18 Oculomotor System This chapter discusses the ocular motor system. It is organized into six sections: (1) a description of the four types of eye movements, including saccades, smooth pursuit, vestibular-optokinetic, and vergence movements; (2) a discussion of the anatomy and disorders of the supranuclear control system; (3) a discussion of the anatomy and localization of lesions involving the oculomotor nerves; (4) a description of the clinical features and anatomic localization of nystagmus; (5) a description of the anatomy and disorders of pupillary function; and (6) a discussion of the anatomy and disorders of the eyelid. See Fig. 18.1. The major function of saccadic eye movements is to rapidly place the object of interest on the fovea. Saccadic eye movements may be volitional (voluntary refixations) or involuntary (quick phases of vestibular and optoki-netic nystagmus). The stimulus required to elicit a saccade consists of an object of interest in the peripheral eye field. From stimulus to onset of eye movement, the latency of saccadic eye movements is ~200 msec; a typical velocity is ∼400 degrees/s (but may be as high as 600 to 700 degrees/s.) One hypothesis suggests that saccadic movements are ballistic; that is, they are not modifiable once begun. In support of this hypothesis, it is argued that visual information is sampled by the saccadic system. Once an object of interest is identified in the visual periphery, a decision is made to generate a saccade that will bring the object into focus on the fovea. Depending on the retinal error (i.e., the distance between the retinal location of an image and the fovea), the size, direction, and duration of a saccade are automatically calculated, and an irrevocable decision is made to generate the saccade. Once the saccade is completed, the visual world is again sampled, and the process is then repeated. The anatomic substrate for volitional saccades originates in the frontal eye fields (Brodmann’s area 8), which can initiate saccades in the contralateral direction, via descending pathways to the superior colliculi. The superior colliculus receives an orderly retinal projection such that the visual field can be mapped onto its surface. Stimulation of the superior colliculus drives the eyes toward a point in the visual field that corresponds to the retinal projection to that site. When the image of the target reaches the fovea, a negative feedback system involving the superior colliculus causes the eye movement to end. Examination of saccadic eye movements is best performed at the bedside by instructing the patient to fixate alternatively on two targets, such as the tip of a pen and the examiner’s nose. Saccadic eye movements should be examined in each field of gaze in both the horizontal and vertical planes. The examination should determine whether the saccades are normal, whether they are promptly initiated, and whether they are accurate. Fig. 18.1 Saccades. See Fig. 18.2. The major function of the smooth pursuit system is to maintain the object of regard near the fovea, matching the eye with the target. The stimulus for smooth pursuit is the image of an object moving across the retina, usually within or near the fovea. The image of the object may not cover the fovea (it may be smaller) and may indeed project outside the margin of the fovea, in the parafoveal region. This is preferential under conditions of poor ambient light because rods are more efficient photoreceptors than are cones. In certain circumstances, the stimulus is not a visual image at all. Nonvisual stimuli, such as proprioceptive information related to the movement of one’s own limbs, may be sufficient to generate smooth tracking