Figure 20.1. Schematic representation of the reactions to injury.
20.3 Assessment of Nutritional Status
The use of artificial nutrition has generated the need for an effective method to assess nutritional status in hospitalized patients. Because there is no simple method to define nutritional status, it is very important to have a comprehensive approach that includes careful medical history taking and anthropometric measurement, coupled with laboratory biochemical studies that can provide data about functional proteins and the patient’s immune status as well.
The first step in assessing nutritional status is the clinical examination, as it will usually reveal elements that may indicate or suggest the presence of malnutrition. There are well-defined manifestations of a specific nutritional deficit; however, combined or partial clinical syndromes are most often observed. The classic malnutrition symptoms or signs are shown in Table 20.1. Other types of malnutrition are related to the lack of specific nutrients (electrolytes, minerals, oligoelements and vitamins). This lack can be isolated or combined and is often associated with the aforementioned disorders, which is why it may be masked by clinical manifestations.
A complete clinical examination includes evaluation of quantitative data obtained from objective and comparable studies which can be grouped as anthropometric and biochemical (biological indicators) measurement, immune status, and the nutritional prognostic index.
Malnutrition type | Marasmus (protein-energy malnutrition) | Kwashiorkor (protein malnutrition) | Mixed |
Muscular mass | Very decreased | A little changes | Decreased |
Visceral protein | A little changes | Very decreased | Decreased |
Edema | Absent | Present | Present |
Lymphocytes count | Decreased | Decreased | Decreased |
Skin tests | Non-reactive | Non-reactive | Non-reactive |
Table 20.1. Malnutrition. Classic types.
20.4 Anthropometric Measurement
Body weight is a simple guide to the patient’s nutrition status, although it expresses the nature of tissue loss and may have errors of interpretation when there is dehydration or edema. Because this parameter alone has relatively little value, other ways to assess body weight have been described.
Current weight as the ideal weight percentage can be calculated as follows:
% ideal body weight = actual weight x 100/ideal weight
Values are:
- Normal >90%.
- Mild malnutrition 80-89%.
- Moderate malnutrition 70-79%.
- Severe malnutrition <70%.
Current weight as usual weight percentage is equal to:
% usual weight = current weight x 100/usual weight
Values are:
- Normal >95%.
- Mild malnutrition 85-94%.
- Moderate malnutrition 75-84%.
- Severe malnutrition <75%.
Modified body weight or recent weight change is equal to:
% modified body weight = (usual weight – current weight) x 100/normal weight
Weight loss >10% indicates malnutrition, but even minor losses are reason for concern.
Triceps skinfold provides an estimate of subcutaneous fat which accounts for almost half of the body fat. It should be taken in the not-dominant arm (usually left) with the patient in the standing position or sitting with the arm hanging next to the body. If the patient is lying down, the arm should be placed with the forearm flexed over the chest. Measurement is taken at the midpoint between the acromion and the olecranon on the back of the arm. The fold thickness is measured with a Lange caliper or another type of instrument. A pressure of about 10 g/mm3 should be applied, taking three measurements and then calculating the average value.
Standard values are 12.5 mm in men, and 16.5 mm in women. The obtained measurement is compared with the standard value using the formula:
% standard value = current measurement x 100/standard measurement
Values >80% indicate that adipose tissue is preserved; values <80% indicate loss of body fat. It is a useful index for assessing the degree of malnutrition, since changes in subcutaneous fat happen slowly.
Upper arm circumference is an indicator of muscle mass. Measurement should be taken in the half of the extended arm, at the midpoint between the spinous process of the acromion and the olecranon. The values can be compared using percentile tables or compared with the standard values using the formula:
% standard value = current measurement x 100/standard measurement
The reference values are 29.3 cm in men and 28.5 cm in women.
Values <85% indicate malnutrition.
Arm muscle mass is used to estimate body muscle mass, indicating protein reserves.
