Monitoring EEG during Carotid Surgery
Thoru Yamada
Malcolm Yeh
INTRODUCTION
The studies from the North American Symptomatic Carotid Endarterectomy Trial Collaborators (1) and the European Carotid Surgery Trialists’ Collaboration Group (2) have shown the benefit of carotid endarterectomy (CEA) to prevent stroke, especially for patients with high-grade carotid artery stenosis. A more recent study has reaffirmed the effectiveness of CEA, especially in symptomatic patients with severe carotid stenosis (>70%) (3). Patients with asymptomatic stenosis in the 50% to 60% range are in general uncertain candidates for CEA, but may well benefit, especially for patients with additional medical conditions having a higher stroke risk. The CEA benefit is significantly less for asymptomatic patients (4,5), but CEA may still be applicable for asymptomatic patients with a high degree of stenosis (2), plaque with ulceration (6), and an occluded contralateral carotid artery (7).
Because CEA always carries a risk of morbidity and mortality (allowable rates of stroke and death rate of less than 6%), CEA for asymptomatic patients should only be considered if surgery could be done by an expert surgeon with track record of low complication rates of less than 3%. CEAs are generally not indicated for patients with less than 50% symptomatic or less than 60% asymptomatic stenosis (8).
CEA can be performed under general or regional anesthesia (RA). During CEA, the internal carotid artery must be cross-clamped; therefore, the brain becomes subject to ischemia or hypoperfusion. The brain can tolerate more than 1 hour if cerebral blood flow is greater than 15 mL/100 g/min, but the severe infarct would occur within 4 minutes with zero blood flow. A number of monitoring techniques are available for examining the cerebral perfusion and detecting the risk of cerebral ischemia. These include EEG, somatosensory-evoked potential (SSEP) (median nerve stimulation), transcranial Doppler, and oxygen saturation of hemoglobin using near-infrared spectroscopy, stump-pressure measurement, and cerebral blood flow by Xenon-133. In order to protect brain from ischemic damage during cross-clamping, placement of a catheter to shunt blood around the surgical site has been used. Because the shunt placement itself carries a significant risk of embolization as a result of dislodging atherosclerotic emboli coming from the vessel wall, only patients with a potentially high risk of developing ischemic brain damage are selected for shunt placement. Determining which patients require shunts depend on the multiple factors. EEG has been the most commonly used monitoring method for shunt determination but other tests are complimentary or may be more sensitive and accurate for predicting ischemic insult in some cases.
Whether to use a shunt has long been argued by many investigators. Some have proposed the use of shunt in all CEAs (9) while others have stressed the increase of stroke incidence with the use of shunt and have argued that the shunt should not be used at all (10,11). Most CEAs, however, have been done with selective shunting based on EEG or other monitoring methods. Irrespective of the shunt used routinely, selectively or not at all, the incidences of perioperative stroke were overall similar, <3% (1). In three groups of studies, however, the incidence of stroke was highest among those using a shunt routinely and lowest among those never using a shunt. These data suggest that intraoperative or postoperative stroke is more likely due to emboli rather than hypoperfusion or ischemia and thus raises a question of the value to shunting and monitoring (12). Indeed in a study using RA (patients were awake during the CEA), only one of 21 neurologic deficits (a total of 345 patients) was attributable to cerebral anoxia and remaining 20 patients were due to thrombo-embolic origin (13). Another large scale of review study (3062 CEAs) showed that intraoperative ischemia, thrombosis, embolization, and intracranial hemorrhage accounted for 47 of the 63 deficits (14). Of these, thrombo-embolic events were the most common cause of neurologic deficit accounting for 45%. Nonetheless a substantial number of patients would suffer from ischemic stroke unless appropriate protection is provided. Moreover, in some cases, postoperative stroke could be extensive resulting in global cerebral dysfunction. This is where various monitoring methods can be used to warn of an impending ischemic event. One study comparing selective shunting based on EEG (311 patients) and a nonshunt group (591 patients) in a single institute reported much higher incidence of postoperative stroke in the nonshunt group (13 patients) as compared to a selective shunt group (1 patient) (15).
