Abstract
Clinical trials in noninvasive brain stimulation (NIBS), such as transcranial electrical stimulation and transcranial magnetic stimulation, have been conducted over the past 15 years to evaluate their potential therapeutic effects on various conditions, such as stroke, depression, or neuropathic pain. Many different parameters can influence the treatment effect of these techniques, influencing the results of a clinical trial. In this chapter, we will review the most important factors that can influence the efficacy of NIBS and how to optimize them by answering the following questions: (1) How to stimulate? Stimulation parameters; (2) Where to stimulate? Brain region targets of interest; (3) When to stimulate? Timing of stimulation; (4) Who to stimulate? Patients selection considerations; and (5) What can be used to optimize stimulation induced neuroplasticity? Combination of therapies.
Keywords
Neuromodulation, Randomized clinical trial (RCT), Transcranial alternating current stimulation (tACS), Transcranial direct current stimulation (tDCS), Transcranial electrical stimulation (tES), Transcranial magnetic stimulation (TMS), Transcranial random noise stimulation (tRNS)
Outline
Introduction 1627
How to Stimulate? Stimulation Parameters 1628
Where to Stimulate? Brain Region Targets of Interest 1629
When to Stimulate? Timing of Stimulation 1630
Patient Selection Considerations 1630
Combinations of Therapies 1631
Summary of Clinical Effects 1631
The Future of NIBS 1633
References 1633
Introduction
Noninvasive brain stimulation (NIBS) has been increasingly investigated in the last decades ( ). Two of the most-studied NIBS techniques include transcranial magnetic stimulation (TMS) and transcranial Electrical Stimulation (tES).
The concept of NIBS involves the use of electrical or magnetic stimulation to either increase or decrease cortical excitability in a specific brain region ( ). TES uses a weak electrical current to induce changes at a subthreshold level, modulating the probability of neural firing when a neuron receives an input from another ( ). The current is delivered through two scalp electrodes, where the current flows from the anode to the reference electrode (cathode) ( ). In the case of TMS, the delivery of magnetic pulses is done through a coil, is able to generate action potentials (APs) in the underlying stimulated cortex ( ). For this specific technique, high-frequency TMS is excitatory, while low-frequency TMS is inhibitory ( ).
In addition to the short-term effects, TMS and tES can induce long-term changes at the synaptic level through mechanisms that resemble long-term potentiation (LTP) and depression (LTD) for anodal and cathodal stimulation respectively. Through LTP and LTD, NIBS can enhance beneficial neuroplasticity and also block maladaptive plasticity in various neuropsychiatric disorders ( ).
tES and TMS are attractive therapeutic options, as they are both noninvasive and affordable. tES, in particular, is also portable and easy to use. In addition to affordability, both techniques have proven to be safe in a number of conditions and populations with very few serious adverse events (AEs) when complying to current safety guidelines ( ).
It is evident that the interest in these techniques have increased over the past decade. Up until now, several stimulation parameters, combination of therapies, and neurophysiological effects have been assessed across multiple studies. Despite this tremendous advance, it still remains an important need to optimize NIBS protocols to potentiate the results. In this chapter, we attempt to clarify and summarize the current evidence for TMS and tES and answer the following questions:
- 1.
How to stimulate? Stimulation parameters
- 2.
Where to stimulate? Brain region targets of interest
- 3.
When to stimulate? Timing of stimulation
- 4.
Who to stimulate? Patients selection considerations
- 5.
