akt, through phosphorylation of the transcription factor FoxO1 [6, 7]. FoxO1 has decreased binding affinity to the MEN1 promoter, resulting in inhibition of menin expression and enhanced proliferation. Homozygous deletion of the MEN1 gene is embryonic lethal [8]. Mice with heterozygous deletion of the MEN1 gene develop multiple endocrine tumors, reminiscent of the human syndrome [9–11]. Moreover, menin promotes the expression of cell-cycle inhibitors being an essential component of the mixed lineage leukemia (MLL) histone methyl transferase complex that regulates chromatin remodeling [12, 13]. In normal pancreatic islet cells, the tumor suppressor activity of menin is due to the inhibition of proliferation, through H3K4 methylation at promoters of CDKN2C and CDKN1B [14, 15]. In addition, menin may also be involved in the response to DNA damage. Genome-wide chromatin studies have found menin to be associated with promoter and coding regions of hundreds of genes [16, 17]. In particular it associates with the 5′ regions of genes whose protein products are involved in DNA repair, implicating menin in the DNA damage response and therefore genomic stability [18, 19]. Taken together, these data suggest that loss of menin causes deregulation of normal cell growth control in conjunction with increased genomic instability.
Von Hippel-Lindau syndrome is the second autosomal dominant predisposition syndrome featuring an elevated rate of NETs [20]. VHL-related pancreatic tumors are mostly exocrine microcystic adenomas, but 5–17 % of patients with VHL could be affected by PanNETs [21–23]. These tumors have typical morphological characteristics, consisting of solid, trabecular, or glandular structures composed in about 60 % of cases of clear cells with vacuolated lipid-rich cytoplasm [24, 25]. Most VHL PanNETs are multiple and nonfunctioning, and 30–40 % of them demonstrate focal positivity for pancreatic polypeptide, somatostatin, glucagon, and/or insulin [24]. Like VHL-associated renal tumors and retinal and/or cerebellar neoplasms, pancreatic islet-cell tumors are markedly vascular. Most of these tumors are slow-growing and asymptomatic, but some cases can grow rapidly or metastasize. Despite the variety of tumor types observed clinically in this disorder, progression to malignancy in VHL disease is associated primarily with the development of renal carcinomas and PanNETs [24, 25]. VHL syndrome results from germline mutations in the VHL gene, a tumor suppressor gene located at 3p25 with three coding exons [23]. That has been shown to be involved in multiple functions including ubiquitination and the regulation of angiogenesis, as well as a gatekeeper function in the G0/G1 checkpoint. A crucial function of VHL protein is the oxygen-regulated degradation of hypoxia-inducible factor (HIF) alpha, a transcription factor that regulates gene expression in response to low-oxygen conditions [26, 27]. Lack of degradation of this factor, due to the absence of the VHL protein, results in the uncontrolled production of factors promoting the formation of blood vessels such as vascular endothelial growth factor implicated in tumor development [28–31]. Germline VHL mutations have been characterized in more than 500 patients and have provided a wealth of data for genotype-phenotype correlations [32–34]. Among patients with VHL syndrome, about 40 % of mutations are genomic deletions and the rest are predominantly truncating or missense mutations [35]. VHL-associated tumors show somatic alteration of the remaining wild-type allele by allelic loss or, more rarely, by promoter hypermethylation [36]. Molecular profiling of VHL PanNETs compared to sporadic PanNETs has recently demonstrated that VHL PanNETs have specific genetic alterations; most striking are those related to angiogenesis and HIF signaling [37].
Neurofibromatosis type 1 and tuberous sclerosis are the two other inherited autosomal syndromes that are associated, albeit infrequently, with increased susceptibility to PanNETs (Table 20.1). Both NF1 and TS are disorders of unregulated progression through the cell cycle, in which causative genes behave as characteristic tumor suppressor genes. The pathogenesis of these familial syndromes is linked by the shared regulation of a common pathway, the protein kinase mammalian target of rapamycin (mTOR).
NF1 is caused by a germline mutation of the NF1 gene at 17q11.2, which encodes the protein neurofibromin. Half of the mutations in the NF1 gene occur de novo. Loss of function mutations in NF1 therefore cause increased activation of ras and increased signaling via downstream pathways, including the mitogen-activated protein kinase (MAPK) pathway and mTOR pathway. Gastroenteropancreatic NETs that develop in the setting of NF1 include duodenal somatostatin-cell tumors and insulinomas (in up to 10 % of patients) [38].