ANESTHESIA
Commonly used anesthesia for CEA surgery is gaseous anesthesia with isoflurane ranging between 0.4% and 0.6% with 40% to 60% N2O and oxygen. This combination may be supplemented by sufentanil or remifentanil (16,17). An alternative anesthetic regimen consists of nitrous oxide in oxygen and propofol after induction with etomidate and fentanyl (17). Other commonly used inhalation agents include desflurane, enflurane, and sevoflurane. Overall different anesthetic agents show a similar EEG pattern when used at a concentration below their minimal alveolar concentrations (MAC) level (the level necessary to prevent movement to painful stimuli in about 50% of subjects).
EEG Change during Induction
Most anesthesia inductions are carried out by etomidate, propofol, or thiopental via intravenous administration. This initially produces an EEG showing beta activity with a decrease in the alpha rhythm. The beta activity rapidly becomes more widespread, with increases in amplitude. This is followed by a slowing in the beta frequency that approaches the alpha frequency activity. During this phase, the beta or alpha activity may be intermixed with a burst of high amplitude, intermittent rhythmic delta activity, which is often of frontal dominance, that is, FIRDA (frontal intermittent delta activity) before a steady-state anesthetic pattern is established (Figs. 39.1A, B and 39.2A,B) (18).
EEG Patterns at Sub-MAC Concentration
At this light level of steady-state anesthesia, a widespread anteriorly dominant rhythm (WAR) pattern, usually in lower beta or alpha frequency activity, is seen with all of the above described agents (18). The frequency of this pattern tends to slow with increasing concentration of these agents. This anesthetic WAR pattern is strikingly similar to the alpha-coma pattern often seen in a postanoxic cerebral insult (Figs. 39.1D, 39.2D, 39.3A, and 39.4A). However, the alpha frequency WAR pattern may be simply due to slowing of the beta frequency activity induced by anesthesia and may approach alpha or even theta frequency activity.
In addition to the WAR pattern, anterior maximum intermittent slow (AIS) waves may appear (Figs. 39.1C and 39.2C) (18). AIS waves are commonly diphasic having a sharply contoured negative-positive configuration with a duration of less than a second occurring singly or in brief trains.
Another pattern during this level of anesthesia is widespread persistent slow (WPS) waves (Figs. 39.1C and 39.2C) (18). The WPS waves consist of polymorphic slow waves with a duration usually exceeding 1 second. This pattern is least obvious with halothane and enflurane and is most prominent with isoflurane (18). With deepening of the anesthetic level, there is an increase in the amplitude and wave length of the WPS pattern and a decrease in WAR pattern.
EEG Pattern with Supra-MAC Concentration
The EEG patterns of different anesthetic agents are similar when the concentrations are at sub-MAC level. But the EEG patterns are different when the concentration levels are above MAC. For instance, enflurane elicits spike and wave at 1.5 MAC and a burst suppression pattern that may include a spike component at MAC levels greater than 2. At these levels even seizure activity may be elicited when activated by hyperventilation (19).
In contrast, isoflurane tends to exert an antiepileptic effect producing an intermittent suppression pattern at 1.5 MAC and continuous suppression at MAC above 2. Halothane also has an antiepileptic property but may not produce EEG inactivity even with concentrations as high as 4 MAC (18). Desflurane and isoflurane tend to rapidly produce EEG patterns of suppression or burst suppression at supra-MAC levels. Adding nitrous oxide to isoflurane or desflurane may potentiate the EEG suppression at a lower concentration of the primary anesthetic agent (18).
EEG Pattern During Recovery from Anesthesia
When discontinuing anesthetics at the sub-MAC level, the WAR pattern decreases in its amplitude and increases in frequency, which subsequently changes to the beta range activity. There is also a decrease of the WPS pattern before the patient regains consciousness (18). During the transition from the anesthetic state to the arousal state, bursts of intermittent delta activity, resembling a FIRDA pattern, may appear which are similar to but less prominent than those seen during induction.