The formula is:
Arm muscle mass = upper arm circumference (cm) – 0.31416 x triceps skinfold (mm)
The current measurement is compared with standard values (25.3 cm in men and 23.2 cm in women):
% standard value = current measurement x 100/ standard measurement
Values >80% indicate adequate muscle mass; values <80% indicate protein mass deficit.
Body height is obtained by dividing the height (cm) and the wrist circumference (cm) at the level of the styloid process.
Body height = height (cm)/wrist circumference (cm)
Values in men are:
- Normal <9.6.
- Light malnutrition 9.6-10.4.
- Moderate malnutrition >10.4.
Values in women are:
- Normal <9.9.
- Light malnutrition 9.9-10.9.
- Moderate malnutrition >10.9.
Creatinine-height index is an estimate of muscle mass because creatinine is formed from creatine only in the muscle. In patients with normal renal function this value is relatively constant for a given weight and height. This index is defined as the relationship between urinary creatinine excretion over 24 hours and the expected urinary excretion in a normal adult of the same size.
Creatinine-height index: = current urinary creatinine x 100/ideal urinary creatinine
The ideal urinary creatinine is obtained from tables (Table 20.2).
Men | Women | ||
Height (cm) | Creatinine (mg/dl) | Height (cm) | Creatinine (mg/dl) |
157.5 | 1288 | 147.3 | 830 |
160.0 | 1325 | 149.9 | 851 |
162.6 | 1359 | 152.4 | 875 |
165.1 | 1386 | 154.9 | 900 |
167.6 | 1426 | 157.5 | 925 |
170.2 | 1467 | 160.0 | 949 |
172.7 | 1513 | 162.6 | 977 |
175.3 | 1555 | 165.1 | 1006 |
177.8 | 1596 | 167.6 | 1044 |
180.3 | 1642 | 170.2 | 1076 |
182.9 | 1691 | 172.7 | 1109 |
185.9 | 1739 | 175.3 | 1141 |
188.0 | 1785 | 177.8 | 1174 |
190.5 | 1831 | 180.3 | 1206 |
193.0 | 1891 | 182.9 | 1240 |
Table 20.2. Urinary creatinine values according to sex and height.
Creatinine-height index values <60% indicate a severe depletion of somatic proteins; values between 60 and 80% indicate a moderate depletion.
Errors in anthropometric measurement are due to methodological inaccuracies. Some authors argue that there is an insufficient correlation between these measurements and body nitrogen.
20.5 Biochemical Measures (Biological Indicators)
20.5.1 Estimated Somatic Protein Mass
Excretion of urinary 3-methylhistidine (3-me-his): 3-methylhistidine is formed from histidine methylation and is found only in the myofibre protein in the muscle. During muscle breakdown, actin and myosin proteins are released, but as they cannot be reused for protein synthesis, they are excreted with the urine, which provides an index of muscle catabolism. Each gram of muscle protein releases 4.2 micromoles of 3-methylhistidine.
Values depend on the pathological situation:
- Simple fasting <100 µmol/24 hours.
- Elective surgery 130±20 µmol/24 hours.
- Trauma 200±20 µmol/24 hours.
- Sepsis 450±50 µmol/24 hours.
20.5.2 Creatinine Urinary Excretion
Creatinine is produced by creatine metabolism. In patients with normal renal function, urinary excretion correlates with the total organic creatinine value.
Normal values: 23 mg/kg/day in men and 18 mg/kg/day in women.
These values vary according to renal function and nutritional intake of the patient.
20.5.3 Estimated Visceral Protein Mass
It refers to the measured plasma proteins synthesized by the liver.
Albumin, the main protein produced by the liver, maintains plasma oncotic pressure and is involved in the transport of various substances. It is not a good indicator of malnutrition, since it has a long half-life (16-21 days) and its concentrations are reduced and recovered very slowly during changes in nutritional status.
Values are:
- Normal 35 g/l.