What can be used to optimize stimulation induced neuroplasticity? Combination of therapies
How to Stimulate? Stimulation Parameters
The most common and first-studied tES technique is transcranial direct current stimulation (tDCS) ( ). Current protocols use defined stimulation parameters according to the safety guidelines ( ). The most frequently used parameters include an electrode size of 25–35 cm 2 , a current of 1–2 mA for up to 20–40 min, for 1 up to 20 sessions; these parameters have shown to be safe and effective across multiple studies in both healthy subjects and patients with different neurological conditions ( ). However, severe AEs in the skin have been reported, which has led to the assessment of the stimulation intensity, electrode–skin interface, electrochemical processes, and electrode design and placement ( ). The issue is that, when applying tDCS, the current distribution at the electrode–skin interface is not uniform ( ). In two studies, eight cases of skin injuries were reported with the use of repeated tDCS, with three occurring at the anode site ( ) and five at the site of the cathode ( ). The findings were associated with the use of tap water and high impedance levels after multiple repeated and prolonged sessions of tDCS. To avoid these AEs, tDCS should be applied with nonmetallic rubber electrodes covered completely by a saline-soaked sponge. Although the current methods of tDCS application seem adequate, it is essential to follow the safety guidelines and good clinical practices ( ). Clinically, tDCS has shown to improve cognitive functions (such as memory, attention, or language processing) as well as motor functions (such as strength or dexterity) in both healthy individuals and patients with neurological conditions ( ). It has also been tested in chronic pain, depression, and other psychiatric disorders with positive results ( ; ).
Another technique is transcranial alternating current stimulation (tACS). It is used to modulate ongoing brain oscillations, and its importance relies on the association of different cognitive states to specific brainwave frequencies ( ). In this particular technique, the sinusoidal wave pattern changes cyclically over time; in the first half, one electrode will serve as the anode and the other as the cathode, and on the other half of the oscillation, the electrodes roles are reversed ( ). This means that the membrane potential is likely not affected. Although the full mechanisms are not fully understood, it has been proposed that tACS works by (1) entraining the underlying cortical oscillations through the induction of a specific electroencephalography (EEG) frequency band and (2) inducing synaptic plasticity through LTP and LDP mechanisms ( ). It is well known that brain oscillations represent various cognitive functions ( ), and so, the enhancement of these oscillations through an exogenous input have the potential to modulate cognitive and sensory-motor processes ( ). This is why the modulation of tACS parameters such as the amplitude, frequency, and phase of the EEG amplitude is quite different from other NIBS techniques. tACS delivers a weak current (≤2 mA) in an alternating pattern between two or more scalp electrodes (usually 16 cm 2 ) that are placed in similar positions to tDCS. Several frequencies can be used including 1, 10, 15, 30, and 45 Hz; with a voltage of 5–15 mV ( ). In the case of amplitude and frequency, ongoing brain oscillations of the patient/subject need to be well assessed to then be “synchronized” with the desired oscillation in the target area of the patient’s brain.
Multiple studies have shown associations between particular diseases and brainwaves as a result of pathological processes. For example, patients with chronic pain conditions frequently have a reduced alpha rhythm and increased theta power ( ). A study with tACS showed that when the current was applied in the posterior area of the brain at a frequency of 10 Hz, there was an increase in alpha waves amplitude ( ). This is evidence of how it is possible to modulate maladaptive plasticity seen in some disorders, through the synchronization of the brain, according to a specific EEG frequency. As for the phase of the EEG amplitude, it gives information about the timing of the underlying neuronal activity ( ). Multiple studies have shown how the EEG phase can be locked to the transcranial alternating current ( ). A study showed that 10 Hz applied to the auditory cortex showed that the phase of alpha activity predicted whether a near-threshold auditory stimuli was perceived ( ). Regarding the safety of tACS, studies have reported minor side effects. The most common side effect has been retinal phosphenes ( ) in a frequency and intensity dependent manner ( ). Clinically, tACS has shown to improve attention ( ) and working memory ( ) but also to induce lucid dreaming when applied during sleep ( ).