TS is caused by mutations in one of two different genes: the TSC1 gene located at 9q34 and the TSC2 gene located at 16p13.3, which encode for the proteins hamartin and tuberin, respectively [39, 40]. The development of PanNETs in this syndrome is extremely rare, with only nine cases of TS-associated PanNETs reported in the literature [41]. The hamartin-tuberin complex functions as an inhibitor of downstream cellular signaling via mTOR pathway, and the development of tumors in patients with TS is the result of dysregulated signaling of mTOR to downstream targets [42].
20.2 Sporadic Pancreatic Neuroendocrine Tumors
Before the PanNETs exome was sequenced, much of the knowledge about the genetics of PanNETs arose from the studies of the abovementioned hereditary syndromes and by applying these discoveries to sporadic lesions.
Somatic mutations of the MEN1 gene have been found in roughly 30 % of sporadic PanNETs with frequent deletion of the wild-type allele in these cases [43–51]. Allelic deletion on 11q is frequently found in sporadic PanNETs, and by combining data from all the studies referred to, it appears that the LOH rate is usually two to three times higher than the frequency of mutations of the MEN1 gene. This suggests that LOH at 11q13 per se is not an indicator of MEN1 mutations. Such findings can be explained by other mechanisms of MEN1 gene inactivation, such as methylation of the promoter or the presence of mutations in unexamined noncoding regions. It also seems likely that the higher frequency of allelic deletions is an indicator for the inactivation of another tumor suppressor gene on 11q.
Compared to the MEN1 gene, the VHL gene is only rarely mutated in sporadic PanNETs, mainly in a small proportion of nonfunctioning PanNETs [52]. Moreover, the VHL gene may be inactivated by alternative means, such as promoter hypermethylation or deletion, as has been reported for up to 25 % of PanNETs [53]. Allelic deletion on 3p was frequently found in sporadic PanNETs and a positive correlation between 3p loss and malignant tumor behavior was observed by different authors [54, 55]. Chung et al. [54] and Barghorn et al. [55] identified the smallest common region of allelic loss between 3p25.3-p25.1 and 3p23, suggesting the presence of another tumor suppressor gene centromeric to the VHL gene. Barghorn et al. [55] observed that most nonfunctioning tumors lost the entire chromosome 3 during progression to the metastatic phenotype, while functioning PanNETs lost only parts of chromosome 3p and rarely the entire chromosome 3p. Since the pattern of allelic deletions at 3p differs among PanNET subtypes and microsatellite markers at 3p25.3-p23 may have already been lost at an early tumor stage, caution was suggested with respect to the use of 3p markers in distinguishing clinically benign from potentially malignant PanNETs.
Finally, while no NF1 mutations have been reported in sporadic PanNETs, recent evidence demonstrates that the TSC2 gene may have a role in the development of these tumors. Thus, Missaglia et al. found TSC2 expression was downregulated in a majority of sporadic PanNETs [56], and more recently Jiao et al. found the TSC2 gene to be mutated in 8.8 % of 68 sporadic PanNETs [57]. In this study, the complete exome of ten primary PanNETs was sequenced, followed by screening for mutations in the most commonly altered genes in an additional 58 tumors. As anticipated from prior studies, MEN1 was found to be the most frequently mutated gene in PanNETs, as it was altered in 44 % of the tumors. In addition, genes in the mTOR signaling pathway were also mutated in about 16 % of PanNETs, with inactivating mutations in TSC2 and PTEN genes, plus a single case showing an activating mutation in PIK3CA (Table 20.2). The most striking result from the exome sequencing was the identification of recurrent somatic mutations in two genes that had previously not been associated with cancer, namely, DAXX (death domain-associated protein; located on 6p21.3) and ATRX (alpha-thalassemia/mental retardation syndrome; located on Xq21.1). Intriguingly, mutations in ATRX or DAXX were mutually exclusive, occurring in a total of 43 % of PanNETs examined. Most of ATRX and DAXX mutations were of an inactivating type, and there was a significant positive correlation between gene mutation and loss of the nuclear protein, as assessed by immunostaining. Both of the proteins encoded by these genes participate in heterochromatin maintenance at telomeres, particularly in the incorporation of the histone variant H3.3 at the chromosome ends [58, 59]. Interestingly, tumors with loss of ATRX or DAXX proteins show the presence of a telomerase-independent telomere maintenance mechanism known as alternative lengthening of telomeres (ALT) [60]. ALT occurs in approximately 5 % of cancers [61] and is considered an alternative telomerase-independent telomere maintenance mechanism [62]. Recently, de Wilde and colleagues demonstrated that ATRX and DAXX mutations are relatively late events in PanNET tumorigenesis being associated both with higher grade (G2 versus G1) and with larger tumor size. Remarkably, patients with PanNETs exhibiting altered ATRX or DAXX genes have a significantly longer survival rate than patients with wild-type tumors [63]. In addition to gene mutation analyses, numerous cytogenetic and molecular studies have been performed to detect