5 Penetrating Cerebral Trauma Arnett Klugh III and Griffith R. Harsh IV Despite the advancements of sophisticated imaging modalities, microsurgical technique, neuroanesthesia, and specialized intensive care medicine, the treatment of patients with penetrating craniocerebral trauma remains a formidable task for the neurosurgeon. Each year 1.4 million people in the United States suffer a traumatic brain injury (TBI).1 Missile injuries to the head are the most common cause of penetrating cerebral trauma. Since 1990, the leading cause of TBI-related death in the United States has been penetrating craniocerebral trauma from firearms.2 The U.S. citizenry owns more than 250 million firearms.3 Although, by estimates from the Centers for Disease Control (CDC), gunshot wounds to the head represent only 10% of all TBI in the United States, these injuries account for 44% of all TBI-related deaths. Most cases of firearm-related penetrating cerebral trauma involve suicidal intent, but greater than 25% are the result of assaults by others. Other less frequent causes of penetrating cerebral trauma are of low velocity: knives, screwdrivers, ice picks, axes, arrows, and pneumatic nail guns. The alarming increase in civilian penetrating cerebral trauma associated with gunshot wounds has occurred in both rural and urban environments. In rural communities, suicides outnumber homicides, and shotgun and rifle injuries are more common than those caused by handguns and automatic weapons.4 The incidence of firearm-related penetrating cerebral trauma is highest among urban African American males. Such injuries are often devastating to the patient, family, and society. The prevalence and the variability of penetrating brain injury demand that neurosurgeons understand the fundamentals of wound ballistics and the associated mechanisms of cerebral injury. Missile injuries to tissue reflect the kinetic energy imparted by the projectile, the shape of the projectile, the approach angle of the projectile, and the impedance of intervening tissues (bone, muscle, mucosa, brain). Kinetic energy is described by the equation KE = 1/2 mv2. Although the mass of the projectile is important, the squared velocity term predominates. Projectiles causing penetrating cerebral trauma are thus classified into low-velocity (<1000 ft/s) and high-velocity categories (>1000 ft/s).5 Low velocity sharp projectiles travel 120 to 250 ft/s (36 to 76 m/s) and include objects such as knives, screwdrivers, or arrows.6 These projectiles cause focal injury along their trajectories consistent with their shapes and do not convey significant energy to surrounding tissues. In contrast, military weapons, such as the U.S. Military M16A1 rifle, expel projectiles at velocities in excess of 3000 ft/s. Civilian handguns produce bullet velocities of 710 to 1610 ft/s (216 to 491 m/s) and civilian rifles produce bullet velocities that range from 2690 to 3150 ft/s (820 to 960 m/s). These higher velocity weapons create extensive damage in tissues surrounding the trajectory of the bullet by a process termed cavitation. The biomechanical effects of higher velocity projectile penetration have been studied using ordnance gelatin as a tissue simulant.7 Initially, an impact shock wave precedes the strike of the projectile. This wave is brief and biologically benign. Penetration of the skull by the projectile causes direct and permanent crush injury of brain tissue along its trajectory (Fig. 5-1). In addition, kinetic energy is directed tangentially from the projectile. This creates a cavity surrounded by compressed brain tissue. This cavitation, created by pressure waves as kinetic energy is released, is temporary. After the positive pressure wave passes, the pressure gradient across the wall of the cavity is reversed. The negative intracavitary pressure both causes its walls to collapse inward and sucks contaminated external debris into the wound. The cavity then undergoes attenuating repetitive expansions and contractions. Because the brain parenchyma has relatively high density and low elasticity, it is highly susceptible to this cavitation; the width of injury tracts can be 10 to 20 times the diameter of the projectile. Cavitation injury has the potential to produce elevations in intracranial pressure (ICP) ranging from 60 to 100 mm Hg.8 The magnitude of tissue damage also depends on other factors. Missiles may ricochet within the cranium to create additional tracks of brain traverse and injury. Their collisions with the inner table of the skull may also fracture off fragments of skull or projectile that become secondary missiles that magnify injury (Fig. 5-2). Although its velocity strongly influences the projectile’s energy, the manner in which its energy is transferred to brain tissue is also very important. This depends on such factors as missile deformation, yaw, and fragmentation. Mushroom deformation and irregular fragmentation of projectiles upon contact increase the spatial distribution of energy transfer and create multiple injury tracks, causing greater tissue destruction. Dumdum and hollow-point bullets are examples of rounds engineered to enhance deformation and fragmentation upon striking tissue. The Devastator bullet combines a hollow tip with an explosive packed within the tip designed to detonate on impact. The significant failure rate of this explosive ammunition represents a serious risk to health care