- Mild depletion 30-34 g/l.
- Moderate depletion 25-29 g/l.
- Severe depletion <25 g/l.
Albumin levels <28 g/l lead to the development of edema because of the decrease in plasma oncotic pressure.
Prealbumin, a protein involved in the transport of thyroxine, has a half-life of 2 days and is present in a small pool in the body which decreases quickly with any sudden demand for protein synthesis, as in trauma or acute infection. It is useful as a short-term nutritional index.
Values are:
- Normal 15-30 mg/dl.
- Mild depletion 10-14 mg/dl.
- Moderate depletion 5-9 mg/dl.
- Severe depletion <5 mg/dl.
Transferrin (TFN), a betaglobulin synthesized in the liver, conjugates the iron in plasma. Its half-life (t ½) is 8 to 10 days. It belongs to the group of acute phase reactive proteins and shows more precisely the changes in acute visceral proteins (depletion and repletion) due to its short half-life and small pool. It can be measured by radial immunodiffusion or calculated from total iron binding capacity (TIBC) with the following formula:
TFN = (0.8 x TIBC) – 43
Values are:
- Normal 250-400 mg/dl.
- Mild depletion 150-250 mg/dl.
- Moderate depletion 100-150 mg/dl.
- Severe depletion <100 mg/dl.
Retinol binding protein, a specific protein that transports vitamin A alcohol is bound to prealbumin. Since it is filtered by the glomerulus and excreted by the kidney, it rises in renal disease. It is a sensitive index of subclinical malnutrition and a good responder to nutritional recovery because it breaks down within about 10 to 12 hours.
Normal range is 2.6-7 mg/dl.
Fibronectin is a protein with a half-life of less than 2 days. It has a very important relevance, more than other proteins, and is also used in monitoring the patient.
Values are:
- Normal range 320-360 mg/dl.
- Mild depletion 230-319 mg dl.
- Moderate depletion 200-229 mg/dl.
- Severe depletion <200 mg/dl.
Values <200 mg/dl are associated with increased mortality.
Techniques for measuring visceral proteins do not provide an accurate assessment of nutritional status, as they are influenced by factors other than malnutrition. There is a direct correlation between albumin, transferrin and fibronectin depletion with higher morbidity and mortality, delayed healing and decreased resistance to infection.
20.5.4 Measurement of Immune Response
Immune function is reduced in malnutrition, which is associated with higher morbidity and mortality from infectious diseases.
Decreased total lymphocyte count (TLC) is related to depressed cellular immunity, according to the following formula:
TLC = % lymphocytes x total white blood cells/100
Values are:
- Normal >2000/mm3.
- Mild immune deficiency 2000-1200/mm3.
- Moderate immune deficiency 1200-800/mm3.
- Severe immune deficiency <800/mm3.
Skin tests indicate the cell-mediated immune state; to perform it are used antigens as the purified protein derivative antigens (PPD), Candida, mumps, histoplasma, and streptokinase-streptodornase and trichophytum. Intradermal hives appear on the anterior forearm when 0.1 ml of each of the antigens is given. Reading should be performed at 24 to 48 hours.
Values are:
- Positive or reactive: wheal >5 mm.
- Negative or non-reactive: wheal <5 mm.
A patient is considered immunocompetent when he has a good response (>5 mm) to two or more antigens. Anergy is defined as negative reaction to all antigens. These tests are less useful in patients with cancer, cirrhosis or in those under immunosuppressive therapy, because they may be incompetent due to the immunological condition itself, even when nutritional status is adequate.
20.5.5 Nutritional Prognostic Indexes (NPI)
These indices are very important to establish a relationship between nutritional status, as evaluated by objective parameters (anthropometric, biochemical or immune status) and morbidity and mortality. The index described by Buzby is derived by the formula:
NPI = 158 to 16.6 (Alb) – 0.78 (TS) – 0.20 (ST) – 5.8 (DHR)
where: Alb = serum albumin (g/l); TS = triceps skinfold (mm); ST = serum transferrin (mg/dl); DHR = delayed hypersensitivity response.