Other Factors That Affect the EEG
The decreasing PaCO2 level below 40 mm Hg or decreasing the amount of stimulation that would be painful if given during awake state would produce a pattern suggestive of a deeper level of anesthesia, that is, increase of slow waves, especially during light sub-MAC level of anesthesia. Severe blood pressure drops are associated with increases of slow waves and eventual flattening of the EEG activity. Hypothermia slows the EEG frequency spectrum with gradual reduction of amplitude and eventually leads to EEG “flattening” at about 20°C to 25°C. However, electrocerebral inactivity created by severe hypothermia could act as cerebral protective mechanism. Hypothermia also accentuates the EEG effect of anesthetic agents (18).
EEG MONITORING
EEG has been used for monitoring the brain function during CEA for many years and probably has been the most commonly used method for the detection of cerebral ischemia that would determine the need for selective shunting. EEG can be recorded easily in the operating room despite an electrically hostile environment where multiple electrical instruments are connected to or working nearby the patients and surgical personnel. Most EEG monitoring are performed under general anesthesia and its activity may vary depending on the type and depth of anesthesia, blood pressure, body temperature, or other physiological factors such as PaCO2 and PaO2 level. These variables should be thoroughly understood by the monitoring team.
Recording Technique
Because EEG changes during the CEA can be diffuse or focal, the electrodes across the entire scalp, using 16 EEG data channels, are commonly used. It is preferable to use collodion, which ensures the secure attachment of electrodes to the scalp during the surgery.
The anterior-posterior longitudinal bipolar (so-called banana) montage is commonly used because this montage allows easy detection of hemispheric asymmetry.
The filter setting is usually the same as of routine EEG with the high (low pass) filter of 70 Hz. Some laboratories may choose the low (high pass) filter setting of 0.3 Hz and high (low pass) filter setting of 35 Hz. Because of the electrically “noisy” operating room environment, 60 Hz contamination onto the EEG recording may be inevitable. The monitoring team should try to find the source of 60-Hz artifacts and to eliminate the artifacts by technical modifications if possible. If 60-Hz artifacts cannot be eliminated, the use of 60-Hz notch filter is allowed.
![]() Figure 39.3 (continued) Within a few seconds after the shunt was placed, the EEG started to show recovery (C,D). It took more than 3 minutes to recover fully to the preclamp EEG (E). |
The sensitivity setting is usually 7 or 5 µV/mm. It is not uncommon for the EEG amplitude to be exceedingly low under anesthesia, requiring a sensitivity of 3 µV/mm.
The sweep speed is usually a 10-second display on a full video screen but may be adjusted to 5 seconds or 20 to 30 seconds screen depending on the interpretation needs and preference of electroencephalographer.
A preoperative, awake resting EEG can be recorded in the operating room before anesthesia induction. This allows inspection of any preexisting EEG abnormalities before anesthesia. At least 5 to 10 minutes of baseline preclamp EEG is essential while the patient is under stable anesthesia to appreciate any clamp-associated EEG changes. After the clamp is applied, the EEG of the preclamp state may be displayed on half of the video screen, so that the postclamp on-going EEG can be directly compared with preclamp EEG. Although most clam-prelated EEG changes occur within 20 to 30 seconds after the clamp, the EEG should be monitored throughout the procedure because EEG changes may occur in the midst of procedure, long after the clamp has been applied. Possible causes for the late EEG changes include hypoperfusion secondary to a lowered blood pressure or embolism, or shunt malformation if a shunt is used.
Preclamp Focal EEG Abnormalities in Relationship to the Anesthesia
The majority of EEG patterns during CEA show a symmetric baseline pattern before the clamping of the carotid artery. However, depending on case selection, 30% to 40% of preclamp EEG may show focal abnormality of varying degree (20). The focal abnormality may consist of unilateral reduction of the WAR amplitude. This may be accompanied by polymorphic delta activity on the side of the reduced amplitude. Most focal EEG abnormalities under anesthetic usually correlate with a preoperative waking EEG abnormalities. However, anesthesia may activate an abnormality that was either inapparent or less apparent during the waking state (18). Conversely, the anesthesia may obscure a focal abnormality in some cases that was apparent in EEG recordings during the awake state.
