Figure 1.1. Acute interdisciplinary ICU program.
As the neuroscience ICU becomes established and clinical activity increases, the staffing model becomes key to continued growth and success. Comprehensive care, the goal of any ICU team, is possible only with the provision of ample physician level coverage – attendings, nurse practitioners, fellows and residents – for 24/7 patient oversight. A variety of staffing models exists in U.S. neuroscience ICUs and is an important element in the organizational perspective and intent of the ICU (Table 1.1).
|
Table 1.1. Neuroscience Intensive Care Unit: organizational perspective.
1.1.4 The Standalone Neuroscience ICU: The Hospital Versus the Physician Argument
As medical centres strive to grow their acute neuroscience programs, the debate of creating a subspecialty neuroscience ICU versus managing patients in a more generic model of critical care (general medical or surgical ICU) is often entertained. Arguments for both clinical domains can be made. The hospital argument against a subspecialty ICU is mainly justified by the increased inefficiency of the ICU system. Limited bed flexibility, staffing with ICU specific nurses, and increased physician coverage all increase the overall cost of ICU management (Table 1.2).
|
Table 1.2. Impediments towards a subspecialty neuroscience ICU.
On the physician side, there are numerous overt and potential patient outcome benefits and financial remuneration to the medical centre that should offset the inefficiencies inherent to a subspecialty ICU system. These advantages can be summarized as 1) direct patient benefit in outcome measures as supported by published data; and 2) strengthening hospital neuroscience initiatives and efficiencies of care (Table 1.3). With regard to improved outcomes, there are several published comparative series that review patient care in both neurological and neurosurgical disease. In neurology, intracranial hemorrhage is the most common diagnosis as it accounts for the highest incidence of a homogeneous group of neurological admissions to a neuroscience ICU. Reports by Diringer et al. and Mirski et al. both support the benefit of a neurological subspecialty ICU in terms of decreased mortality, from an estimated 40-50% in a general ICU to a mean of about 20-25% [9,10]. Others groups have also reported the benefits of a subspecialty neuroscience ICU, with lower mortality and improve ICU management [11-13].
|
Table 1.3. Standalone Neuroscience ICU: the physician argument.
Furthering the argument, the U.S. business community led an initiative to provide improved ICU care to their insured employees as a means to enhance quality of care and reduce spending. The Leapfrog Initiative http://www.leapfroggroup.org) lists ICU care metrics presumed to achieve these goals. Among them are several pertinent to the presence of an ICU intensivist: 1) intensivist(s) present in the ICU during daytime hours, days per week, with no other clinical duties during this time; 2) return rates of over 95% of pages within 5 minutes; and 3) reliance on a physician (e.g., fellow or resident) or a non-physician extender in the hospital and able to reach ICU patients in less than 5 minutes during non-daytime hours. In an environment clamouring for more physicians to specialize in critical care, the arrival of neurointensivists to direct and manage existing neuroscience ICUs (or neurosurgical units which are commonplace) makes good business sense. Modelling out such intensivist-driven care, Pronovost and colleagues at Johns Hopkins estimated a cost savings to a medical centre, beyond the additional expense of financing a subspecialty intensivist-driven ICU, of between $ 800,000 and $ 3,000,000. Even in a worst case scenario, the savings would exceed $ 1 million on average [14] (Table 1.4).
Revenues |
|
Expenses |
|
Table 1.4. Potential areas of revenue and expense for a neuroscience ICU. Specific to this issue, comparison of the function and cost of care of a newly formed neuroscience ICU as compared with the same patient population cared for in a mixed medical-surgical ICU set up just 2 years earlier yielded substantial cost savings in length of stay and cost reduction in expenditures for radiology, pharmacy and laboratory testing services [10].
1.1.5 Conclusions
Looking toward the future, the reality is that even if all the current academic fellowship training sites for neurocritical care continue to graduate several dozen subspecialty intensivists, their number will be too few to meet the demand within the acute neurosciences. In large, medium, and even smaller community hospitals, there will be a greater need for neurological expertise in the critical care environment as we promote newer therapies for acute stroke, head injury, and perioperative care. In general, much of this domain is beyond the usual training of a medical or surgical intensivist. That may indeed have to change. But currently neurological disease is not well addressed in ICU programs in the U.S., and too few neurologists seek engagement in a hospital critical care setting. The likely answer lies in the realm of the expanding demand and supply of telehealth solutions that can provide virtual bedside consultation directly between an intensivist or a surgeon and a neurointensivist. In the U.S. alone there is a disproportionate situation of a mere 50 well-staffed neuroscience ICUs amongst 6000 hospitals and their corresponding medical and surgical critical care areas. As a sudden genesis of first tier comprehensive neurological centres is unlikely, it appears reasonable to expect that the care provided in such domains must be directed outwards. That will very likely be the second evolution of neurological critical care.
1.2 NICU Organization: Past, Present and Future: Prof. Robertson’s Point of View
I would like to see the day when somebody would be appointed surgeon somewhere who had no hands, for the operative part is the least part of the work
Harvey Cushing
Neurocritical patient care draws a parallel line with the principles of general intensive care which requires a thorough understanding of the pathophysiology and focuses initially on the resuscitation of patients in extreme physiological deterioration. The focus of the initial approach is usually on the acute condition without considering in detail the patient’s medical chronic condition. Then, the intensivist should gather the patient’s medical history, essential to complement the assessment of his/her current physiological condition. General intensive medical care has been predicated on the ability to give time for a specific therapy to work or for restoring tissue to promote the recovery of organ function. However, there are few available effective therapies for brain injury (trauma, vascular, etc.): the limited plasticity of the fully mature brain should also be considered.
1.2.1 Past
The history of neurosurgery and the management of traumatic brain injury (TBI) are inextricably linked. Trepanation was sometimes named the “first surgical procedure”, with the first reported trepanations found in Northern Africa and dating back to around 10000 BC [1]. There is abundant archeological evidence for the success of trepanation worldwide, with the Peruvian Pre-Columbian collection being perhaps the most noted [2]. Identifying the purpose of this practice isn’t easy, but some answers include both medical reasons and mystical practices: treatment for headache after TBI [3], treatment for epilepsy, migraine or psychiatric disorders, and even “letting out the evil spirit.”
It is prudent to mention that there is ample room for speculation in this area, and that the “after surgery” care provided by the “first neurosurgeons” also gives rise to controversy. Very likely, trepanation developed in different regions of the world independently. A precise starting point of the history of neurosurgery and neurocritical care surgery is a topic of discussion, and some medical historians have preferred to establish arbitrary phases in the history of neurosurgery [4] or characters or regions, without any current convention universally accepted.
To mention some from the ancient world: the Edwin Smith Surgical Papyrus is about 3700 years old and an alleged copy of a 5000-year-old manuscript (during Egypt’s Old Kingdom [5]) describes injuries in the head, spine and/or spinal cord, as well as other neurological conditions. Of course, the father of modern medicine is the Greek Hippocrates of Cos who, in his treatise “On Injuries of the Head” written around 400 BC, described six specific types of TBI, treatments and even prognosis [6]. Six centuries later, Galen (129-200 AD), a Greek physician working in Rome, improved the trepanation technique based on the foundations of the Hippocratic body of works and collected, developed, and expanded all previous medical knowledge. His works became the basis of knowledge of the nervous system taught up until the Renaissance and into the late sixteenth century [7]. In the Middle Ages, neurosurgical developments, like all other scientific knowledge, were obscured by medical scholasticism, where metaphysical explanations became prominent in medical schools and “Hippocratic/Galenic orthodoxy” prevailed. The subsequent translation of Greek and Latin writings into Arabic and back into Latin led to many misunderstandings, particularly in the anatomical works, and those new findings that differed from the ancient texts were unfortunately ignored. The lack of anatomical knowledge and poor surgical outcomes led physicians to recommend against brain surgery.