A similar technique to tACS is transcranial pulsed current stimulation (tPCS). The main difference when compared to tACS is the electrode location. The current is delivered through two electrodes that are placed in extracephalic positions including earclip and bimastoid montages. Computational studies as well as other studies ( ) have shown how electrode positioning determines which brain structures are reached and what are the underlying electrophysiological processes, based on the montage. Unlike tACS, the best results with tPCS were found with random frequencies between 6 and 10 Hz ( ). The possible underlying mechanism is the stochastic resonance phenomena, where the addition of a random noise is able to amplify the weak signal on a system because the sum of two signals exceeds the threshold at certain times, depending on what is needed for a particular task ( ). By increasing synchronization of neural firing, it can improve the signal-to-noise ratio to enhance cognitive performance or perception. However, more research on this technique is needed. Preliminary results on tPCS showed that the bimastoid montage has similar effects to tACS in terms of alpha power spectrum, and thus, on the already known corticocortical and corticothalamic connectivity ( ). In summary, the optimization of tPCS depends on several factors that provide evidence of how simple variations in the parameters of stimulation lead to significant clinical benefits.
Finally, transcranial random noise stimulation (tRNS) is another type of tACS where the frequency and intensity of the delivered current change randomly ( ); typically, between −500 and +500 μA, 0.1–640 Hz respectively. Interestingly and different to tACS and tPCS, studies have shown that it enhances cortical excitability and may alter glutamate mediated synapses ( ). The duration has also shown to play a crucial role in the tRNS effects. As an example, the application of tRNS with a noise containing frequencies from 101 to 640 Hz, with a maximum density of 62.5 μA/cm 2 over the left motor cortex showed significant increased facilitation of motor cortical excitability with 5 and 6 min rather than 4 min ( ).
Similarly to tES, repetitive transcranial magnetic stimulation (rTMS) has shown to be a safe technique if used under the safety criteria ( ). The common parameters involve magnetic pulses delivered in stimulation trains of 1–5 s in duration with frequencies that usually ranges from 1 to 20 Hz. Lower frequencies inhibit cortical excitability, whereas higher frequencies increase it.
In addition to parameters related to clinical effectiveness, safety factors also need to be considered. Spacing pulses and excluding metals that are highly conductive is essential as prolonged heating can cause skin burns. It is also not recommended to test subjects that have metallic implants in the head, as they may overheat with a TMS-induced currents ( ). Besides temperature considerations, it is important to be cognizant of some of the common TMS side effects. These include acute and transient changes in mood, cognitive performance, headaches, or pain/discomfort ( ). Also, one of the most fearful side effects of TMS is seizures. Although theoretical evidence states there can always be a risk of seizures due to the direct effect on APs, very few cases have been actually been reported with the use of standardized guidelines ( ). Overall, TMS sessions have shown to be well tolerated among a wide variety of subjects.
Where to Stimulate? Brain Region Targets of Interest
Early NIBS trials have investigated the effects of TMS and tDCS on main brain regions, such as prefrontal cortex to improve cognition or motor cortex to improve motor function, based on their underlying functions. However, the way the current is distributed in a healthy subject and in a patient with a brain lesion is not comparable, and therefore, changes the effect and underlying function. Thanks to head modeling, it is now possible to anticipate the actual current field based on the patients’ MRI. Depending on the individualized patients’ brain lesion, personalized head and current field modeling could help to determine which electrode montage would be most appropriately to target the desire brain area. Besides the patient’s brain lesion, it may also be promising to select specific brain targets based on the exploration of functional connectivity, assessed by neuroimaging techniques to target a specific brain network ( ). Also, we know that tDCS can affect not only the target brain region but also the entire network related to the stimulated area ( ). In this scenario, a specific NIBS montage could be developed that targets a particular disrupted brain network, using, for example, a multichannel tDCS. An additional fact is the depth of the electrical field and the neurophysiological changes produced at various brain levels. This may potentiate the clinical efficacy of the NIBS, considering that deep brain structures, such as the thalamus or the basal ganglia, are known to be involved in the pathophysiology of several neurological conditions.