professionals.9 Removal of these rounds requires careful surgical planning, since manipulation, ultrasound imaging, and exposure to microwaves may cause detonation. Yaw is the rotation of a projectile around its long axis. When projectiles contact tissue, they frequently tumble with high yaw. This promotes higher energy translation tangentially, which creates larger cavities and more complex wounds, explaining why exit wounds are frequently larger than entrance wounds.10 Figure 5-1 (A) CT scan of a gunshot wound victim shows the right-to-left path of the bullet, with intracranial bone fragments and air. (B) Soft-tissue window shows parenchymal blood and edema along the path of the bullet, as well as interhemispheric and intraventricular blood and the air and bone fragments. There was a large amount of intraventricular blood, mass effect, and herniation at the time of the scan, and the patient expired soon after. Figure 5-2 (A) Bone window from a CT of a gunshot wound victim shows comminuted right frontal fractures, pneumocephalus, and both bone and bullet fragments. The metal is distinguished from bone fragments by higher density and associated artifact. (B) Soft-tissue window reveals a broad area of hemorrhage and edema in the right frontal lobe, as well as interhemispheric blood. The right frontal lobe was debrided, and the patient survived initial hospitalization to rehabilitation. Figure 5-3 Pseudoaneurysm from gunshot wound. After intravenous contrast administration, an intensely enhancing structure becomes visible; this was a pseudoaneurysm of the callosal marginal artery, confirmed at angiography and surgery. There is a thin rim of enhancement around the hematoma. Injury from penetrating cerebral trauma is characterized by a primary insult created by the ballistic properties of the projectile, followed by a cascade of secondary tissue and cellular alterations in response to the primary insult. Cavitation creates a mechanical load on the cerebral vasculature that may result in partial or complete vessel transection. Direct laceration of vessels by the projectile is also possible. The incidence of subarachnoid hemorrhage (SAH) is as high as 78% on the basis of computed tomography (CT) scan data.11 Parenchymal contusion and intracerebral and intraventricular hematomas are also common. A review of the literature reveals that the incidence of traumatic intracranial aneurysms caused by penetrating cerebral trauma is uncertain. However, 20% of all traumatic aneurysms after TBI (Fig. 5-3) are caused by penetrating cerebral trauma, and early recognition and treatment significantly reduce the incidence of aneurysmal rupture and its accompanying morbidity and mortality.6 A projectile trajectory that spans the Sylvian fissure or travels near the supraclinoid carotid artery, cavernous sinus, or a major venous sinus, or the development of delayed, unexplained SAH should prompt concern for a vascular injury and cerebral angiography should be obtained. Secondary tissue and cellular responses to missile injury cause brain swelling. This reflects both vasogenic and cytotoxic edema.12 In animal models, edema appears almost exclusively adjacent to the wound track and has been identified by electrolyte composition as predominately vasogenic.13 Astrocytic swelling also contributes as the disruption of normal cell integrity induces a cascade of cell injury involving release of glutamate and excitotoxicity, creation of free oxygen radicals, and further cellular injury, with influx of sodium, release of calcium, and cellular expansion. All these factors combine with tissue hypoxia and ischemia to raise ICP. In animal models of penetrating cerebral trauma, reproducible cardiopulmonary responses have been identified. Mean arterial pressure (MAP) increases within seconds of the injury, gradually returning to baseline within the first few minutes after injury. Intracranial pressure shows an initial dramatic spike, reflecting the pressure wave associated with cavitation, followed by a gradual decrease over several minutes to a reset level elevated above baseline. In severe injuries, ICP then increases over several hours in proportion to the energy delivered by the projectile. The cerebral perfusion pressure (CPP = MAP – ICP) also follows a predictable pattern. Immediately after wounding occurs, an increase in MAP is the predominant influence; CPP increases accordingly. Several minutes after the initial insult, an elevation in ICP predominates, the MAP falls, and CPP decreases, creating cerebral hypoxia and ischemia. Apnea frequently occurs after gunshot wounds to the head and its duration correlates with wounding energy. These cardiopulmonary changes are postulated to be caused by a pressure differential created across the foramen magnum that distorts the brainstem and displaces it caudally. Support for this hypothesis is found in changes observed in adrenergic and serotonergic nuclei of the brainstem in animal models of missile brain injury.14 Systemic changes in coagulation frequently accompany craniocerebral missile injuries. The extrinsic coagulation pathway is activated by the release of thromboplastin from the injured brain. Abnormalities of blood coagulation in brain injury predict a poor outcome and may be exacerbated by the excessive catecholamine release associated with severe brain injury.15 Modern treatment of penetrating cerebral trauma is based on knowledge