With the Scientific Revolution of the sixteenth to the nineteenth century, neuroanatomy based on direct observation returned, allowing new detailed descriptions of anatomy and physiology, including other vital organs such as the heart and vessels, as well as innovative approaches. The first published attempt (failed) to resurrect a dead patient, with the implicit hope that the brain would recover, can be attributed to Paracelsus in 1530. Jackson, in 1746, described the insertion of a tube into the airway to provide artificial respiration. He proposed the possibility that “it may be necessary to make an opening in the windpipe for this purpose.”
Joseph Black discovered carbon dioxide in 1754, and Joseph Priestley discovered oxygen in 1774. Chaussier developed a device that administered oxygen to humans in 1780, which closely resembled the mask with reservoir bag in use today (but without a valve). Concern about possible adverse consequences of positive pressure ventilation and excessive oxygen administration began soon after its appearance, and still continues today. Negative pressure ventilation started with the first tank respirator invented in 1832 by Dalziel, and pursued by von Hauke 40 years later.
In 1880, Waldenburg invented the cuirass respirator which covers only the chest instead of the entire body below the neck. Bowditch invented the first mechanical ventilator in 1879, with several of his contemporaries making significant contributions [8].
Little progress was made during this period of almost 400 years owing to the lack of the concept of brain mapping, availability of anesthesia, and understanding of infections. The immediate need for trepanising after TBI was not appreciated and less importance was given to the neurological damage until the last decades of the nineteenth century. Hutchinson in 1867 published a series of works that introduced a new diagnostic sign for brain injury: third cranial nerve palsy. In that year, Lister published his first works on antisepsis.
1.2.2 Present
Modern neurosurgery can trace its origins back to the late nineteenth century. By 1880 a surgical environment free of infection was established, laying the scientific basis for deliberate surgical invasion of the central nervous system as we know it today. Results of surgical interventions, though imperfect, were significantly improved over previous useless treatments. The most important contributions to our understanding of the microscopic anatomy of the nervous system were made by Santiago Ramón y Cajal in a series of works that began in 1892. Neurologists fostered neurosurgical interventions by diagnosis and locating lesions. Since most of the surgeons lacked any neurological skill, they also helped the neurosurgeons in the operating room and postoperative care.
In 1920, Cushing talked about neurosurgery as a “new specialty”, creating a separate entity, the American Association of Neurological Surgeons. He reported a 7.4% operative mortality in his series in Boston. At that time, European centres reported mortality rates between 40% and 50% [9], emphasizing the need for special training beyond general surgery, turning it into a national American model, with a subsequent demonstration of its success in training, treatment outcomes, and research productivity: Cushing’s model became the global model.
The poliomyelitis epidemic with subsequent respiratory failure revived the development of negative pressure ventilators. Stewart and Rogoff improved the original concept of the tank in 1918. In 1929, Drinker and McKhann described further refinements, and Drinker’s “iron lung” saved countless lives. The subsequent use of endotracheal tubes allowed wider use mechanical ventilators. In the polio epidemic of the 1950s, medical students frequently used the reservoir bag system to ventilate patients for weeks. The mechanical ventilator was first introduced by the Bennett Corporation in 1948. The ability to maintain the breathing of polio patients with any of these mechanical ventilation systems defined the start of modern neurocritical care.
Many neurologists became the family doctors for these patients. A battle began between neurologists and infectivologists over who should take care of patients with other forms of neurogenic respiratory failure, such as tetanus, botulism, Guillain-Barré syndrome, and myasthenia gravis. Fortunately, in the early 1950s, Salk developed the polio vaccine which, with the Sabin vaccine, led to the near eradication of this scourge from the face of the earth.
Again, medical historians face the question of the first general intensive care unit with three reasonable candidates: Johns Hopkins Hospital in Baltimore, Maryland, in 1928 [10], with a three-bed unit specialized in post-surgical care; Bjørn Ibsen, in Copenhagen, Denmark, in 1952 [11]; and Peter Safar in 1961 in Baltimore City Hospital, whose interest focused on cerebral protection and resuscitation. Therefore, the early generation of intensivists viewed neurological function as a major part of their responsibility [8]. At the University of Colorado, Earnest established a neurological ICU in 1969. Jackson led a course in intensive care for neurologists in Cleveland in the 1970s.
The Baylor College of Medicine opened its neurosurgical ICU in 1982 at Ben Taub General Hospital in the Texas Medical Center in Houston, and it is still one of the largest units specialized in neurotrauma critical care and research. Ropper, Kennedy, and Zervas opened a neurological/neurosurgical ICU at Massachusetts General Hospital and wrote the first textbook on neurocritical care in 1983 [12]. Hanley and Borel opened a neurocritical care unit with a training program at Johns Hopkins Hospital, Fink at Columbia, and Haley and Bleck at the University of Virginia in 1989. Although the first contemporary neurosurgical ICUs date back about 40 years, neurocritical care is a recently recognized specialty: in 2007, the United Council for Neurological Subspecialties approved neurocritical care as a subspecialty and the first certification exam was also featured that year.
Evolution of Technology in Neurocritical Care
Neurocritical care is an important and ongoing topic in research, education and medical practice. After brain injury, a key principle is that the primary lesion helps to determine the prognosis, but secondary injury (inflammation, edema, free radicals, etc.) also plays an important role in the outcome. Of paramount importance in neurointensive care is the close monitoring of the factors that usually ensue and contribute to the worsening of secondary injury, i.e., hypoxemia, hypotension, seizures, fever, and intracranial hypertension.
Initially, neurological ICUs (NICUs) between the 1960s and 1980s focused almost exclusively on the postoperative care of neurosurgical patients, and neuromonitoring was restricted mainly to serial neurologic examination and, in some units, monitoring of intracranial pressure (ICP). Often, these neurological examinations did not reveal changes in brain function until they were irreversible. The idea was to detect clinical deterioration as soon as possible, allowing early efforts to reverse or correct any damaging process. This era can be considered as the age of “clinical neuromonitoring” [13]. A brief description of the evolution of the technology typically used in modern neuromonitoring and management is provided here.
Intracranial Pressure Monitoring
The first published reports of left ventricular puncture date back to 1850. Over the next 100 years, the procedure was refined; with the addition of a manometer in 1948, the modern system of external ventricular drainage (ventriculostomy) was introduced, but its routine use began only in the early 1970s for treating Reye’s syndrome and subarachnoid hemorrhage. In the early 1980s, several reports appeared showing better results in severe TBI with the use of ICP monitoring. In 1995, ICP monitoring was officially recommended for trauma victims with a Glasgow Coma Scale (GCS) score of 3-8 [14]. Typically, ventriculostomy is one of the first procedures taught to neurosurgery residents and it is still the most complex procedure carried out without supervision. Technology has now provided several new strategies to measure ICP non-invasively, but the need to place a ventriculostomy will continue.