As an example, H-coil deep TMS (dTMS) is a technique that allows stimulation of extensive neural pathways including cortical and subcortical regions. It has already been approved for the treatment of resistant, major depressive disorder, and has promising results for other psychiatric conditions such as obsessive-compulsive disorder ( ). Due to the report of seizure events in some adolescents receiving dTMS ( ), researchers have to carefully assess the risk-benefit ratio until further stimulation parameters are tested that improve its safety.
When to Stimulate? Timing of Stimulation
In this section, we discuss the most appropriate timing to stimulate a subject, as the level of ongoing brain activity has an important impact on the success of TMS and tDCS. Conventional NIBS protocols do not usually take it into account. This has led to mixed results, as effects may vary depending on the ongoing level of brain activity. Indeed, it is now clear that the effects of NIBS are state-dependent ( ). For instance, an index that can differentiate a conscious from an unconscious state has been elaborated. It is called perturbational complexity index (PCI) and is based on the brain reaction to a TMS stimulus ( ). Because TMS’s ability to modulate cortical activity is dependent on the level of arousal, its capacity to change cortical activity relies on the subjects’ state and ongoing tasks during the stimulation session.
The first step would be to determine when is the best observed response, based on the subjects’ cortical activity, behavior, or mental state and then trigger the stimulation once a predefined threshold has been reached. This is called a closed-loop system. More and more studies investigate the combination of NIBS and cortical outputs, such as electroencephalogram markers. For instance, EEG beta rhythm, generated by the imagination of foot movements has been already used to induce grasping movements for functional electrical stimulation (FES) ( ). The combination of NIBS and EEG is feasible. The most difficult part is determining the best biological marker and individual threshold that would trigger the stimulation. The closed-loop system involves the integration of (1) specific neural patterns found in EEG for behavior/cognition and (2) corresponding NIBS-polarity induced effects. Using this information, a closed-loop system would allow a more effective modulation of cognitive performances, based on the ongoing brain activity ( ). In addition to this cognitive enhancement, there is growing evidence of the effects of tDCS on motor learning ( ). In this context, if tDCS is integrated in a closed-loop system, which promotes motor learning, the therapeutic effects of this system could be substantially enhanced. In fact, such closed-loop systems employing TMS has been suggested as a potential tool to improve poststroke motor recovery.
From a more simplistic perspective, the effect of NIBS can be modulated positively or negatively, based on the subject’s state, and therefore, it is something that one needs to be cognizant of and reported when conducting a study.
Patient Selection Considerations
Responsiveness to NIBS therapy varies considerably with the involved neural network and the corresponding disorder. Several examples can be seen in the literature: (1) 40% of patients with tinnitus respond to tDCS with a mean reduction of 13.5% in the intensity ( ) (2) 60% of patients with multiple sclerosis related fatigue exhibit significant reduction in the fatigue impact scale after an anodal tDCS ( ) and (3) as high as 80% for patients with human T-lymphotropic virus (HTLV-1)-induced chronic pain ( ). Furthermore, a study assessing the effect of a single tDCS session on severely brain injured patients in minimal consciousness state, demonstrated that 43% had significant clinical improvement following tDCS ( ). A closer look to the former patients’ MRI and PET scans revealed that those who responded to tDCS had a partial preservation of metabolic activity as well as grey matter in the underlying area of stimulation (i.e., left prefrontal cortex). In addition, there was preservation of the thalamus and precuneus, two critical regions for the recovery of consciousness ( ). This study highlights the importance of neuroimaging as a tool to predict what patients are the best candidates for NIBS, based on the brain function and anatomical integrity.
Interestingly, a study in depression that used repeated sleep deprivation as an antidepressive therapy, identified high cortical excitability at baseline (as measured by the global mean field power) among responders when compared to nonresponders. In addition, the level of cortical excitability of each individual during and after tDCS was correlated to the clinical response. This TMS/ EEG combination led to the elucidation of how the cortex reacts (EEG) to an external stimuli (TMS) and how this could be used as a tool to adequately predict the response to NIBS treatment.