In patients with a lesion in the anterior head region where the alpha rhythm tends to be unaffected in the awake state, the anesthesia may bring out a reduction of the WAR pattern along with decreased beta and increased polymorphic slowing in the anterior head region. Preexisting intermittent rhythmic delta in the temporal region in the awake state may become more prominent and persistent, along with a reduction of the WAR pattern (18). A more posterior lesion having decreased alpha activity on the side of lesion may leave the anesthetic WAR pattern symmetrical without focal slowing (18). Preexisting nonlateralizing FIRDA or persistent generalized slowing during awake state may be observed and cannot be distinguished from the anesthesia-induced slowing that commonly occurs during induction or during the recovery phase of anesthesia (18).
EEG Changes after Cross-Clamping the Carotid Artery
Most preclamp EEG patterns have a symmetrical pattern without focal features and the development of an asymmetric pattern after the clamp can be easily recognized. The clamp-associated EEG change occurs within the first minute in the majority of patients (>80%) with most (69%) appearing within 20 seconds after the clamp (21). The most common and sensitive EEG change indicating cerebral ischemia is the decrease of alpha and beta frequency activity (WAR pattern) on the clamped side (Figs. 39.3A,B and 39.4A,B). Fortunately the most common EEG pattern under stable sub-MAC level of anesthesia consists of abundant alpha and beta activity (WAR pattern).
Further progress of ischemia is associated with an increase of delta amplitude or slower frequencies. The most severe degree of EEG change is “flattening” of the EEG pattern with depression of all activity including delta and faster frequency components (Figs. 39.3C and 39.4B). These changes occur primarily on the hemisphere ipsilateral to the side of clamp but a bilateral change could occur if the blood flow of contralateral hemisphere depends on the collateral circulation from the ipsilateral hemisphere. This may occur when occlusion or severe stenosis of the contralateral carotid artery exists. When bilateral changes occur, it may be difficult to differentiate if changes are due to global ischemia or systemic factors such as a change in anesthetic level, blood pressure, temperature, or PaCO2/O2 level. However, the bilateral change secondary to hypoperfusion usually shows a greater EEG change where more “flattening” of EEG activity occurs in the presence of slower delta and/or greater depression of fast activity over the ipsilateral hemisphere (Fig. 39.4B,C).
After the shunt is placed, the EEG recovers gradually to the baseline state (preclamp EEG) and may take several minutes to full recovery. It appears that the longer the clamp time before shunt placement occurs, the slower the recovery time. In a small number of patients, the EEG improves but never recovers to the preclamp state. Most of these patients wake up with no neurologic deficit. If any, the deficit is minor or transient. In the majority of shunted or nonshunted cases, the EEG remains symmetric throughout the procedure. In a small percentage of patients, transient and focal EEG changes may appear during the middle of the procedure, long after the cross-clamping has occurred. These transient and focal EEG changes have been thought due to the asymmetrical effects of changing level of anesthesia or resurgence of preexisting focal abnormality. These transient changes have no neurologic consequence (20). This finding may be due to decreased blood pressure. Because the threshold of blood pressure to maintain the appropriate cerebral perfusion is not known and varies individually, slight reductions of systemic blood pressure may result in focal areas of ischemia bringing out EEG changes especially when the blood pressure at the time of clamp is close to the threshold level. Raising the blood pressure usually corrects the adverse EEG change. Another possibility of late and persistent EEG change may be due to embolization especially during dissection or at the time of clamp release which will likely result in neurologic deficit.
A late EEG change may also occur in shunted patient. If this is not due to blood pressure, anesthesia, or embolization, the shunt malfunction should be considered
Predictability of EEG Change
The overall incidence of clamp-related EEG changes has been estimated to be about 10% to 30% (20, 21, 22 and 23). Most studies have agreed that the incidence of clamp-related EEG changes was higher in patients when contralateral carotid artery was occluded. When comparing patients with a patent and occluded contralateral carotid artery in a total of 564 patients from a single institution, a significantly greater incidence of ipsilateral EEG change was found in patients with an occluded artery (39%, 22 patients out of 57 patients) than in patients with patent artery (16%, 80 patients out of 479 patients) showing statistically significant difference (P < 0.001) (24). The greater incidence of clamp-associated EEG changes was found with >90% than <50% stenosis of contralateral carotid artery (25). Other studies also agreed that 30% to 40% of the patients with contralateral occlusion required shunt based on EEG findings (26,27).