movements. The latency from stimulus to onset of smooth pursuit movements is 125 msec. The velocity of smooth pursuit movements matches the velocity of the target, which is typically less than 10% of saccadic eye movements (around 30 degrees/s). The feedback substrate is usually continuous and slow; objects that move fast may require a combination of pursuit and saccadic movements. Sac-cadic movements in these instances allow the eye to keep up with the object. The neural substrate for smooth pursuit involves the following sequence: Retinal information on the speed and direction of a moving target is projected on the striate visual cortex of the occipital lobes. This information is relayed to the ipsilateral middle temporal visual area, the frontal eye fields, and the pontine nuclei in a retinotopic fashion. After modification of the visual impulses in the cerebellum and vestibular nuclei, they are finally projected to the ocular motor nuclei. Note that smooth pursuit movements are identified with the ipsilateral occipital lobes, in contrast to saccadic movements, which are identified with the contralateral frontal lobes. To examine smooth pursuits, ask the patient to hold the head still while tracking a small target, such as the tip of a pencil, held at least a meter in front of the patient. Move the target at a slow, uniform speed, and observe whether the eye movements match the velocity and direction of the target. If the pursuit gain is low, then catchup saccades are noted; if the pursuit gain is too high, then backup saccades are noted. When evaluating a patient, it should be kept in mind that execution of smooth pursuits requires that a patient is able to normally attend and that pursuit movements naturally deteriorate with age. Smooth pursuit movements are also particularly sensitive to drugs, which may affect the results of testing. Such drugs include phenytoin, barbiturates, diazepam, chloral hydrate, methadone, alcohol, and marijuana. Fig. 18.2 Smooth pursuits. See Fig. 18.3. The function of vestibular-optokinetic eye movements is to maintain eye position with respect to changes in head and body position. To hold images on the retina, compensatory eye movements must be made to counteract the effects of head movement. To compensate for loss of sensory input, and to counteract the entire range of different head movements, a redundant system of sensory signals contribute to the programming of compensatory eye movements. These sensory signals include inputs from the labyrinthine semicircular canals, the otoliths, vision, and somatosensors that include muscle spindles and joint receptors. Thus, the vestibulo-ocular reflex (VOR), which depends on the semicircular canals, generates compensatory eye movements during high-frequency rotational head movements. The latency of these movements is extremely short, on the order of 10 msec, and the frequency is as high as 300 degrees/sec. Visual inputs form the stimulus for the optokinetic and smooth pursuit systems, which supplement the VOR during sustained (low-frequency) head movements. The otolithic organs, by virtue of their sensitivity to linear acceleration, generate compensatory eye movements during translational movements of the head. The combined inputs from the semicircular canals, the otolithic organs, the visual system, and the somatosensors are relayed together in the vestibular nuclei, where a best estimate of the head’s movement is determined. As part of the clinical examination of vestibular-optokinetic movements, bedside caloric testing is a relatively simple method of determining the side of a peripheral vestibular lesion. If examination verifies the integrity of the tympanic membranes, the patient