Although this is an important area of research, there are still many unknowns on how to identify best responders. It is not clear whether neurophysiological or clinical parameters may be more useful or even a combination of them. Future large studies using multivariate regression analytic methods can help to answer this question.
Combinations of Therapies
Studies have shown that the combination of NIBS with a behavioral therapy (e.g., physical or occupational therapies) induce better results than either the therapy or NIBS alone. The concomitant use of these therapies could enhance a functional focalization of the NIBS effects as a specific network is reached through two different pathways.
Due to its neuromodulatory effects, tDCS has often been used in conjunction with a specific therapy, either before (offline) or during (online), to improve motor and cognitive functions in both healthy subjects and patients with neurological conditions (for reviews, see ). In patients with stroke, several trials have investigated the efficacy of rehabilitation treatment combined with NIBS. One study identified that the effects of occupational therapy on hand dexterity, which is a common therapy in stroke rehabilitation programs, were significantly higher when combined with tDCS ( ). Another study using more complex technology, upper extremity robot-assisted training, has shown to induce superior effects on motor function in patients with chronic subcortical stroke when compared to each technique alone ( ). tDCS has been used in combination with virtual reality in subacute stroke patients to increase their neurorehabilitation (e.g., improve motor function, Barthel Index, and increase in corticospinal excitability; ).
TMS has also been tested in combination with rehabilitation programs. For instance, in multiple sclerosis, a study showed that the effect of combining rTMS, intermittent theta burst stimulation, with physical therapy for spasticity was superior to TMS alone ( ). Furthermore, the effects on perceived fatigue, daily living activity, and quality of life improved when exercise therapy was combined with TMS ( ). The development of NIBS protocols must include an initial assessment of the efficacy and safety parameters for each of the NIBS techniques followed by protocols in which additional interventions (e.g., behavioral therapy) are concomitantly used and assess their effect on rehabilitation.
Pharmaceutical agents have also been a target of NIBS clinical trials. In the same way as behavioral therapies, their effects may enhance NIBS techniques and thus, clinical benefit. As an example, explored the combination of tDCS with Sertraline versus each intervention alone for the treatment of depression. In this clinical trial the authors evidenced that tDCS/Sertraline combination increases the efficacy of each treatment used individually. Due to the extensive pharmaceutical possibilities that can be added to a NIBS protocol, it is necessary to understand the drug’s profile and mechanism of action since they could either enhance or interfere with the NIBS effects. For example, it is theorized that calcium and sodium channel blockers decrease the cortical excitability created by the anodal tDCS, whereas cathodal stimulation effects (reduction in excitability) remain intact ( ). Other drugs such as citalopram (or sertraline in study), which is a 5-HT reuptake-inhibitors, could facilitate plasticity and enhance the effects of anodal tDCS stimulation ( ).
Finally, it is also important to note that choosing which techniques to use is a determining factor in success or failure. In fact, if two techniques are targeting competing networks, combination therapies have the potential to decrease the effects of both types of stimulation. For example, one study combining tDCS and tPCS using concomitant stimulation, had either decreased or no effect on the quantitative electroencephalography power, while each technique used alone increased the power of cortical oscillations ( ).