Temperature and Brain Tissue Oxygen Monitoring
Clark introduced his semipermeable probe of polarographic cells in the 1960s. In the 1980s, Fleckenstein developed the PbtO2 system used in LICOX systems. Since the temperature coefficient is required to calculate the value of oxygen, researchers assumed that rectal temperature reflected brain temperature. Studies carried out by the Baylor College of Medicine at Ben Taub General Hospital later found that brain temperature was consistently and significantly higher than core temperature after brain trauma [15]. The advent of new combined monitors has helped to underscore the importance of controlling fever in patients with severe TBI and to monitor the effectiveness of therapeutic hypothermia. Another new method uses fibre-optic technology and measures PbtO2, PbtCO2 and pH.
Monitoring of Jugular Venous Oxygen Saturation (SjvO2)
Cerebral venous oxygenation was investigated as part of neuromonitoring for 70 years by Gibbs who started in 1935. Use of the fibre-optic catheter in TBI introduced in 1983 by Cruz in Brazil and Philadelphia led to the wider acceptance of SvjO2 monitoring supported by validation studies which showed its usefulness in neurotrauma at the Baylor College of Medicine in Houston, Texas, in the 1990s.
Microdialysis
Devised in 1974 by Ungerstedt in Stockholm, Sweden, human brain microdialysis in neurointensive care has become by far the most widely used application of this minimally invasive technique. Since 1984, bedside analyzers have been used more in Europe than in the United States in patients with severe TBI and subarachnoid hemorrhage. The substances that can be potentially measured are innumerable: neurotransmitters/metabolites related to energy (the most common current application), markers of tissue damage and inflammation, drugs administered, etc. The first effort to reach consensus on the indications for microdialysis in neurointensive care was undertaken in Cambridge in 2000, with a follow-up meeting held at the Karolinska Institute in Stockholm in 2002.
Therapeutic Hypothermia
The first modern scientific report describing the clinical application of therapeutic hypothermia in a case series of patients with severe TBI was published by Fay in 1945. In the 1960s, Rosomoff and Safar carried out studies that discouraged its use because of the adverse effects with profound hypothermia (<30° C). But in the 1980s interest was revived using mild hypothermia with few and minor severe adverse effects thanks to improvements in the capabilities of ICUs to control and prevent side effects. Therapeutic hypothermia is now used for neuroprotection and management of ICP, one of its most studied uses, and always deserves close neurointensive care. Theoretically, local hypothermia may have certain advantages over the adverse effects of systemic hypothermia, and this matter is still under development.
Electroencephalographic Monitoring (EEG)
Far from being unique to neurocritical care, EEG monitoring has become a common tool in NICUs. The first physiological studies were presented by Berger in 1920 in Germany. Gibbs published his first works in clinical EEG in 1935 in Boston at Massachusetts General Hospital, and Jennett in 1974 wrote his work on epilepsy after TBI. In the neurointensive care setting, continuous EEG (cEEG), short-term EEG, and new variants such as the compressed spectral array (CSA) and bispectral index (BIS) help in the diagnosis, prognosis and assessment of targeted therapy.
Neuroimaging
It would be hard to imagine modern neuroscience without the currently available neuroimaging techniques. Since its emergence in 1972 and thanks to the availability of computers, computed axial tomography (CT) easily replaced the archaic use of the angiogram to diagnose epidural hematoma, improving mortality and disability rates [16], transforming all the neurosciences, initially with a special clinical impact on neurotrauma. Neuroimaging is constantly evolving: mastering all new techniques seems impossible, but their usefulness in research and clinical practice remains undeniable. Radiology is one of the best examples of the impact of technology in today’s medicine.
Neurocritical care shares with general critical care several important systemic monitoring tools, as well as therapies essential to closely monitor and improve the chances of survival and recovery during the acute phase of illness, and they have become the standard care recommended by management guidelines. Management includes invasive blood pressure (arterial line), CO2 at the end of expiration (ETCO2), pulse oximetry, mechanical ventilation, continuous electrocardiography, central venous catheter, vasoactive drugs, sedation, nutrition, prophylaxis for gastrointestinal hemorrhage, thrombosis, seizures, pressure ulcers, and infections among others, which are typically provided in conjunction with neuromonitoring, not to mention the irreplaceable role of the clinical laboratory.
Assessment Scales
Because critical care is focused on severely ill patients, where “severe” is not always easy to define, several sets of scales have been developed to help establish specifically how severe ill a patient is, thus facilitating the selection and evaluation of therapy, fulfilling criteria for entry into a research study, and to establish prognosis. Jennett and Teasdale published the Glasgow Coma Scale (GCS) in 1974, and Jennett the Glasgow Outcome Scale (GOS) in 1975 [17], both designed to clinically evaluate the central nervous system status in patients with neurotrauma. The APACHE scale (Acute Physiology and Chronic Health Evaluation) issued in 1981, replaced by APACHE II in 1985 by Knaus, was calculated from 12 routine physiological measurements (blood pressure, arterial pH, etc.) during the first 24 hours after admission. A refined scale known as APACHE III was published in 1992. SAPS II (Simplified Acute Physiology Score), devised by Le Gall in 1993, describes patient morbidity when comparing the results with other patients.
SAPS III is a system to predict a specific definition of mortality (e.g., 30-day mortality) when comparing groups of patients, but it requires computer calibration to achieve its functionality. The SOFA scale (Sequential Organ Failure Assessment Score) devised by Vincent in 1996 [18] is used to track patients’ status daily during their stay and to predict outcome and mortality; it evaluates the degree of failure of 6 organ systems (respiratory, cardiovascular, neurological, hepatic, renal and coagulation). The MPM scale (Mortality Probability Models), introduced by Lemeshow in 1988, predicts mortality at ICU admission, and an updated version (which requires no calibration) was introduced in 2005 [19]. The logistic model published by Narayan in 1988 uses multivariate analysis to predict the probability of positive or negative outcome in coma patients after severe TBI.
These are some of the most commonly used scales. There is still interest in developing new systems of scales or improving the existing ones in order to make them more accurate and specific. No scale is perfect or unlimited, and no scale is generally superior to good clinical judgment. Therefore, decisions in individual patients should never be taken based solely on a statistically derived scale of severity of injury. Where scale systems can be used is to estimate quantitatively the degree of acuteness in severe patients and to adjust the outcome assessments of hospitals [20].
The socioeconomic impact of general or specialized critical care undeniably derives from the fact that nearly 6 million adults are admitted to intensive care each year and that one out of 5 Americans dies in intensive care [21,22]. About 2% of the U.S. population are admitted to an intensive care unit each year, occupying 5-10% of all hospital beds but accounting for 20-35% of total hospital costs [23]. Despite its size, there is no formal standardization or federal monitoring of intensive care.
ICUs continue to have a significant local colour, with wide variation in staffing patterns. There is poor consensus on who should be admitted to an ICU, who should provide intensive care, and how much intensive the care should be delivered [24-28]. One third to one half of the U.S. population will enter an ICU during their last year of life [29,30], and a fifth will die there [31]. One aspect of the ICU on which there is little debate is that it is costly, absorbing about 1% of the U.S. gross domestic product [21,32]. There is also growing concern about the possible long-term sequelae of surviving critical illness [33,34].
Hence, it is essential to understand that intensive care is a significant part of a health care system, which is extremely large, extremely expensive, but not necessarily optimal. This is not always the case, as the U.S. health system is by far the most expensive in the world [35] but ranks 37th in overall performance and 72nd in population coverage [36]. ICUs are a smaller portion of health systems in other industrialized countries but still represent a significant and disproportionate segment of care and medical costs [37-39]. Even changes in how intensive care is proportionate may have public health ramifications that can affect an entire nation [40].