obtained from military conflicts. Cushing’s advocacy of en bloc craniotomy, with meticulous debridement of devitalized tissue, bone, brain, and foreign debris, and watertight closure of the scalp, reduced postoperative mortality to 28%, even without the benefit of antibiotics.16 Evaluation of neurosurgical efforts in the Vietnam War and the availability of CT imaging eventually led to a less aggressive approach to penetrating cerebral gunshot wounds. Carey et al revealed that repeated surgery to aggressively remove retained fragments resulted in significant morbidity and mortality.17 In addition, studies from Vietnam showed that, despite aggressive debridement, 23% of cases had retained fragments by CT follow-up evaluation. Unlike that in civilian injuries, most penetrating cerebral trauma in military conflicts is the result of shrapnel, not bullets. In addition, because the high missile velocity of military weapons often results in either immediate death or triage to nonoperative care, the military population treated has a disproportionate number of low-velocity shrapnel injuries. The military literature provides Class III evidence that aggressive debridement is not necessary to either prevent infection or prevent epilepsy and is associated with higher morbidity and mortality.6 Military wounds are frequently contaminated from battlefield conditions. Multivariate analysis of the 1964 Iran-Iraq War experience revealed cerebrospinal fluid (CSF) leakage, transventricular injury, and disruption of air sinuses as independent predictors of infectious complications of penetrating cerebral trauma.18 In particular, the Vietnam Head Injury Study (VHIS) demonstrated a mortality of 22.8% for patients with CSF leakage, versus 5.1% for patients without leakage.19 In the study, only half of the CSF fistulae were found at the wound site. These findings urge vigilant and effective treatment of CSF fistulae to minimize potentially lethal secondary complications. Despite in-field resuscitation and rapid access to a full range of medical resources, the mortality for penetrating cerebral trauma remains high in the civilian realm, approaching 94% by CDC estimates. In contrast to the military literature, the civilian literature reflects a bias to limited debridement even during the pre-CT imaging era. Emphasis is placed on evacuation of space-occupying lesions, mass effect causing increased ICP in patients with potential for significant neurological recovery. Initial evaluation of a patient with penetrating cerebral trauma should follow resuscitation in accord with the Advanced Trauma Life Support guidelines. Endotracheal intubation is indicated for impaired ventilation, inability to protect an airway, and potential for neurological deterioration. Patients with a Glasgow Coma Scale (GCS) score of 8 or less meet these criteria. During intubation, ICP should be treated as though it were increased. Maintenance of a systolic blood pressure of at least 90 mm Hg is recommended. Intravenous access is obtained and resuscitation is begun with isotonic normal saline (0.9%). Cervical spine injury concurrent with penetrating cerebral trauma is rare. A primary survey of the neck, chest, abdomen, pelvis, and extremities is completed. Priority is given to treatment of life-threatening airway, pulmonary, or circulatory compromise. Caution is used in placing endotracheal and gastric tubes to avoid inadvertent passage into the cranial cavity. A brief history, including the patient’s age, events surrounding the injury, and the GCS at the scene, upon arrival in the emergency room and after resuscitation, is obtained. In addition, any evidence of hypotension or hypoxia at the scene or during transfer should be noted. A focused, rapid neurological examination, assessing level of consciousness, pupillary size, symmetry and reaction, brainstem reflexes, motor response, and respiration, should be performed. This information provides initial prognostic data. Intentional injuries are associated with higher mortality, as are low GCS, and even brief episodes of hypotension and hypoxia.20 A CT scan of the head is obtained when the patient is hemodynamically stable. Shaving the scalp facilitates detection of small wounds. Large scalp defects should be assessed for wound contamination, preservation of vascular supply, presence of associated fractures, CSF egress, or brain herniation. Significant scalp hemorrhage can be controlled temporarily with Leroy-Rainey clips until definitive operative treatment is performed. In the case of gunshot wounds, the patient requires careful examination to identify entrance and exit wounds. Lower velocity penetrating objects, like knives or arrows, should be left in place until full assessment, including radiological studies, is performed and the object can be removed in the operating room under surgical control. Computed tomography scanning of the head is the study of choice for evaluating penetrating cerebral trauma. Clinical management requires localization of the projectile, fragments, bony destruction, and in-driven debris, and identification of any hematoma, contusion, mass effect, IVH or SAH. In addition, CT provides prognostic information; traverse of both hemispheres, multiple lobes, the diencephalon, or the brainstem is associated with increased mortality. Cerebral angiography should be performed in cases of suspected vascular injury. A wound trajectory through