Results are:
- No reaction = 0.
- Induration <5 mm = 1.
- Induration >5 mm = 2.
The result indicates the probability of occurrence of complications due to malnutrition, the most important of which is sepsis. Based on this index, several authors have applied multiple linear regression analysis using two or three variables which showed good correlation with severe complications and mortality, thereby generating various indexes, the most useful of which in our practice is:
NPI (Alb-DHR) = 55 to 10.2 (Alb) – 9 (DHR)
DHR is used as the skin response to five antigens, scored as 1 when it is normal and 0 if there is anergy.
The values associated with a greater probability of complications are:
- NPI (Buzby) >40.
- NPI (Alb-DHR) >18.
20.5.6 Energy Expenditure
Living organisms need energy for all sorts of biological activities such as the synthesis of cellular material, transport of substances against a concentration gradient, muscle contraction, and production of heat to maintain body temperature constant. Nutrients (carbohydrates, fats and proteins) are transformed to provide the energy needed for body functions. When energy intake exceeds consumption, the energy is stored, resulting in weight gain. Conversely, when energy expenditure exceeds intake, the deficit is made up for by the body’s reserves, resulting in weight loss.
Basal energy expenditure (BEE), also known as basal metabolic rate (BMR) or basal metabolic index (BMI), refers to the production of energy per unit of time in basal conditions, defined as physical and mental rest, soon after awakening in the post-absorptive stage (about 12 hours after the last meal) and normal body temperature (18-26°C). This is the minimum energy consumption during physiological conditions. The liver, brain, heart and kidney consume 60% to 70% of BEE, although they account for only 5% to 6% of body weight, which reflects their high metabolic activity. In contrast, the muscles constitute 40% of body weight and use only 18% of BEE, depending on the activity of respiratory muscles and those required to maintain posture. BEE depends on cellular processes such as ion pump, synthesis and degradation of cellular constituents in which the protons cross the mitochondrial membrane, and also respiratory muscle and myocardial contraction. BEE is generally between 1500 and 1800 kcal/day and can be predicted by several methods (Table 20.3).
Body weight (kg) | BEE (kg Kcal/day) |
50 | 1316 |
55 | 1411 |
60 | 1509 |
65 | 1602 |
70 | 1697 |
75 | 1784 |
80 | 1872 |
Table 20.3 Normograms.
Harris-Benedict formula:
BEE (men) = 66.5 + 13.75 (W) + 5 (H) – 6.75 (A)
BEE (women) = 65.5 +9.56 (P) +1.84 (H) – 4.7 (A)
where: W = weight (kg), H = height (cm), A = age (in years).
BEE is expressed in kcal/kg. This formula has the advantage that it can be rapidly calculated and is easy to apply, although errors of up to 30% of total calories have been reported.
Resting energy expenditure (REE) refers to the energy expenditure at basal conditions. The difference between BEE and REE derives from food-specific dynamic actions and thermogenic response, which is the increased energy expenditure caused by nutrition itself. REE is equal to BEE increased by 10%. It is reported that the REE of patients with traumatic brain injury is 140% on average. Barbiturates, propranolol and muscle relaxants can decrease hypermetabolism, although this has not proved to influence the prognosis.
Total energy expenditure (TEE) refers to the caloric intake to be administered to maintain body weight. It is usually obtained from BEE, and it takes into account the degree of catabolism associated with the disease or injury.
Different forms of production have been described:
TEE (Rutten) = BEE x 1.75
This formula is unreliable because it does not adequately reflect the involvement of patient’s physical activity or increased activity in situations of stress.