Together with the projection of the World Health Organization (WHO) that by 2020 road accidents, a major cause of TBI, will be the third leading cause of global burden of disease and injury, after ischemic heart disease and unipolar major depression [41], also the COMPACCS (Committee on Manpower for Pulmonary and Critical Care Societies) estimated that by 2020 the supply of pulmonologists will meet only 35% of the demand. By 2030, the gap will widen to 46% of demand. The shortage calculated for intensivists is 22% and 35% for 2020 and 2030, respectively [27].
This has placed the inevitable task on neurocritical care of proving its importance and, above all, its effectiveness. In 1989 and 1991, two studies conducted in England and the United States, respectively, documented considerable variability in the management of patients with severe TBI [42]: this led to the creation of TARN (Trauma Audit & Research Network) in Europe and the Guidelines for the Management of Severe Traumatic Brain Injury in the United States [14]. Subsequent publications comparing mortality before and after guidelines implementation showed a marked reduction in mortality [43-45], with subsequent evaluations in 2000 and 2006 showing marked adherence to guidelines since 1991 [46].
In 1997, members of the Task Force of the European Society of Intensive Care Medicine published recommendations for the minimum requirements for intensive care in ICUs [43], emphasizing the fact that intensive care medicine depends on the tight interaction between human, technological and space resources. Since the creation of NICUs, data on long-term outcome and quality of life of survivors of NICUs have been limited. A recent study in Austria found, however, that TISS-28 (Therapeutic Intervention Scoring System) scores on admission and at discharge and age were independent predictors of poor outcome in this unselected representative population of neurocritical care [47].
Outcome measurement is a suitable tool to reflect the daily work of intensivists and to legitimize its important implications for the allocation of hospital resources. Given the association between mortality and the economic impact of such patients, a more detailed understanding of this patient population may facilitate care and management decisions [48]. The purpose of any recommendation is to provide guidance for those planning a new department or adapting an existing one. However, it should be clarified that most recommendations are not always based on well-documented scientific evidence, but rather express the view of experts involved in intensive care medicine and may require adjustment to local situations. Many existing intensive care departments may be unable to comply with some recommendations due to human and financial resource issues.
As an emerging subspecialty, neurocritical care is a highly specialized field which demands specialized staff. There is growing concern about the need for trained personnel in neurocritical care, emphasizing the trend toward more efficient and accurate practice patterns designed for neurocritically ill patients. Modern NICUs are not only a place for neurocritical patients but also a place where advanced medical technology is coupled with the understanding of organ dysfunction resulting from nervous system failure. Several different types of institution-dependent practices of NICUs have emerged, in part because of competition between neurointensivists, neurosurgeons, neurologists, and other intensive care physicians, resulting in three different models of NICU organization: open, closed and closed-cooperative (hybrid). The selection of “best practice” is still under discussion and under scrutiny.
The open NICU is probably the most common model, especially in private practice. It involves a neurointensivist with privileges to admit patients to a NICU but not responsible for all patients of a NICU. The neurosurgeon can admit patients directly to a NICU and consult other intensivists to manage ventilated patients. The open model may decline in tertiary centres but it may persist in smaller NICUs where there are fewer resources for in situ 24-hour supervision of an intensivist [49].
In addition, other factors driving these changes include reductions in salary [50], loss of the physician’s individual autonomy, sharper distinction between hospital-based and consulting-based practice, and the increasing emphasis on patient outcomes. Some authors have demonstrated shorter duration of ICU stay, improved mortality rates and greater team satisfaction with the closed model.
The closed NICU is the model where all neurological ICU patients are managed primarily by the neurointensivist. After discharge from a NICU, patients are attended by the neurologist or the neurosurgeon team. Some units also take care of non-neurological patients. Most procedures are carried out by the NICU team.
The hybrid NICU is where neurology/neurosurgery continues to have an active role in managing NICU patients (mainly with regard to informing the family and taking long-term management decisions). The NICU team handles routine situations and is responsible for most procedures [51]. This model is generally adopted in teaching hospitals.
TBI cases are medically complex, involving the physical, cognitive, behavioural, social and emotional aspects of survivors. Often catastrophic, these cases require substantial financial resources not only for the patient’s survival but also to achieve optimum results in productive life, with a return to family responsibilities and work. TBI cases involve the injured person, his/her family, medical professionals including the attending physician and therapist, the attorney, the employer, the community resources, the source of resources, and usually the insurance company. The case management department facilitates the achievement of optimum results by engaging the collaboration of all parties involved, assessing priorities and options, coordinating services, and educating and communicating with all that concerns [52].
At the Ben Taub General Hospital (a Trauma Level 1 teaching hospital), our NICU has 16 beds managed by the Baylor College of Medicine and the staff of the Harris County Hospital District. It may be defined as a closed model, with a neurointensivist as medical director attending patients on the daily rounds, undertaken by the chief neurosurgeon of neurosurgery and neurosurgical residents (grades II, III, IV and chief resident), one or more temporary rotators (from general surgery, maxillofacial surgery, otolaryngology, plastic surgery, undergraduate medical students), a research team member, a physician assistant, the head nurse, the nursing administrator, and usually both a nurse from the previous shift and one from the following shift, joined once a week by the case manager, and the nutrition and rehabilitation staff.
The neurosurgery team performs the physical examination, writes progress notes and medical prescriptions, checks lab results and images, decides on admissions and discharges, performs most procedures and asks for consultations from other services when necessary. The medical director is available within the hospital 7 days a week during working hours and is reachable 24/7 by phone. The neurosurgery team is available within the hospital 24/7. The research team is available within the hospital during working hours and 24/7 by phone.
The nurse/patient ratio is 1:2, in a distribution of 13 contiguous beds arranged in a C shape, with a sink/bed ratio of 1:2, and a group of three additional isolation rooms that close the ring where the nurses’ station and administrative assistant occupy the centre, with an additional team for the telemetry monitoring system. Other routine staff that is usually not present during the daily rounds consists of inhalation therapy staff, radiology technicians who operate a portable CT scanner (exclusive of the NICU) for simple skull CT in addition to xenon-CT in coordination with the research team, the team of nuclear medicine for blood cerebral flow scanning with radionuclide, bioengineering technicians, social workers, pharmacy staff, and cleaning staff familiar with the NICU environment.
Medical Staff
The appointment of a neurointensivist as medical director and the availability 24/7 within the hospital of a neurosurgeon for trauma patients have been shown to improve survival, mortality and outcome, increase efficiency, reduce the length of stay, reduce the use of resources, facilitate the implementation of protocols (drugs, ventilation weaning, etc.) and daily formal visits to the patients, lower the level of frustration for nurses, improve the layout and clinical record documentation [45,47,53-56]. In contrast, there are also data demonstrating that academic institutions in the United States that offer 24/7 coverage by staff within the hospital and other medical extensions as critical care support, either by pager or cell phone, do not offer any temporary change in mortality, length of NICU or hospital stay [57].
Non-medical Staff
The collaboration between the NICU physician and the nurse has been promoted as a way to improve care. Theoretically, this allows the contribution of both professionals and should produce decisions that lead to better outcomes because the decisions are based on more complete information. Collaboration is defined as physicians and nurses working together, sharing responsibility for solving problems and making decisions to formulate and carry out plans for patient care [58].