recurrent genetic changes in PanNETs. The primary techniques used in these works have been loss of heterozygosity (LOH) analysis to identify genetic losses and comparative genome hybridization (CGH), including classical CGH and array-based CGH (aCGH), which provides information on both gains and losses of genetic material. Although these studies are diverse, featuring different (frequently small) tumor cohorts and a variety of techniques, some patterns emerge from the overall results. One frequent observation is that the quantity of genetic abnormalities is often positively correlated with indicators of tumor aggressive behavior [64–70]. Overall, these results indicate that neuroendocrine tumor progression is likely to be driven by ongoing chromosomal instability (CIN) leading to the accumulation of multiple genetic aberrations, with increasing numbers of alterations associated with more aggressive biological behavior and consequently worse patient prognosis. The alterations described are not randomly distributed on chromosomes but are particularly common in distinct chromosomal regions. In PanNETs, losses are common at chromosomes 1p, 1q, 3p, 6q, 10q, 11p, 11q, 22q, and Y, while frequent gains are observed on 7p, 7q, 9p, 9q, 14, 17q, and 20q [47, 54, 65, 66, 68, 69, 71–78]. Some differences have been reported among PanNETs considering both type and number of DNA alterations. Insulinomas exhibit a lower number of genomic alterations than other PanNETs and they frequently show gain of 9q32 and loss of 22q13.1, which appear to be early genetic events in these tumors [79]. By contrast, they rarely show 3p and 6q losses associated with malignancy [55, 77]. Malignant insulinomas, in contrast, harbor a large number of chromosomal alterations similar to those seen in other types of malignant PanNETs [79]. In pancreatic gastrinomas, only limited chromosomal imbalances are encountered. Losses at 3p and 18q21 occur in approximately 33 % and 22 % of cases, respectively [66, 74]. Nonfunctioning PanNETs (NF-PanNETs) in general harbor higher numbers of chromosomal gains and losses than functioning tumors [72].
Table 20.2
Genetic mutations in sporadic PanNETs
Gene | Protein function | Mutation frequency (%) |
---|---|---|
MEN1 | Histone remodeling | 44 |
DAXX | Chromatin assembly | 25 |
ATRX | Chromatin assembly | 18 |
TSC2 | GTPase-activating protein | 9 |
PTEN | Protein tyrosine phosphatase | 7 |
PIK3CA | Phosphoinositide 3-kinase | 2 |
TP53 | Cell-cycle arrest | 5 |
To date very few studies have been published about DNA methylation changes in PanNETs, and there is scant information about the role of gene promoter-specific hypermethylation as well as global hypomethylation in the development and progression of these tumors. Using global analysis of the methylation status of LINE-1 and Alu repeat sequences that are widely distributed throughout the genome, it was found that these sequences were hypomethylated in the vast majority of cases, when compared to adjacent normal tissue and, when present, hypomethylation correlated with tumor aggressive behavior [80–82]. In addition, epigenetic silencing through promoter hypermethylation has also been assessed. Several specific CpG-island-associated genes are frequently methylated in PanNETs, namely, RASSF1A, CDKN2A, HIC1, RARß, APC, MGMT, and ER [83–88]. The cumulative methylation frequency ranges from only a few percent to more than 70 % for some of these genes. Among the genes examined, the methylation status of MGMT is of potential clinical importance because it may help in predicting the clinical efficacy of alkylating agents in these tumors [89, 90]. House et al. found that the MGMT promoter was methylated in 40 % of the 48 PanNETs examined, thus potentially explaining the decreased protein expression observed in up to 50 % of these tumors by other studies [86, 90]. The methylation status of the MGMT gene is of possible clinical importance because expression of this DNA repair enzyme is associated with poor clinical responses to alkylating agents [91]. The RASSF1A tumor suppressor gene, located on 3p21, is reportedly methylated in 75–83 % of lesions, where methylation is correlated with reduced RASSF1A mRNA expression, increased tumor size, and the presence of metastases [85, 87]. Although several authors suggested that RASSF1A methylation may provide prognostic information in PanNETs, there is evidence that this epigenetic marker is also observed in a significant fraction of tumor-adjacent normal pancreas tissue [92] and further studies are required to clarify this point. The CDKN2A gene codes for the p16 tumor suppressor protein, a member of the Rb cell-cycle regulatory pathway that modulates the arrest of the cell cycle at G1-S. Muscarella et al. [93] reported the presence of CDKN2A promoter hypermethylation or homozygous deletion in a limited number of pancreatic gastrinomas and in nonfunctioning PanNETs. These data were confirmed by Lubomierski et al. [94] who reported loss of expression of at least one of the tumor suppressor genes CDKN2A/p16, CDKN2B/p15, and CDKN2D/p14 localized as a gene cluster at 9p21. mRNA transcripts of these genes were lost more frequently in nonfunctioning PanNETs (57 %) than in insulinomas (30 %) and gastrinomas (22 %). Unlike RASSF1A, methylation of the CDKN2A in PanNETs displayed good specificity for tumor tissue versus adjacent normal tissue.