TEE (Long) = BEE x activity factor x stress factor
The activity factor (AF) corresponds to the degree of patient mobilization. In critically ill bedridden patients it is 1.20 (20% increase in BEE) and 1.30 in awake patients. Some authors use an average of 1.25 in all situations. This factor does not take into account paralyzed patients or ventilated patients under muscle relaxation. In mobile patients, one must use expenditure energy (EE) tables which link it with the type of activity. The stress or aggression factor (AF) corrects the EE for different conditions and is obtained from tables that have been created for this purpose. Table 20.4 describes the one used in our unit.
Clinical conditions | Correction factors |
Prolonged fasten | 0.85-1.00 |
Non complicated postoperatory period | 1.00-1.05 |
Fever* | 1.10-1.13 |
Peritonitis | 1.05- 1.25 |
ARDS | 1.20 |
Long bone fracture | 1.15-1.30 |
Infection: | |
Mild | 1.20 |
Moderate | 1.30 |
Severe | 1.50-1.60 |
Head trauma | 1.60 |
Burns | |
10 to 30 % body mass | 1.50 |
30 to 50 % body mass | 1.75 |
More than 50 % body mass | 2.00 |
Table 20.4. Correction factors in different clinical conditions.
* For each temperature grade above 37oC or fever lasting more than 24 hours, increase occurs.
Another way to calculate TEE is by indirect calorimetry, which determines the amount of energy lost as body heat. This method measures the amount of energy expenditure of all metabolically active cells in the body, which is equal to TEE.
To calculate TEE by this method, one must know the concentration of inspired (FiO2) and expired oxygen (FeO2), inspired and expired CO2 fractions (FiCO2 and FeCO2, respectively), inspired and expired minute volume (IMV and EMV), CO2 production (VCO2) and the respiratory quotient (RQ). This is done using the following formulas:
VO2 (l/day) = FiO2 x IMV – FeO2 x EMV
and
VCO2 (l/day) = FeCO2 x EMV – FiCO2 x IMV
Using these parameters, the Weir formula allows the calculation of TEE with three levels of accuracy.
Using only VO2 in the formula TEE = 4.83 x VO2, the energy expenditure can be estimated with a minimum of accuracy. The combined use of VO2 and VCO2 increases the level of accuracy and allows the calculation of respiratory quotient (RQ):
RQ (TEE) = 3.9 x VO2 + 1.1 x VCO2
Finally, the incorporation of protein metabolic factor into the evaluation of blood gas production and consumption allows for greater accuracy and to determine the oxidation of each nutrient separately.
TEE = 3.94 x VO2+ 1.1 x VCO2 – 2.17 x No
Where No denotes urinary nitrogen.
It has been found that the exclusion of No generates an error of less than 25% in calculating TEE. Determining the respiratory quotient (RQ) is valuable for estimating the nutrients that are mainly oxidized. The nonproteic physiological RQ ranges from 0.71 to 1.2, which indicates the oxidation of fats and carbohydrates; when it is about 0.7, it depends only on the oxidation of fat; if around 1, it indicates that only carbohydrates are oxidized; and when greater than 1, this suggests that the carbohydrate supply is excessive and lipogenesis is occurring.
The difficulties with this method are that VO2 and VCO2 vary constantly; therefore, computers are used to process and analyze the data every minute to obtain a 24-hour average.
The combustion characteristics of each nutrient are related to its chemical structure. Table 20.5 presents the energy characteristics of different nutrients.
Nutrient | Kcal/g | VCO2 (l/g) | VO2 (l/g) | RQ | Caloric value per liter of O2 | |
Carbohydrates | Monosaccharides | 3.75 | 0.75 | 0.75 | 1 | 5 |
Polysaccharides | 4.30 | 0.83 | 0.83 | 1 | 5 | |
Proteins | 4.20 | 0.75 | 0.94 | 0.80 | 4.5 | |
Fats | 9.30 | 1.39 | 1.96 | 0.71 | 4.7 | |
Ethanol | 7.10 | 0.98 | 1.46 | 0.67 | 4.9 | |
Ordinary diet | – | – | – | 0.82 | 4.8 |
Table 20.5. Energetic value for different nutrients.