This can be conceptualized and measured at either the individual patient or the organizational level of the NICU, and both types of collaboration have been positively associated with patient’s outcome in the neurocritical care setting [59]. The value of having nurses specialized in different ICUs has also been emphasized [58,60-62].
The importance of the nurse/patient ratio was highlighted in the European Prevalence of Infection in Intensive Care (EPIC) study conducted by the European Society of Critical Care Medicine, which suggested that minimizing the nurse/patient ratio reduced the spread of infections acquired in the ICU [63]. However, ICUs throughout the country are struggling to recruit and retain nurses. The projected growth rate of 6% in the supply of nurses will not match the 40% increase in demand by 2020 [64], and physician assistants (PAs) have been proposed as a partial solution to predicted shortages of doctors and nurses [65]. Although only about 6% of graduate PAs take advantage of advanced training programs [66], the example of effective functioning of PAs in surgical ICUs has been explored [67].
Research Team
Besides the undeniable importance of research to expand knowledge and enhance the practice of evidence-based medicine, placebo groups have demonstrated significantly improved outcomes when comparing data from the late 1980s: as we have seen, this course may be related to adherence to management guidelines, neuromonitoring and the presence of a specialized intensive care team; in theory, however, patients could benefit from having only closer monitoring as when they are enrolled in a research study.
In fact, it has become a common joke among investigators when asked about their availability to be study subjects, expressing their enthusiasm to participate and, if possible, to choose to belong to the placebo group. Awareness of the society about the importance of research lies primarily with the research teams: the errors committed by this particular workforce can negatively impact on the desire of society to participate in the future.
Rehabilitation and Physical Therapy
The early rehabilitation program in the acute phase has been associated with improved cognitive functioning levels, shorter duration of coma, and shorter hospital stay of patients with severe TBI [68]. However, some authors have found no benefit gained from early rehabilitation in elderly patients or patients with pre-existing morbidity after TBI [68]. Additional work is needed to determine how physical rehabilitation impacts on specific medical issues, long-term outcome and cost of health care.
Case Management
Severe TBI is often considered catastrophic in terms of cost, recovery time, consequences and burden on society. The case manager’s work in the long-term recovery after a disaster is to identify the needs of the survivor and connect him/her with resources, working with the committee for long-term recovery to do what is necessary to put patients back on their feet. The case manager works over the long term with the survivor to make a thorough assessment of his/her needs to determine which ones are related to the disaster and then help the survivor to develop a recovery plan.
The relationship between the manager and the survivor is to advocate; in doing so, the development of the relationship is as important as the patient’s needs. Because staff have experienced the largest growth compared with other hospital personnel, they also face the need to demonstrate their effectiveness. While this has also been explored [69], evidence for case management effectiveness is mixed. Some studies show a positive relationship [70,71], whereas there are conflicting results concerning the effects of case management on functional status, quality of life, family impact and other aspects of recovery. Further research is needed to resolve the doubts of the effectiveness of case management among TBI survivors and their families [69]. The value of the case management department at the patient’s level is irrefutable, even though the evidence for its effectiveness on a large scale remains unclear.
Transport of Critically Ill Patients
Despite the enormous sophistication of NICUs, sometimes not all patient care can be carried out there. Patients may need to be transferred to the radiology department, operating room, or more importantly, to other medical centres with ICU facilities for specialized care. These situations can jeopardize patient safety, as demonstrated in several studies [72-74], where increased adverse events during transport between facilities were rare, although analysis of the data in greater detail revealed a number of preventable events.
Patients transferred over greater distances or receiving vasopressor drugs may be at increased risk for minor adverse events during flight. Improvement could be achieved through better communication between the referring and the receiving hospital, and through strict adherence to and the use of a checklist of published protocols. Patients transported between ICUs are still critically ill and should be handled as such. A transport environment presents challenges not faced in an ICU, and care provider capabilities are limited once outside the unit. The transport of critically ill patients should be avoided at all cost, and it is advisable to plan to have portable diagnostic equipment for radiology procedures or make transports shorter (e.g., having the operating room nearby on the same floor).
Trauma Centres
NICUs must have well-defined communication channels in order to work closely with emergency rooms and rescue services, especially in the care of neurotrauma patients. This has led to the creation of regional trauma care services, with a positive impact on survival, mortality, length of stay, outcome, and resource utilization. A Level 1 trauma centre requires the immediate availability of trauma surgeons, anesthesiologists, specialized doctors, nurses, and a resuscitation team. Its goals include providing complete trauma care, serving as a regional resource, and providing education and research. The positive relationship between trauma systems and neurosurgeons has also been documented [75].
1.2.3 Future
The future of NICUs and other specialized ICUs seems to be both amazing and disturbing. Neurointensive care is expanding, with much work still to be done and countless questions to solve, while facing shortages in financial and human resources, along with the rest of the health system: physicians should participate as active players by exploring different solutions to the economical issues, while improving care in terms of outcome and cost-effectiveness. Having an ideal NICU is by definition impossible not only because of financial cuts but because patients, families, nurses and physicians have different perspectives on what an ideal ICU would be and what kind of culture would exist in an ideal ICU [76].
In the future, inputs from all these stakeholders will be required to help create an ideal ICU and the environment around the bed [77]. The NICU at Emory Hospital, Georgia, has explored a more patient/family-targeted approach, with rooms designed with enough space to perform complicated procedures at the bedside, eliminating the need to transport fragile patients elsewhere in the hospital. For the patient’s family there are rooms equipped with a folding sofa, wireless Internet access, and TV adjacent to the patient’s room. Separated by a glass wall, the rooms foster the family’s role in the healing process. Amenities within the unit, such as showers, kitchen and laundry facilities, alleviate many of the daily pressures on families.
The future often depends on technological advances and the NICU is no exception: cECG with improved diagnostic accuracy, as in arrhythmia, reduced autonomic activity, accurate non-invasive continuous blood pressure monitoring, systemic arterial blood gas monitoring, venous blood gas monitoring in the superior sagittal sinus (SSS), monitoring of cerebral tissue oxygenation, online indirect calorimetry. In 30 to 100 years, nanotechnology will provide us with new tools able to monitor cell and tissue function with unparalleled detail [78], perform quantitative pupillometry [79], diagnose diffuse axonal injury (DAI), and predict the outcome with magnetic resonance imaging-diffusion tensor imaging (MRI-DTI) [80].
Two recently published studies examining the feasibility of telemedicine in the ICU and the associated outcome of patients [81,82] proposed telemedicine as a way to relieve the predicted shortage of neurointensivists. New biomarkers of damage in brain injury have been documented, such as the breakdown products of αII-spectrin (spectrin breakdown products [SBDP]) after severe TBI [83] and subarachnoid hemorrhage [84], along with the neuron-specific enolase, S100B, and myelin basic protein in ischemic and traumatic injury [85]. Advances in technological and neuroprotective agents have attracted the attention of researchers, but much clinical work remains to be done in trying to answer physiological questions in TBI patients, such as individualization of treatment and prognosis based on the stratification of the pathophysiology of injury, early intubation at the emergency scene, and hyperoxia as neuroprotective treatment, adequate hemoglobin concentration, appropriate prophylaxis for venous thromboembolism, detection and treatment of adrenal insufficiency that probably contributes to the outcome [86]. Also, health care will continue to feel the pervasive influence of computers (electronic medical records, lab results and electronic images). Developing countries where resources are even more limited should recognize the fact that simple data collection can help to improve the outcome despite low budgets [87], for example, detailing the incidence, the causes, the approaches and the results, while keeping in mind the guidelines for improving data quality in a database of brain injury [88].