Another study also reported a 48% incidence of clam-prelated EEG change with contralateral carotid occlusion compared to 18% for stenosis and 21% for normal carotid artery (28). Clamp-related EEG changes are also more common in patients when contralateral abnormalities on the preoperative awake EEG exist than in patients with only ipsilateral or diffuse abnormalities (21) or patients with previous history of stroke (29). It should be noted that there are still substantial numbers of patients who have a complete occlusion of the contralateral carotid artery that do not show EEG changes when clamping of the ipsilateral carotid artery occurs. This is likely due to adequate collateral circulation. In such cases, the surgeon may prefer to shunt even if there is no EEG change.
It is intuitive to expect that the greater degree of ipsilateral stenosis is associated with a lower chance of EEG change, because the collateral flow from the contralateral hemisphere would be well established in patients with severe stenosis than in patients with a patent ipsilateral carotid artery. However, one study did not support this expectation (15).
CEA RESULTS WITH AN EEG MONITORING FOR THE SHUNT NEED
There are three types of surgical preferences for CEA: one is a surgical protocol using shunts routinely (9,30), another protocol will not use a shunt at all (21,31), still another protocol places a shunt selectively by one or more monitoring techniques (20,32). The reported incidences of perioperative stroke were not much different among these three protocols but the overall incidence tends to be highest among those using a shunt routinely and the lowest among those who do not use a shunt at all.
This suggests that perioperative stroke are more likely due to embolism rather than cerebral ischemia, and thus the necessity of any type of monitoring can be questioned. Only a large-scale, prospective study in a single institution can make accurate comparisons of these three methods of surgery. Nonetheless, many studies agree that CEAs without shunt appear to be associated with a substantially higher risk of stroke than CEAs without selective shunting (15,23,33). In a retrospective review of 902 consecutive CEAs from a single institute, 591 CEAs were performed without shunting and also without EEG monitoring. This was compared to 311 CEAs that were done with selective shunting based on the EEG findings (15). This study reported that clamp-related EEG changes occurred in 40 patients (12.8%) and a postoperative stroke occurring in only one patient (0.32%) in the selective shunted group, whereas in the nonshunted group (no EEG monitoring), 13 patients (2.19%) had postoperative stroke (P < 0.05).
This suggests that perioperative stroke are more likely due to embolism rather than cerebral ischemia, and thus the necessity of any type of monitoring can be questioned. Only a large-scale, prospective study in a single institution can make accurate comparisons of these three methods of surgery. Nonetheless, many studies agree that CEAs without shunt appear to be associated with a substantially higher risk of stroke than CEAs without selective shunting (15,23,33). In a retrospective review of 902 consecutive CEAs from a single institute, 591 CEAs were performed without shunting and also without EEG monitoring. This was compared to 311 CEAs that were done with selective shunting based on the EEG findings (15). This study reported that clamp-related EEG changes occurred in 40 patients (12.8%) and a postoperative stroke occurring in only one patient (0.32%) in the selective shunted group, whereas in the nonshunted group (no EEG monitoring), 13 patients (2.19%) had postoperative stroke (P < 0.05).