VO2 = oxygen consumption; VCO2= carbon dioxide production; RQ = respiratory quotient.
20.6 Nutritional Requirements
The energy requirements of different essential elements, as well as vitamins, minerals and water, vary in the hypercatabolic and critically ill patient in relation to the normal patient.
Energy requirements. The patient’s energy needs are equivalent to TEE and determine the caloric intake to be provided to maintain body weight. If the objective is to achieve anabolism and weight gain, 1000 kcal should be added to the calculated value, which will increase a weight gain of approximately 1 kg or 2 pounds per week. Normometabolic patients must receive at least 25% of TEE as carbohydrates to save proteins. Since stressed patients have a higher need of carbohydrates to save proteins and maintain high-energy deposits in the tissues, at least 50% of TEE should be supplied as carbohydrates. When carbohydrates are used as the sole source of energy, the needs are around 500 up to 650 g/day. Total carbohydrates should be calculated at a rate of 2 g/kg/day in normal patients and 4-6 g/kg/day in hypercatabolic conditions.
The use of a high caloric intake based exclusively on carbohydrates can lead to essential fatty acid deficiency, liver dysfunction with elevated enzymes, inhibiting the synthesis of very low density lipoprotein (VLDL), increased hepatic triglyceride synthesis, decreased lung surfactant production and increased CO2 production.
To avoid problems caused by the use of carbohydrates as the sole source of energy, it is recommended to associate them with fats, which have multiple functions, as well as providing metabolic energy reserve in the adipocytes. Also, phospholipids are formed in the cell membrane and act as intracellular modulators (prostaglandins). Lipids can be used two times per week, with the aim of providing essential fatty acids (linoleic acid), or administered as an energy source, thus supplying some of the calories calculated. The daily fat requirement is 1 to 2 g/kg/day under basal metabolic conditions, which is higher in catabolic situations (3-5 g/kg/day).
The proportion of carbohydrates and fats to be administered varies according to different sets of criteria and the patient’s illness. Some authors recommend using no more than 60% of nonprotein calories as fat, stating it should not exceed 100 g/day, although some set the limit as high as 75% of TEE.
Fat emulsions are not well tolerated in certain clinical situations, such as Gram-negative sepsis, neonatal hyperbilirubinemia and carbohydrate and lipid metabolic disorders.
Studies published in the 1960s reported on the need to differentiate long-chain triglycerides (LCT) from medium-chain triglycerides (MCT), highlighting the benefit of the latter because of their high caloric value, similar clearance and metabolism to glucose.
LCT emulsions of fatty acids containing 12 to 24 carbons undergo hydrolysis to fatty acids and glycerol in the intestinal lumen, which are esterified after being re-absorbed to form triglycerides that bind to proteins and phospholipids, forming chylomicrons. They then enter the lymphatic system and finally the blood, where they are distributed to the tissues.
MCT emulsions of fatty acids containing 6 to 12 carbons, mainly octanoic acid, esterified cholesterol are hydrolysed in the intestine five times faster than LCT (although some authors note that they do not need to be absorbed hydrolyzed) and their free fatty acids are absorbed twice faster. They then go directly to the portal vein and are transported to the liver and other tissues as free fatty acids or bound to albumin. They have the advantage of not being stored as fat in the body and are oxidized quickly and completely.
The use of LCT as the sole source of fat produces lipid deposition in the heart, kidneys and lungs, because less than 10% are oxidized and the rest is stored as neutral fat.
The use of MCT alone delivers more calories more rapidly than LCT. MCT are also associated with decreased fatty infiltration but do not prevent essential fatty acid deficiency. At present, the combined use of LCT and MCT is considered most advantageous; therefore, LCT solutions with 25 to 50% MCT are recommended.