References
Part I
1. Evans RW (ed.). Neurology and Trauma. Oxford University Press USA, 2006, pp. 18-20
2. Breasted JH. The Edwin Smith Papyrus. New York Historical Society: New York, 1922
3. Rutkow IM. The History of Surgery in the United States, 1775-1900. Norman Publishing, 1988
4. Hippocrates. On Injuries Of The Head (translated by Francis Adams). The University of Adelaide Library: Adelaide (South Australia), 2005
5. Gross CG. Galen and the squealing pig. Neuroscientist 1998; 4: 216-21
6. Chamberlain D. Never quite there: A tale of resuscitation medicine. Clin Med 2003; 6: 573-7
7. Bleck T. Historical aspects of critical care and the nervous system. Crit Care Clin 2009; 25: 153-64
8. Chase CM, Ulatowski JA. Organization of a neuroscience critical care unit: historical perspectives and vision for the future. In: Suarez JI (ed.). Critical Care Neurology and Neurosurgery. New Jersey: Humana Press, 2004; pp. 9-23
9. Diringer MN, Edwards DF. Admission to a neurologic/neurosurgical intensive care unit is associated with reduced mortality rate after intracranial hemorrhage. Crit Care Med 2001; 29: 635-40
10. Mirski MA, Chang CWJ, Cowan R. Impact of a neuroscience intensive care unit on neurosurgical patient outcomes and cost of care. J Neurosurg Anesthesiol 2001; 13: 83-92
11. Suarez JI, Zaidat OO, Suri MF, et. al. Length of stay and mortality in neurocritically ill patients: impact of a specialized neurocritical care team. Crit Care Med 2004; 32: 2311-7
12. Varelas PN, Conti MM, Spanaki MV, et. al. The impact of a neurointensivist-led team on a semiclosed neurosciences intensive care unit. Crit Care Med 2004; 32: 2191-8
13. Varelas PN, Schultz L, Conti M, et. al. The impact of a neuro-intensivist on patients with stroke admitted to a neurosciences intensive care unit. Neurocrit Care 2008; 9: 293-9
14. Pronovost PJ, Needham DM, Waters H. Intensive care unit physician staffing: financial modeling of the Leapfrog standard. Crit Care Med 2006; 34: S18-S24
Part II
1. Ferembach D. La Nécropole Épipaléolithique de Taforalt (Maroc Oriental). Étude des Squelettes Humains. Rabat: CNRS, 1962
2. Rifkinson-Mann S. Cranial surgery in ancient Peru. Neurosurgery 1988; 23: 411-6
3. Weber J, Czarnetzki A. Trepanationen im frühen Mittelalter im Südwesten von Deutschland – Indikationen, Komplikationen und Outcome (in German). Zentralbl Neurochir 2001; 62: 10
4. Greenblatt SH. A History of Neurosurgery: In Its Scientific and Professional Contexts. New York (NY): Thieme, 1997
5. Breasted JH. The Edwin Smith surgical papyrus, published in facsimile and hieroglyphic transliteration with translation and commentary. Chicago: The University of Chicago Press, 1930
6. Chang A. Hippocrates’ influence on the origins of neurosurgery. Neurosurg Focus 2007; 23: E9
7. Missios S. Hippocrates, Galen and the uses of trepanation in the ancient classical world. Neurosurg Focus 2007; 23: E11
8. Bleck TP. Historical Aspects of Critical Care and the Nervous system. Crit Care Clin 2009; 25: 153-64
9. Cushing H. Concerning the results of operations for brain tumor. JAMA 1915; 64: 189-95
10. Poalillo FE. Critical Care in the United States of America. Crit Care Clin 2006; 22: 447-55
11. Celis-Rodriguez E. Critical Care in Latin America: Current Situation. Crit Care Clin 2006; 22: 439-46
12. Ropper AH, Kennedy SF, Zervas NT. Neurological and neurosurgical intensive care. Baltimore (MD): University Park Press; 1983
13. Wartenberg KE, Schmidt JM, Mayer SA. Multimodality Monitoring in Neurocritical Care. Crit Care Clin 2007; 23: 507-38
14. Bullock RM, Chestnut RM, Clifton GL, et al. Part I: Guidelines for the management of severe traumatic brain injury. J Neurotrauma. 2000; 17: 449-554
15. Rumana Ch, Gopinath S, Uzura M, et al. Brain temperature in head injured patients. Paper presented at: 10th International Symposium on Intracranial Pressure and Neuromonitoring in Brain. Injury; May 25-29, 1997; Williamsburg, VA
16. Cordobes F, Lobato RD, Rivas JJ, et al. Observations on 82 patients with extradural hematoma comparision of results before and after the advent of computerized tomography. J Neurosurg 1981; 54: 179-86
17. Jennett B, Bond M. Assessment of outcome after severe brain damage. Lancet 1975;1: 480-4
18. Vincent JL, Moreno R, Takala J, et al; for the Working Group on Sepsis-Related Problems of the European Society of Intensive Care Medicine. The SOFA (Sepsis-related Organ Failure Assessment) score to describe organ dysfunction/failure. Intensive Care Med 1996; 22: 707-10
19. Higgins TL, Teres D, Copes W, et al. Updated mortality probability models (MPM -III). Chest 2005; 128: 348S
20. Pholman TH, Bjerke HS, Offner P. Trauma scoring systems. eMedicine. Jul 16, 2007
21. Annane D, Sebille V, Charpentier C, et al. Effect of treatment with low doses of hydrocortisone and fludrocortisone on mortality in patients with septic shock. JAMA 2002; 288: 862-71
22. Kersten A, Milbrandt EB, Rahim MT, et al. How big is critical care in the US? Crit Care Med 2003; 31(12 Suppl): A8
23. Halpern NA, Bettes L, Greenstein R. Federal and nationwide intensive care units and healthcare costs: 1986-1992. Crit Care Med 1994; 22: 2001-7
24. Angus DC, Barnato AE, Linde-Zwirble WT, et al. Use of intensive care at the end of life in the United States: an epidemiologic study. Crit Care Med 2004; 32: 638-43