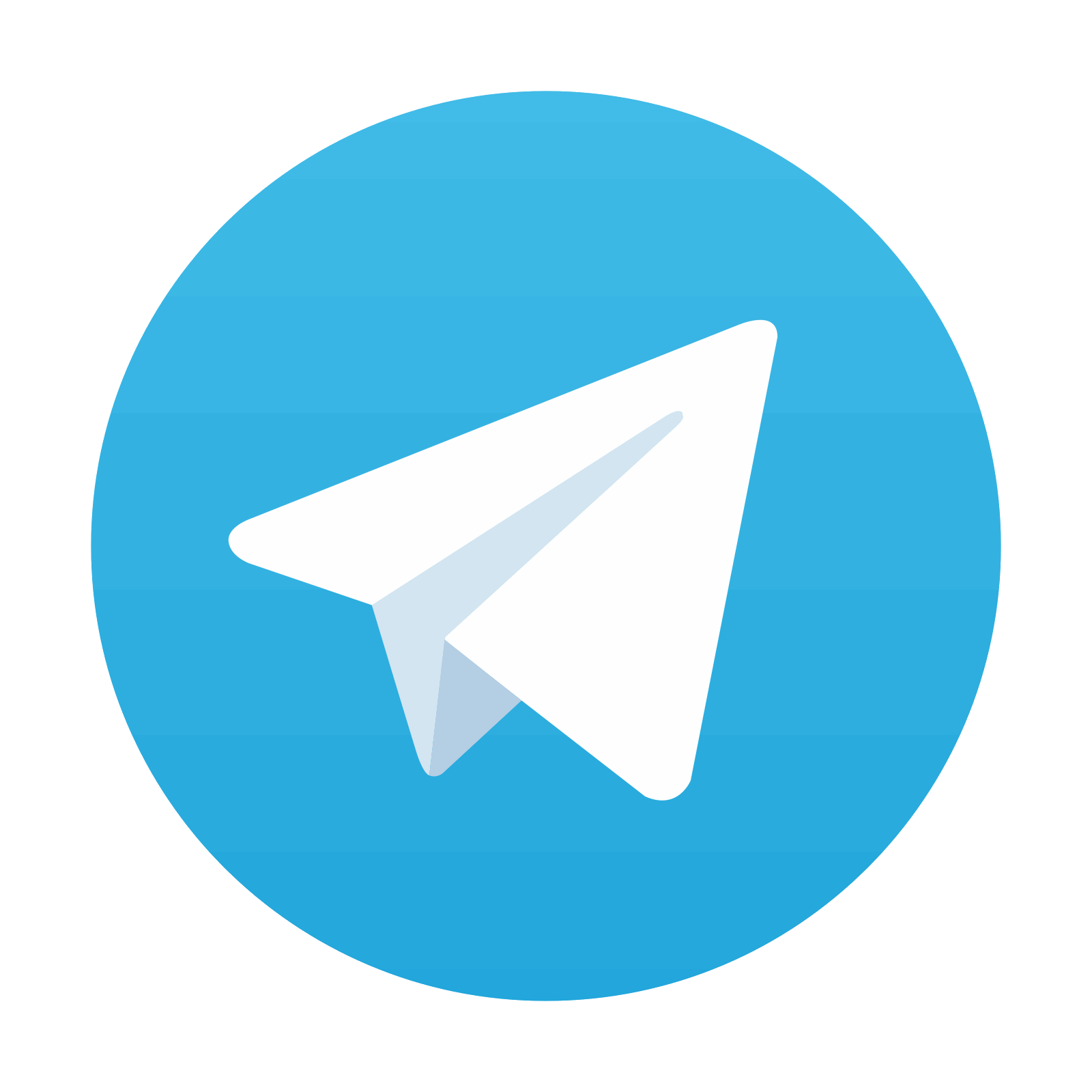
Stay updated, free articles. Join our Telegram channel

Full access? Get Clinical Tree
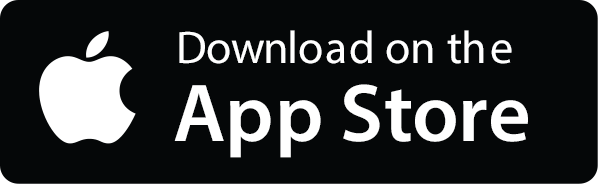
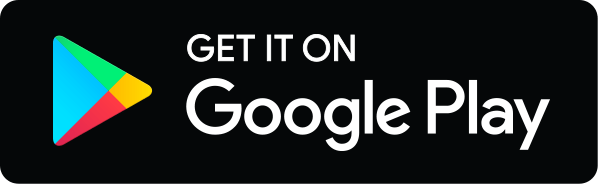