In enteral nutrition, MCT should be introduced in small amounts, increasing them gradually to a daily intake of 50 to 100 g.
MCTs are ketogenic, so they are contraindicated in diabetic patients or in those with ketosis and acidosis, in which the capacity of extrahepatic tissues to use ketone bodies is saturated. They should not be used in patients with liver cirrhosis, as the medium-chain fatty acids are metabolized in the liver and liver function is reduced. Octanoic acid accumulates in these patients, so symptoms may appear similar to hepatic encephalopathy.
Protein requirements. In the body there is usually a loss of protein due to the synthesis and degradation of body proteins. The main protein losses occur through the urine and feces, the latter due to intestinal secretions and cell desquamation. In addition, there is minimal loss through the skin and during menstruation in women.
In healthy patients there is a positive nitrogen balance when nonprotein calories are provided for each gram of nitrogen (300-350/1) (7% of TEE). In hypercatabolic and critically ill patients, because urinary nitrogen elimination is increased, the patient needs more protein in relation to the total calories, as protein should comprise about 10 to 20% of TEE. In this particular situation, a ratio of nonprotein calories per gram of nitrogen (100 to 150/1) must be maintained. In septic patients this ratio should be 80 kcal of nonprotein nitrogen per gram of nitrogen.
In patients with acute brain injury, the estimated daily intake of nitrogen should be between 0.3 and 0.5 grams of nitrogen per kilogram of body weight per day.
Protein requirements can be calculated in the ways described below.
From 10 to 20% of TEE as proteins: it must be remembered that each gram of protein provides 4 kcal and that with each 6.25 g of protein 1 g of nitrogen is released (1g of protein is equivalent to 0.16 g of nitrogen).
Using tables that show the daily losses of nitrogen in different clinical situations (replacement must equal losses).
Measured urea in 24-hour urine: nitrogen excretion in urine is the final product of protein metabolism to obtain energy; therefore, the protein requirements can be determined by calculating the daily losses of nitrogen from the elimination of urea in 24-hour urine. For the proper calculation of nitrogen excretion, a steady diet should maintained for 24 to 48 hours beforehand.
Urea in 24 – hour urine (g/24 hours) = urine volume (l/24 hours) x urea in urine (g/l)
The urea molecule is not entirely composed of nitrogen. To obtain the real amount of nitrogen that it contains, it must be divided by 2.14.
UUN (g/24 hours) = urea in 24 – hour urine (g/24 hours) / 2.14
Where urinary ureic nitrogen (UUN) represents 80% of total urinary nitrogen losses (total urinary nitrogen or TUN). The latter can be calculated when 20% of UUN is added to itself.
TUN = UUN + 20% UUN
Added to the obtained value must be 2 to 4 g due to losses by the skin and feces, although these values may be higher in patients with diarrhea, burns, and extensive wounds or diffuse exfoliative dermatitis.
Calculation from TEE, using the desired ratio: nonprotein calories per gram of nitrogen.