25. Angus DC, Shorr AF, White A, et al; Committee on Manpower for Pulmonary and Critical Care Societies (COMPACCS). Critical care delivery in the United States: distribution of services and compliance with Leapfrog recommendations. Crit Care Med 2006; 34: 1016-24
26. Schmitz R, Lantin M, White A. Future needs in pulmonary and critical care medicine. Cambridge (MA): Abt Associates, 1998
27. Angus DC, Kelley MA, Schmitz RJ, et al. Current and projected workforce requirements for care of the critically ill and patients with pulmonary disease: can we meet the requirements of an aging population? JAMA 2000; 284: 2762-70
28. Kelley MA, Angus DC, Chalfin DB, et al. The critical care crisis in the United States: a report from the profession. Chest 2004; 125: 1514-7
29. Center for the Evaluative Clinical Sciences Staff. The Dartmouth atlas of health care 1999. Chicago (IL): American Hospital Publishing, 1999
30. Barnato AE, McClellan MB, Kagay CR, et al. Trends in inpatient treatment intensity among Medicare beneficiaries at the end of life. Health Serv Res 2004; 39: 363-75
31. Centers for Disease Control and Prevention. National Center for Health Statistics. Deaths by place of death, age, race, and sex: United States, 1999-2002. Available at: http://www.cdc.gov/nchs/data/dvs/mortfinal2002_work309.pdf. Last accessed June 2012
32. Provonost PJ, Angus DC, Dorman T, et al. Physician staffing patterns and clinical outcomes in critically ill patients. A systematic review. JAMA 2002; 288: 2151-62
33. Herridge MS, Cheung AM, Tansey CM, et al. One-year outcomes in survivors of the acute respiratory distress syndrome. N Engl J Med 2003; 348: 683-93
34. Angus DC, Carlet J; on behalf of the 2002 Brussels Roundtable Participants. Surviving intensive care: a report from the 2002 Brussels Roundtable. Intensive Care Med 2003; 29: 368-77
35. National Center for Health Statistics. Health, United States, 2003. Hyattsville, MD: National Center for Health Statistics, 2003
36. World Health Staff. The World Health Report 2000: health systems; improving performance. World Health Organization 2000. Available at: http://www.who.int/whr/2000/en/index.html. Last accessed June, 2012
37. Jacobs P, Noseworthy TW. National estimates of intensive care utilization and costs: Canada and the United States. Crit Care Med 1990; 18: 1282-6
38. Reis-Miranda D, Schaufeli WB, vanRossum W, et al. Intensive care units in de landen van de Europese gemeenschap. Medisch Contact 1997; 29/30: 921-5
39. Sirio CA, Tajimi K, Taenaka N, et al. A cross-cultural comparison of critical care delivery: Japan and the United States. Chest 2002; 121: 539-48
40. Garland A. Improving the ICU. Chest 2005; 127; 2151-64
41. Finfer SR, Cohen J. Severe traumatic brain injury. Resuscitation 2001; 48: 77-90
42. Ghajar J, Hariri RJ, Narayan RK, et al. Survey of critical care management of comatose, head-injured patients in the United States. Crit Care Med 1995; 23: 560-7
43. Fakhry SM, Trask AL, Waller MA, et al. Management of brain injured patients by an evidence-based medicine protocol improves. outcomes and decreases hospital charges. J Trauma 2004; 56: 4929. Discussion 499–500
44. Palmer S, Bader MK, Qureshi A, et al. The impact on outcomes in a community hospital setting of using the AANS traumatic brain injury guidelines. Americans Associations for Neurologic Surgeons. J Trauma 2001; 50: 657-64
45. Patel HC, Menon DK, Tebbs S, et al. Specialist neurocritical care and outcome from head injury. Intensive Care Med 2002; 28: 547-53
46. Hesdorffer DC, Ghajar J. Marked Improvement in Adherence to Traumatic Brain Injury Guidelines in United States Trauma Centers. J Trauma 2007; 63: 841-8
47. Broessner G, Helbok R, Lackner P, et al. Survival and long-term functional outcome in 1,155 consecutive neurocritical care patients. Crit Care Med 2007; 35: 2025-30
48. Martin CM, Hill AD, Burns K, et al. Characteristics and outcomes for critically ill patients with prolonged intensive care unit stays. Crit Care Med 2005; 33: 1922-7
49. Carlson R, Weiland DE, Srivathsan K. Does a full-time 24 hour intensivist improve care and efficiency? Crit Care Clin 1996; 12: 525-51
50. Health Care Financing Administration: http://www.hcfa.com. Last accessed June 2012
51. Zakaria A, Provencio JJ, Lopez GA. Emerging Subspecialties in Neurology: Neurocritical care. Neurology 2008; 70: e68-e69
52. Pressman HT. Traumatic brain injury rehabilitation: case management and insurance-related issues. Phys Med Rehabil Clin N Am 2007; 18: 165-74
53. Lustbader D, Fein A. Emerging trends in ICU management and staffing. Crit Care Clin 2000; 16: 735-48
54. Panayiotis N, Varelas, Eastwood D. Impact of a neurointensivist on outcomes in patients with head trauma treated in a neurosciences intensive care unit. J Neurosurg 2006; 104: 713-9
55. Pollack MM, Katz RW, Ruttimann UE, et al. Improving the outcome and efficiency of intensive care: the impact of an intensivist. Crit Care Med 1988; 16: 11-7
56. Blunt MC, Burchett KR. Out-of-hours consultant cover and case-mix-adjusted mortality inintensive care. Lancet 2000; 356: 735-6
57. Morales IJ, Peters SG, Afessa B. Hospital mortality rate and length of stay in patients admitted at night to the intensive care unit. Crit Care Med 2003; 31: 858-63
58. Mitchell-DiCenso A, Guyatt G, Marrin M, et al. A controlled trial of nurse practitioners in neonatal intensive care. Pediatrics 1996; 98: 1143-8