Overall, many DNA methylation changes have already been uncovered in these initial studies. With the increasing availability of whole genome methods, this list will undoubtedly grow in the near future, with the hope that some of these methylation marks will be useful as diagnostic and prognostic biomarkers.
20.3 Pancreatic Neuroendocrine Carcinomas
PanNECs are poorly differentiated neuroendocrine tumors of the pancreas, characterized by a very aggressive behavior and a dismal prognosis. These tumors are much rarer than PanNETs and at present there are very few reports on their genetic and molecular profiles [95–98].
In contrast to PanNETs, they are characterized by a high proliferation rate and this has allowed standard karyotypic analyses of metaphase chromosomes of PanNECs to be performed [95]. Specifically, Welborn at al. published karyotypic profiles of two PanNECs, and they concluded that they probably represent a genetically distinct entity compared with PanNETs, because of the very different spectra of chromosomal abnormalities observed in the two subsets of tumors. Strong support for this hypothesis comes from recent sequencing analysis of PanNECs [96]. In this study, Yachida et al. performed targeted exomic sequencing and immunohistochemical analyses, comparing high-grade PanNECs (both small cell and large cell subtypes) with PanNETs. Their results showed that small cell and large cell NECs are genetically related entities, because mutations in RB1 and TP53 were very common in these tumors, while they were completely absent in PanNETs. Aberrant p53 and Rb staining occurred exclusively in PanNECs at a rate of 95 % and 74 %, respectively. Interestingly, aberrant p16 staining was not exclusive to PanNECs, but also occurred in a mutually exclusive manner with respect to Rb such that all PanNECs had disruption of the p16/Rb pathway. Related to this finding, inactivating mutations in DAXX and ATRX were exclusively found in PanNETs while they were completely absent in PanNECs. Moreover, BCL-2 overexpression was a more common feature of PanNECs (74 %) versus PanNETs (18 %).
Finally, the potential genetic relationship between PanNECs and pancreatic ductal carcinomas (PDACs) has been considered [99]. At present, available data demonstrates that their mutational spectra are significantly different, although PanNECs and PDACs share a high frequency of TP53 alterations. Indeed, the genetic changes frequently observed in ductal adenocarcinomas, such as KRAS mutations and loss of SMAD4/DPC4, were observed infrequently in PanNECs [96]. These results seem to suggest that most panNECs do not arise from preexisting ductal lesions and that the possibility that some PDACs may coexist with panNECs may be a relatively uncommon occurrence [98]. Only in MANECs (mixed adenoneuroendocrine carcinomas) a close genetic relationship between the exocrine and the endocrine components has been demonstrated, suggesting a monoclonal origin of these rare cancers in all the gastroenteropancreatic tract [98, 100].
References
1.
Jensen RT, Berna MJ, Bingham DB et al (2008) Inherited pancreatic endocrine tumor syndromes: advances in molecular pathogenesis, diagnosis, management, and controversies. Cancer 113:1807–1843PubMedCentralPubMedCrossRef
2.
3.
4.