is positioned supine, with the head flexed 30 degrees. Flexion of the head places the lateral semicircular canals in a vertical position. Irrigate the external auditory canals on each side with a small amount of ice water (a normal response can be elicited with as little as 0.2 mL). Normally, the ear that is irrigated exhibits nystagmus with its quick phase in the opposite direction. Failure to elicit this response indicates loss of unilateral vestibular function. Fig. 18.3 Vestibular-optokinetic eye movements. See Fig. 18.4. Vergence movements align the visual axes to maintain bifoveal fixation so that an object seen by both eyes is perceived as a single object. This requires that the object of interest to fall on corresponding retinal points. In the event that two images of an object fall on noncorre-sponding retinal areas in each eye, one of two possibilities may occur. Either the object is perceived to exist in two separate locations simultaneously, causing diplopia, or the perception is created that two objects are located in the same position in space, causing visual confusion. Normally, when retinal disparity develops, it is so shortlived that it produces neither diplopia nor visual confusion. The latency of vergence movements is ∼160 msec, and the velocity is around 20 degrees/s. The two primary stimuli of vergence movements are retinal disparity and retinal blur. Retinal disparity refers to a disparity between the location of images on the two retinas, and retinal blur refers to defocused images. Whereas retinal disparity is associated with fusional vergence (in which the two retinal images are perceived as one), retinal blur leads to accommodation-linked vergence. This latter type of vergence is associated with pupillary constriction and lens accommodation in response to the retinal blur associated with near vision. Other vergence stimuli include the sense of nearness (proximal vergence), which is related to cues such as perspective and size, and an underlying level of vergence tone (tonic vergence). As mentioned, when the eyes focus on a near object, the response that occurs is the so-called near reflex or accommodation reflex. This reflex consists of (1) convergence of the eyes, (2) accommodation of the lens, and (3) pupillary constriction. As always, the purpose of the vergence component of this reflex is to maintain the image of the object on the fovea of each eye. Accommodation of the lens involves contraction of the ciliary muscle, which reduces the tension on the suspensory ligaments of the lens. This causes the lens to become more spherical to accommodate near vision. Constriction of the pupil helps to produce a sharper image, which also contributes to near accommodation. Fig. 18.4 Vergence eye movements. In the broadest sense, there are two types of eye movements, horizontal and vertical, that provide useful clini-coanatomic correlates. The following section describes the anatomy of these two types of movements and their associated clinical disorders. See Fig. 18.5. Horizontal eye movements originate in either the frontal lobe contralaterally (for saccades) or the ipsilateral occipital lobe (for smooth pursuits). The impulses carrying information about these movements travel through the internal capsule to the paramedian pontine reticular formation (PPRF) in the pons. The left gaze center controls saccades to the left and smooth pursuit to the left. The converse is true for the right gaze center. The tract begins in the frontal lobe gaze center and projects first to the PPRF and then to the ipsilateral sixth nerve nucleus. The projection then reaches the contralateral third nerve nucleus via the medial longitudinal fasciculus (MLF). Fig. 18.5 Anatomy of horizontal eye movements. There are three major types of horizontal eye movement disorders: internuclear ophthalmoplegia (INO), a gaze palsy, and one and a half syndrome. See Fig. 18.6. An INO represents a lesion of the MLF producing failure of adduction on the side of the lesion and nystagmus of the abducting eye. In a posteriorly located INO, the vergence system is intact; in a centrally located INO, the vergence system is impaired. Fig. 18.6 Internuclear ophthalmoplegia. See Fig. 18.7. Gaze palsy is characterized by impairment of the conjugate horizontal gaze to one side or the other. Fig. 18.7 Gaze palsy. See Fig. 18.8. One and a half syndrome consists of (1) impairment of conjugate horizontal gaze to the side ipsilateral to the lesion, and (2) an INO on gaze to the side contralateral to the lesion. This is due to simultaneous involvement of the PPRF (paramedian pontine reticular formation) and ipsilateral MLF by a lesion located in the pons. Thus, as illustrated by Fig. 18.8, a lesion involving the right PPRF and right MLF will result in the impairment of right lateral conjugate gaze (due to interruption of the right PPRF) and an INO on left lateral gaze (due to interruption of the right MLF). Fig. 18.8 One and a half syndrome. Vertical eye movements originate bilaterally and pass to the pretectal area (vertical gaze center) and thence to the third nerve and sixth nerve nuclei. See Fig. 18.9. There are two major types of vertical gaze palsy, Pari-naud’s syndrome and the Steele-Richardson-Olszewski syndrome. Also known as the sylvian aqueduct syndrome, Pari-naud’s syndrome constitutes a problem with the upward gaze and is associated with hydrocephalus secondary to dilation of the sylvian aqueduct. The complete syndrome is characterized by the following problems: (1) vertical gaze paresis, (2) pupillary abnormalities, (3) retraction nystagmus, (4) lid retraction (Collier’s sign) when the patient looks up, (5) loss of convergence, and (6) loss of accommodation. Steele-Richardson-Olszewski’s syndrome (primary su-pranuclear palsy) constitutes a problem with downward gaze and is associated with nuchal rigidity and progressive dementia. Fig. 18.9 Disorders of vertical gaze. The ocular motor nerves comprise the third, fourth, and sixth cranial nerves. See Fig. 18.10. The third nerve nuclear complex is located near the midline at the level of the superior colliculus in the mid-brain. It lies ventral to the cerebral aqueduct and dorsal to the medial longitudinal fasciculus. The Edinger-West-phal nuclei are most rostral. They contain parasympa-thetic neurons that mediate constriction of the pupil. The subnuclei that subserve the levator palpebrae muscle are most caudal. They provide bilateral innervation. All the other subnuclei provide ipsilateral innervation except the superior rectus subnucleus, which innervates the contralateral side. The fascicles of the third nerve exit the midbrain in close association with the red nucleus and the cerebral peduncle. In the subarachnoid space, the third nerve runs between the superior cerebellar artery and the posterior cerebral artery. It also runs in close association with the posterior communicating artery and the medial temporal lobe before piercing the dura just lateral to the posterior clinoid process to enter the lateral wall of the cavernous sinus. Within the cavernous sinus, the third nerve separates into a superior and an inferior division. After traversing the cavernous sinus, both divisions pass through the superior orbital fissure to enter the orbit. The superior division innervates the superior rectus and levator palpebrae supe-rioris muscles. The inferior division innervates the