When using amino acids as a nitrogen source, some measures should be taken into account:
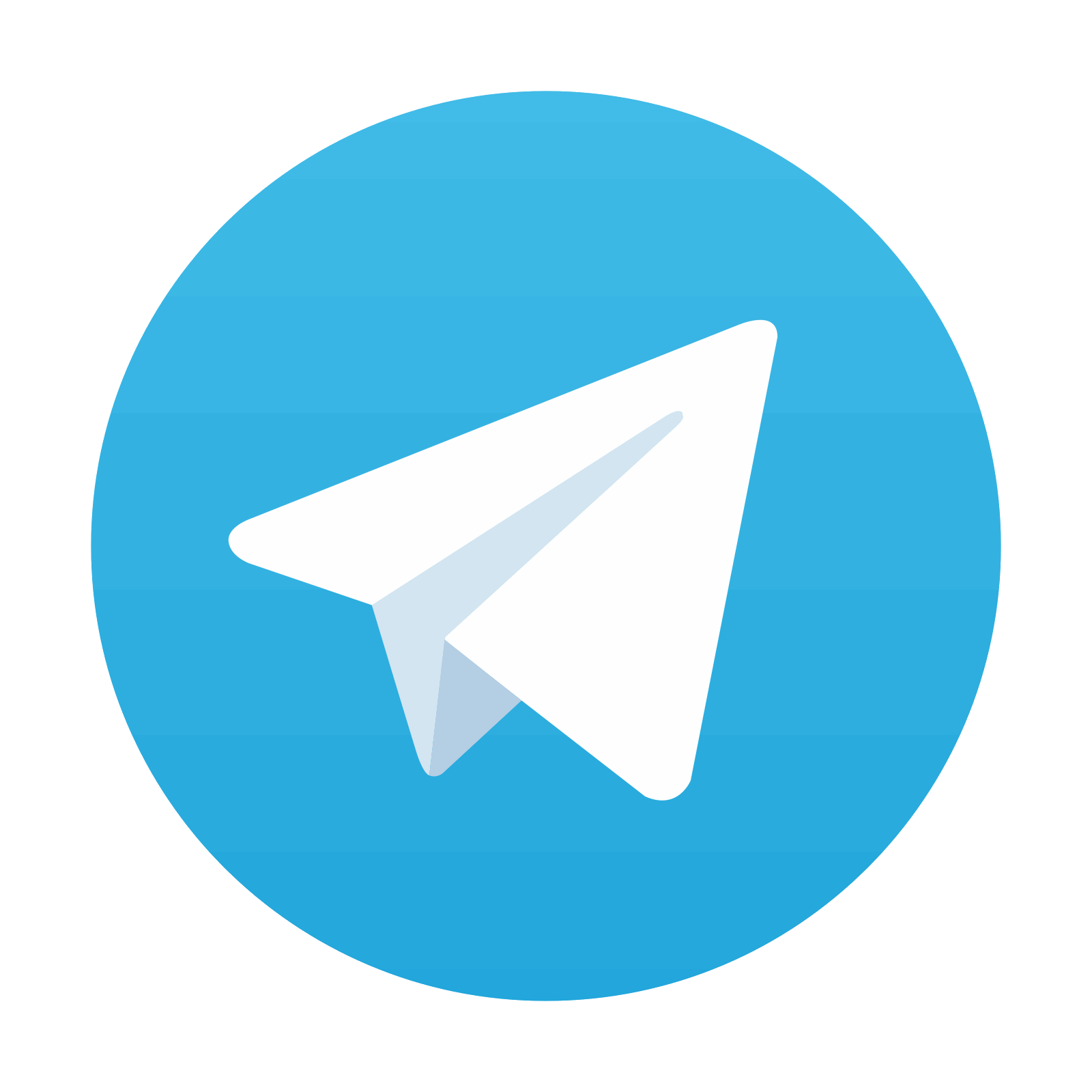
Stay updated, free articles. Join our Telegram channel

Full access? Get Clinical Tree
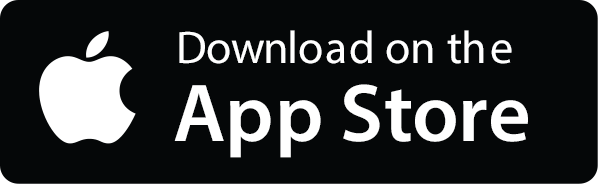
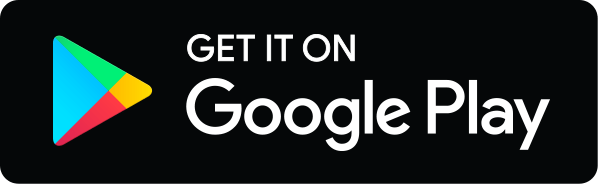