Wu X, Hua X (2008) Menin, histone h3 methyltransferase, and regulation of cell proliferation: current knowledge and perspective. Curr Mol Med 8:805–815PubMedCentralPubMedCrossRef
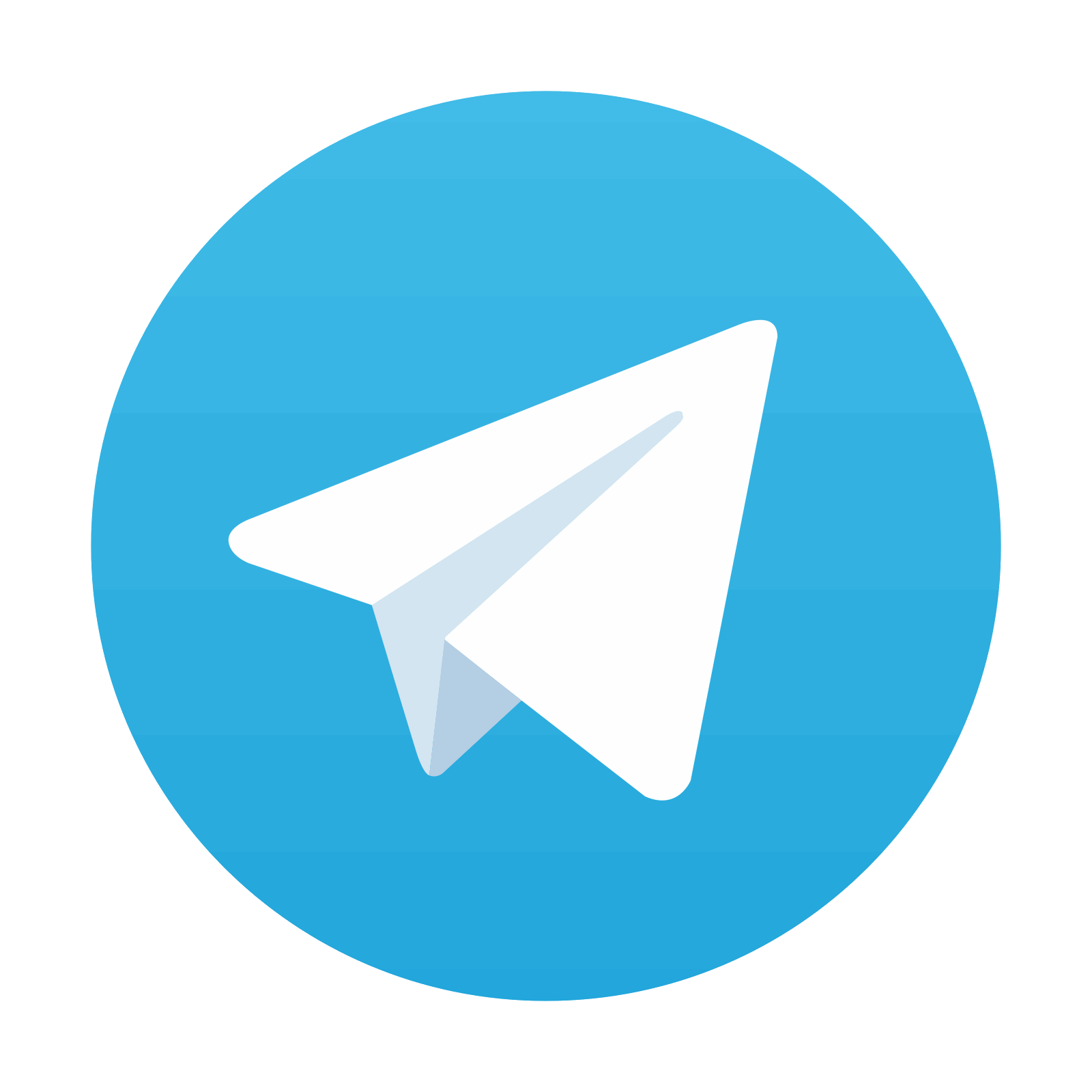
Stay updated, free articles. Join our Telegram channel

Full access? Get Clinical Tree
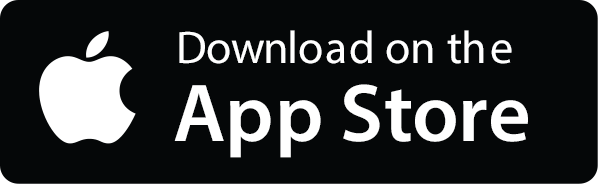
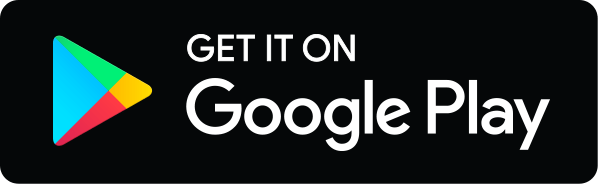