inferior rectus, the inferior oblique, and the medial rectus muscles. The inferior division also contains parasympathetic fibers that mediate pupillary constriction and accommodation. Fig. 18.10 Anatomy of third nerve. See Fig. 18.11. There are three major regions where a third nerve lesion may be produced: (1) nuclear, or within the third nerve nucleus; (2) fascicular, or involving the fibers of the third nerve within the brainstem; and (3) subarachnoid, or involving the third nerve as it passes through the sub-arachnoid space. Nuclear third nerve lesions are rare. Nevertheless, three major patterns of deficits are associated with nuclear third nerve lesions: (1) complete third nerve palsy (including ptosis) on the ipsilateral side, plus ptosis and superior rectus palsy on the opposite side; (2) bilateral pto-sis with normal extraocular movements; and (3) bilateral third nerve palsy with lid sparing. Two brainstem lesions that involve the fascicular third nerve are (1) Benedikt’s syndrome, which comprises an ipsilateral third nerve palsy and a contralateral tremor, usually due to involvement of the red nucleus; and (2) Weber’s syndrome, which comprises an ipsilateral third nerve palsy and a contralateral hemiparesis due to involvement of the ipsilateral cerebral peduncle. Involvement of the third nerve in the subarachnoid space results in symmetric involvement of all divisions of the third nerve, with one exception. The exception is an ischemic lesion. These lesions disproportionately affect the internal portion of the nerve but spare the more peripherally located parasympathetic fibers. The result is a pupil-sparing third nerve palsy. In the cavernous sinus, a third nerve palsy from a compressive lesion is frequently accompanied by other ocular motor nerve lesions and an impairment in the ophthalmic division of the trigeminal nerve. Usually the third nerve palsy is of the pupil-sparing type because compressive lesions in the cavernous sinus preferentially involve only the superior division of the oculomotor nerve, which contains no pupillomotor fibers. Finally, the fronto-or-bital pain that is often associated with a cavernous sinus lesion may be the result of the fact that sensory fibers from the ophthalmic division of the trigeminal nerve join the oculomotor nerve within the lateral wall of the cavernous sinus. Lesions of the third nerve in this location tend to be indistinguishable from lesions in the cavernous sinus, except that the former lesions are more likely to be associated with proptosis. Lesions that involve the third nerve in the orbit are frequently associated with other ocular motor signs as well as optic atrophy and proptosis. Isolated involvement of either the superior or inferior division of the oculomotor nerve is most commonly associated with lesions in the orbit.
Four Types of Eye Movements
Saccades
Smooth Pursuits
Vestibular-Optokinetic Eye Movements
Vergence Eye Movements
Supranuclear Control of Eye Movements
Horizontal Eye Movements
Anatomy
Disorders
Internuclear Ophthalmoplegia
Gaze Palsy
One and a Half Syndrome
Vertical Eye Movements
Anatomy
Disorders
Parinaud’s Syndrome
Steele-Richardson-Olszewski’s Syndrome
Ocular Motor Nerves and Localization of Lesions
Third Nerve
Anatomy
Topographic Localization of Third Nerve Lesions
Nuclear Third Nerve Lesions
Fascicular Third Nerve Lesions
Subarachnoid Third Nerve Lesions
Cavernous Sinus Third Nerve Lesions
Superior Orbital Fissure Third Nerve Lesions
Orbital Third Nerve Lesions
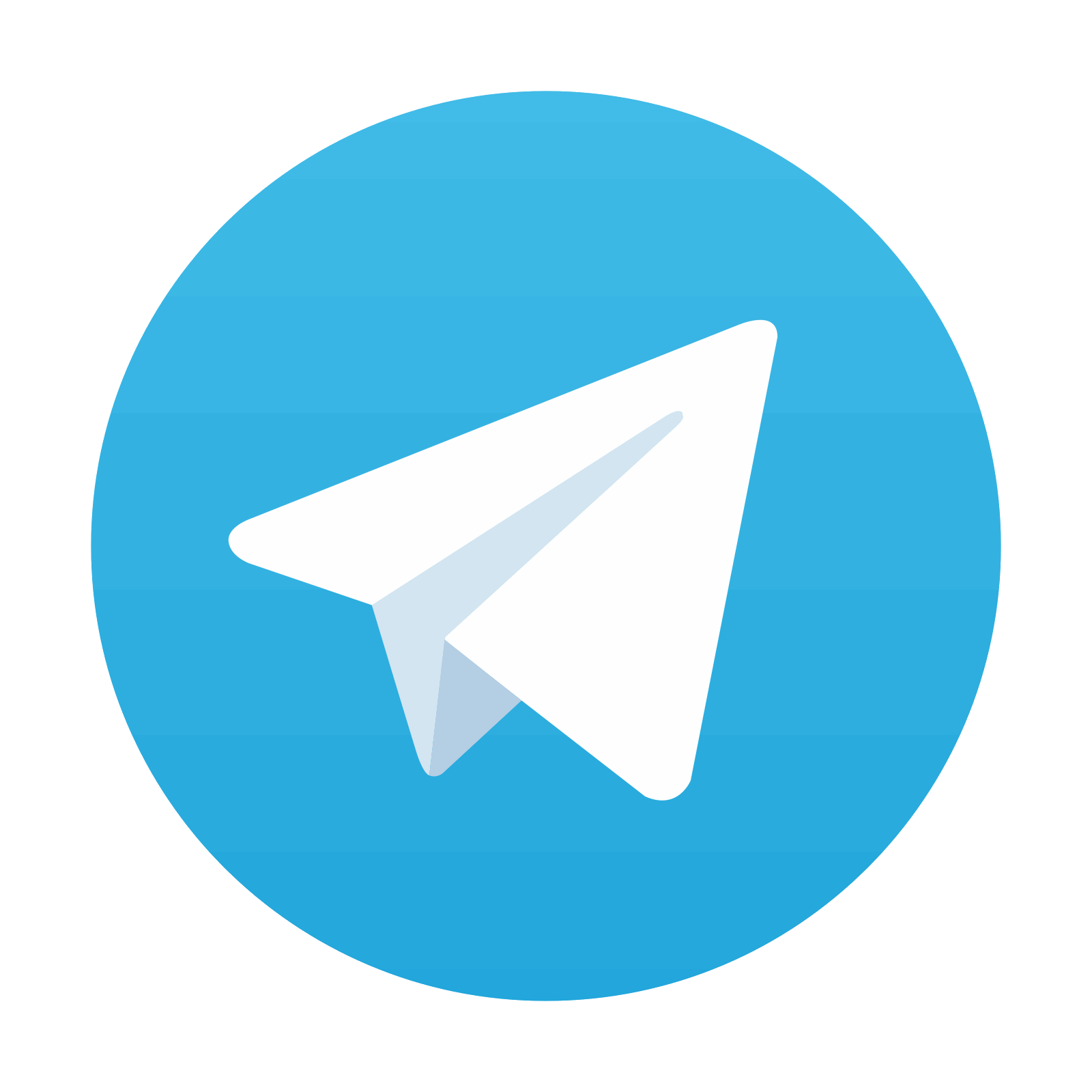
Stay updated, free articles. Join our Telegram channel

Full access? Get Clinical Tree
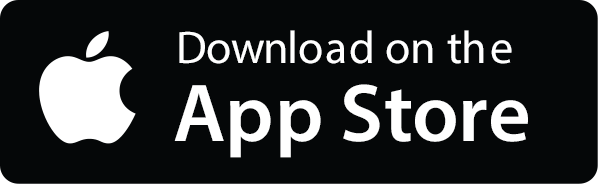
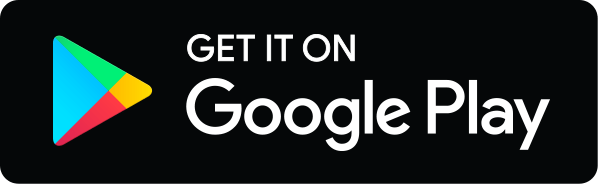