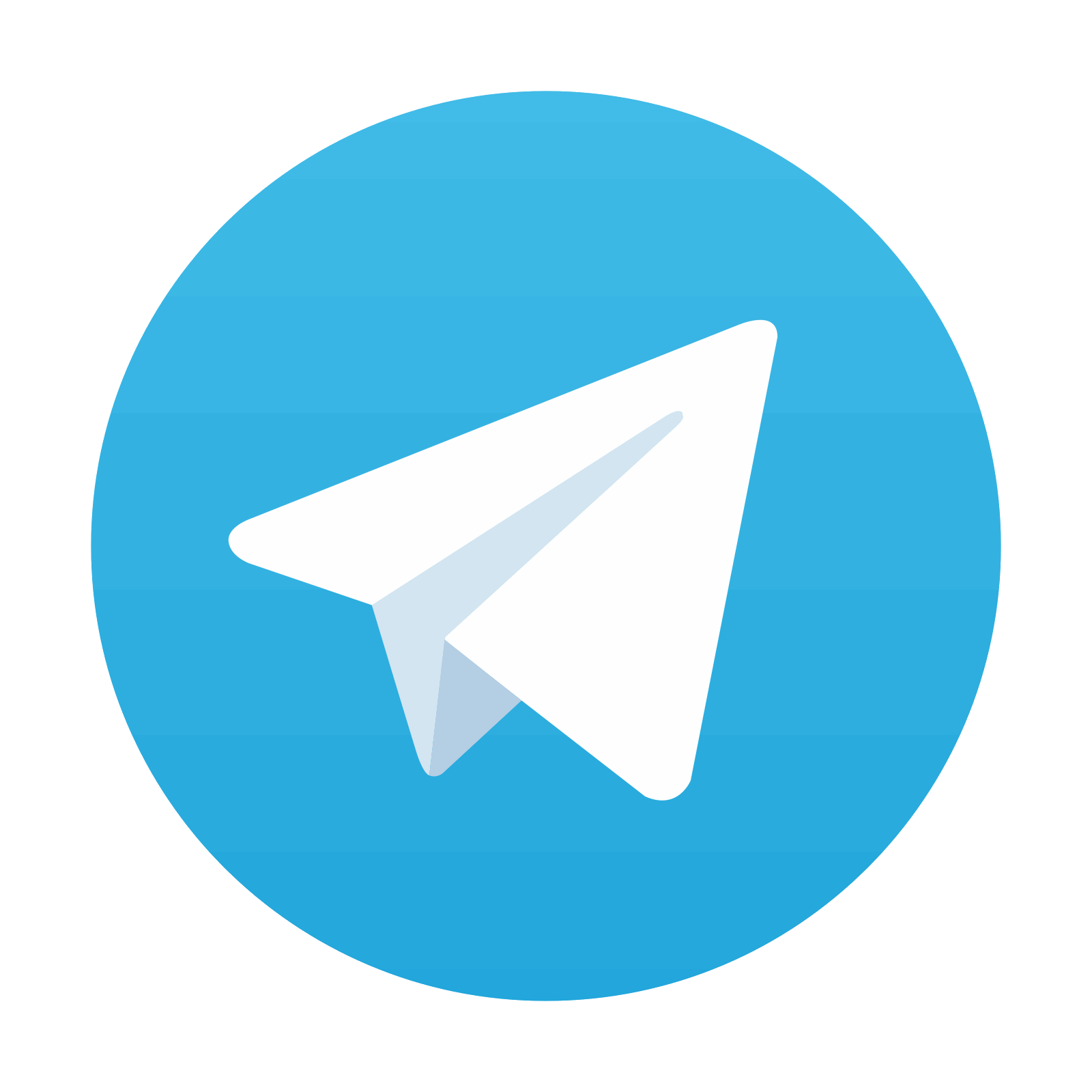
Stay updated, free articles. Join our Telegram channel

Full access? Get Clinical Tree
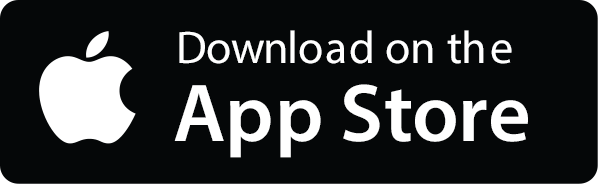
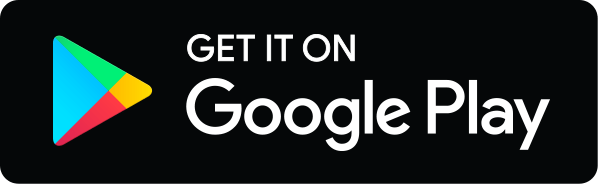