59. Baggs JG, Schmitt JH, Mushlin AI, et al. Association between nurse-physician collaboration and patient outcomes in three intensive care units. Crit Care Med 1999; 27: 1991-8
60. Derengowski SL, Irving SY, Koogle PV, et al. Defining the role of the pediatric critical care nurse practitioner in a tertiary care center. Crit Care Med 2000; 28: 2626-30
61. VanSoeren MH, Kirby AS, Andrusyszyn M. Lessons learned during implementation of an acute-care nurse practitioner role in adult critical care in Ontario. Ann R Coll Physicians Surg Can 2002; 35: 556-62
62. Snyder JV, Sirio CA, Angus DC, et al. Trial of nurse practitioners in intensive care. New Horiz 1994; 2: 296-304
63. Vincent JL, Bihari DJ, Suter PM, et al. The prevalence of nosocomial infection in intensive care units in Europe. Results of the European Prevalence of Infection in Intensive Care (EPIC) Study. EPIC International Advisory Committee. JAMA 1995; 274: 639-44
64. U. S. Department of Health & Human Services, Health Resources and Services Administration, Bureau of Health Professions. Division of Nursing. The registered nurse population: national sample survey of registered nurses preliminary findings. Rockville, MD: U. S. Government Printing Office, 2000
65. Snyder JV, Sirio CA, Angus DC, et al. Trial of nurse practitioners in intensive care. New Horiz 1994; 2: 296-304
66. Jones PE, Cawley JF. Physician assistants and health system reform: Clinical capabilities, practice activities, and potential roles. JAMA 1994; 271: 1266-72
67. Combs RD. The role of physician assistants in critical care. In: Green TP, Carcillo JA (eds). Technique and Technology: Society of Critical Care Medicine 28th Educational and Scientific Symposium. Anaheim, CA: Society of Critical Care Medicine, 1999, pp. 109-12
68. Wagner AK, Fabio T, Zafonte RD, et al. Physical medicine and rehabilitation consultation: relationships with acute functional outcome, length of stay, and discharge planning after traumatic brain injury. Am J Phys Med Rehabil 2003; 82: 526-36
69. Patterson P, Maynard H, Chesnut R, et al. Evidence of traumatic case-management effect on traumatic brain injured adults in rehabilitation. Care Manag J 1999; 1: 87-97
70. Ashley M, Lehr R, Krych D, et al. Post-acute rehabilitation outcome: Relationship to case-management techniques and strategy. J Insur Med 1994; 26: 348-54
71. Malec J, Buffington M, Moessner A, et al. Subspecialty clinics: Physical medicine and rehabilitation. Mayo Clinic Proceedings 1995 70; 1165-71
72. Ligtenberg J, Arnold G, Stientstra Y, et al. Quality of interhospital transport of critically ill patients: a prospective audit. Critical Care 2005, 9: R446-R451
73. Fromm R, Varon J. Critical Care Transport. Crit Care Clin 2000; 16: 695-705
74. Seymour C, Kahn J, Schwab W, et al. Adverse events during rotary-wing transport of mechanically ventilated patients: a retrospective cohort study. Critical Care 2008, 12: R71
75. AAVV. Trauma Systems and the Neurosurgeon. Brain Trauma Foundation. J Neurotrauma 1996; 13: 647-51
76. Daly BJ, Rudy EB, Thompson KS, et al. Development of a special care unit for chronically ill patients. Heart Lung 1991; 20: 40
77. Jastremski C. ICU bedside environment. A nursing perspective. Crit Care Clin 2000; 16: 723-34
78. Kholi-Seth R, Oropello JM. The future of bedside monitoring. Crit Care Clin 2000; 16: 557-78
79. Taylor W, Chen JW, Meltzer H, et al; Quantitative pupillometry, a new technology: normative data and preliminary observations in patients with acute head injury. J Neurosurg 2003; 98: 205-13
80. Xu J, Rasmussen IA, Lagopoulos J, et al. Diffuse Axonal Injury in Severe Traumatic Brain Injury Visualized Using High-Resolution Diffusion Tensor Imaging. J Neurotrauma 2007; 24: 753-65
81. Breslow MJ, Rosenfeld BA, Doerfler M, et al. Effect of a multiple-site intensive care unit telemedicine program on clinical and economic outcomes: an alternative paradigm for intensivist staffing. Crit Care Med 2004; 32: 31-8
82. Rosenfeld BA, Dorman T, Breslow MJ, et al. Intensive care unit telemedicine: alternate paradigm for providing continuous intensivist care. Crit Care Med 2000; 28: 3925-31
83. Brophy G, Pineda J, Papa L, et al. aII-Spectrin Breakdown Product Cerebrospinal Fluid Exposure Metrics Suggest Differences in Cellular Injury Mechanisms after Severe Traumatic Brain Injury. J Neurotrauma 2009; 26: 1-9
84. Lewis S, Velat G, Miralia L, et al. Alpha-II spectrin breakdown products in aneurismal subarachnoid hemorrhage: a novel biomarker of proteolytic injury. J Neurosurg 2007; 107: 792-6
85. Kochanek P, Berger R, Bayir H, et al. Biomarkers of primary and evolving damage in traumatic and ischemic brain injury: diagnosis, prognosis, probing mechanisms, and therapeutic decision making. Curr Opin Crit Care 2008; 14: 135-41
86. Robertson CS. Neuroscience (Editorial Comment). Curr Opin Crit Care 2008; 14: 127-8
87. Maas A. Traumatic brain injury: simple data collection will improve the outcome. Wien Klin Wochenschr 2007; 119: 20-2
88. Beretta L, Aldrovandi V, Grandi E, et al. Improving the quality of data entry in a low-budget head injury database. Acta Neurochir (Wien) 2007; 149: 903-9
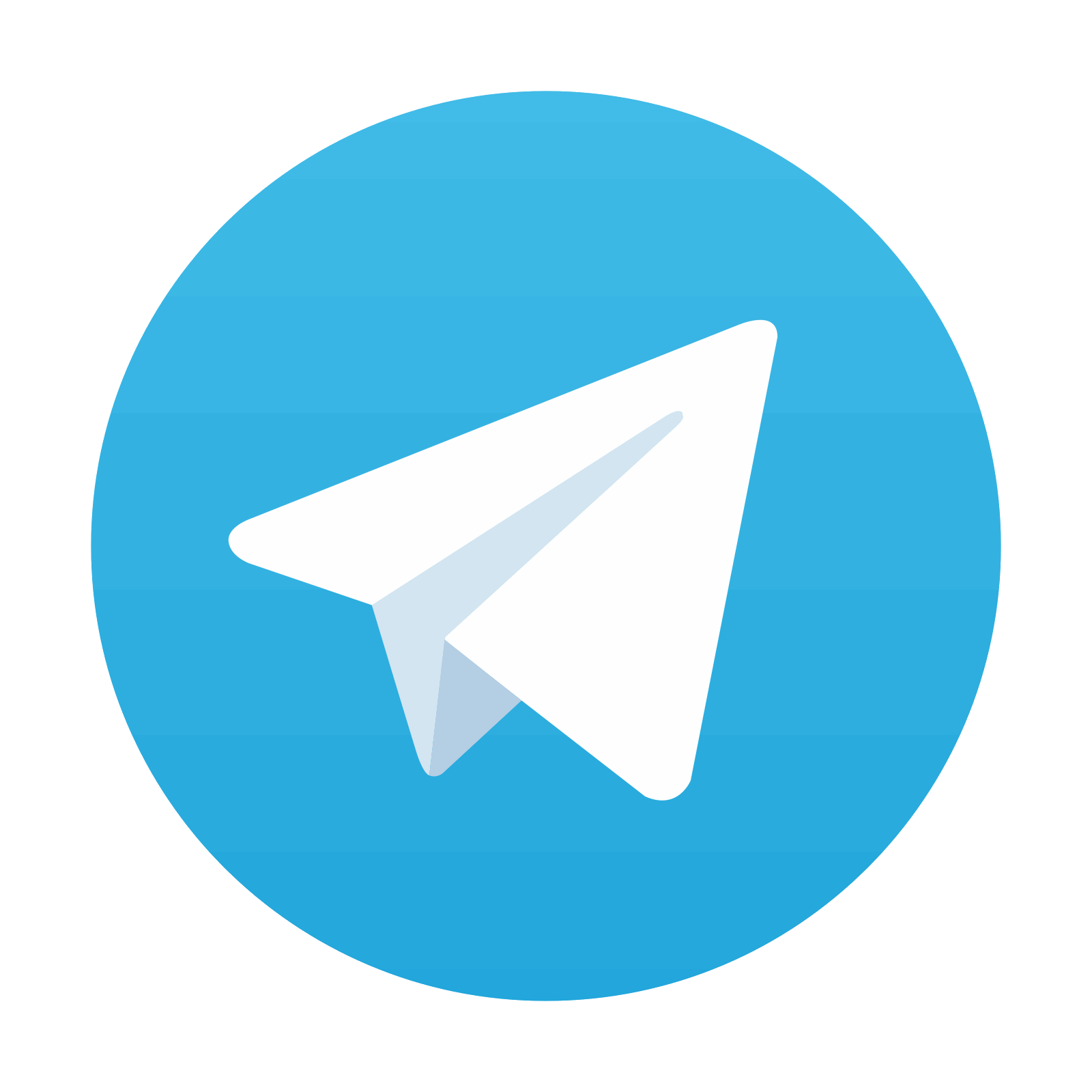
Stay updated, free articles. Join our Telegram channel

Full access? Get Clinical Tree
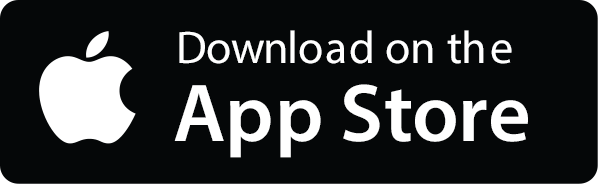
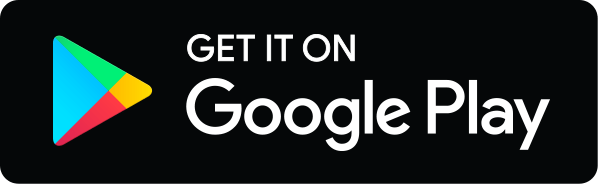