major blood vessels, delayed development of intracranial hematoma, or unexplained SAH should also prompt angiography.20 Magnetic resonance imaging (MRI) is contraindicated in patients with penetrating cerebral trauma involving metal fragments because of the artifact created by the metal and the risk of additional injury caused by migration of the fragment in response to the magnetic field. If there is no intracranial metal, MRI may be helpful in selected cases for evaluation of complications of penetrating cerebral trauma (Fig. 5-4). No strict criteria for surgical treatment of penetrating cerebral trauma exist. Grahm et al developed a set of prospective guidelines for the management of civilian craniocerebral gunshot wounds, emphasizing the initial GCS and CT imaging.21 In 100 consecutive cases, no patient with a postresuscitation GCS of 3 to 5 achieved a satisfactory outcome. The study confirmed the importance of the initial GCS to patient outcome, and the association of bihemispheric, transventricular injury with a poor prognosis. Grahm et al recommended that patients with a GCS above 7 should receive surgical treatment and that those with GCS scores of 5 to 7 should be triaged to nonoperative management if their injury was multilobar, transventricular, or in the dominant hemisphere. This study is confounded by the large number of patients who were transported without intubation for ventilatory support and the lack of standardization of management of postoperative ICP. The decision to pursue aggressive therapy is an amalgamation of history, neurological examination, radiographic evaluation, the patient’s legally expressed desires or the family’s interpretation of the patient’s wishes, and the judgment and experience of the neurosurgeon. A major reason to operate is the evacuation of a hematoma, with mass effect, in the temporal region or the posterior fossa. Other reasons for surgical intervention include an orbitofacial wound, the need to control active hemorrhage, and evolution of an expansive intracranial lesion in a patient who is neither decerebrate nor flaccid. The goals of surgery for penetrating cerebral trauma are evacuation of hemorrhage and wound debridement (Fig. 5-5). Small entrance wounds may be treated with local wound care if the scalp is not devitalized and no intracranial pathology requiring surgical intervention for reduction of mass effect is identified on CT imaging. Wounds that have devitalized scalp, bone, and dura require operative intervention to debride devitalized tissue and close the dura to prevent leakage of CSF. Preoperative planning should allow for wide surgical exposure. The patient’s head is positioned to provide access to all entry and exit wounds. Placing the patient supine with the head on a horseshoe headrest will facilitate access to most bilateral wounds. The choice between craniotomy and craniectomy depends on the degree of fragmentation of the skull. The incision is planned to avoid compromising the scalp’s blood supply and to incorporate the scalp wound if possible. After removal of the bone flap, intact dura is exposed on all sides of the penetration. The subdural space is inspected and any hematoma is evacuated. Necrotic brain tissue and foreign debris that are readily accessible are removed, using a combination of gentle suction and irrigation. Absolute hemostasis is obtained. Watertight dural closure, particularly of wounds that involve the air sinuses, with pericranium, temporal or occipital fascia or fascia lata, is essential to preventing postoperative CSF leakage and infection. Common postoperative complications include recurrent hemorrhage, intracranial hypertension, seizures, infection, and CSF fistulae. Close observation in an intensive care environment is necessary for early recognition and intervention. Victims of penetrating cerebral trauma are frequently plagued with respiratory problems; many suffer aspiration pneumonia. In addition to ICP monitoring, cardiac monitoring is necessary, as the catecholamine release associated with severe head injury may cause myocardial ischemia. Maintenance of CPP greater than 70 mm Hg may require invasive cardiac monitoring. Associated hypothalamicpituitary dysfunction may manifest as diabetes insipidus or SIADH. Nutritional supplementation and prophylaxis of gastric injury with H2 antagonists should be given. Indications for anticonvulsant therapy after penetrating cerebral trauma remain controversial. The Class I evidence for its use in preventing early seizures includes only a small number of patients with penetrating cerebral trauma.22 Based on this, 7 days of prophylactic therapy after injury are recommended. Penetrating cerebral wounds are frequently contaminated by skin, clothing, hair, and external debris sucked into the brain during cavitation. Prophylactic broad spectrum antibiotics are recommended, as culture studies of military penetrating head wounds have revealed Staphylococcus, Acinetobacter, Streptococcus, E. coli, Klebsiella, and Enterobacter in wound, brain, and bone.18,23 The reduction in infection rates following use of antibiotics in World War II is the most compelling evidence to date.
Ballistics
Mechanisms of Injury
Management of Penetrating Cerebral Trauma
Resuscitation and Initial Management
Imaging for Evaluating Penetrating Cerebral Trauma
Surgical Management of Penetrating Cerebral Trauma
Complications and Adjuvant Therapy
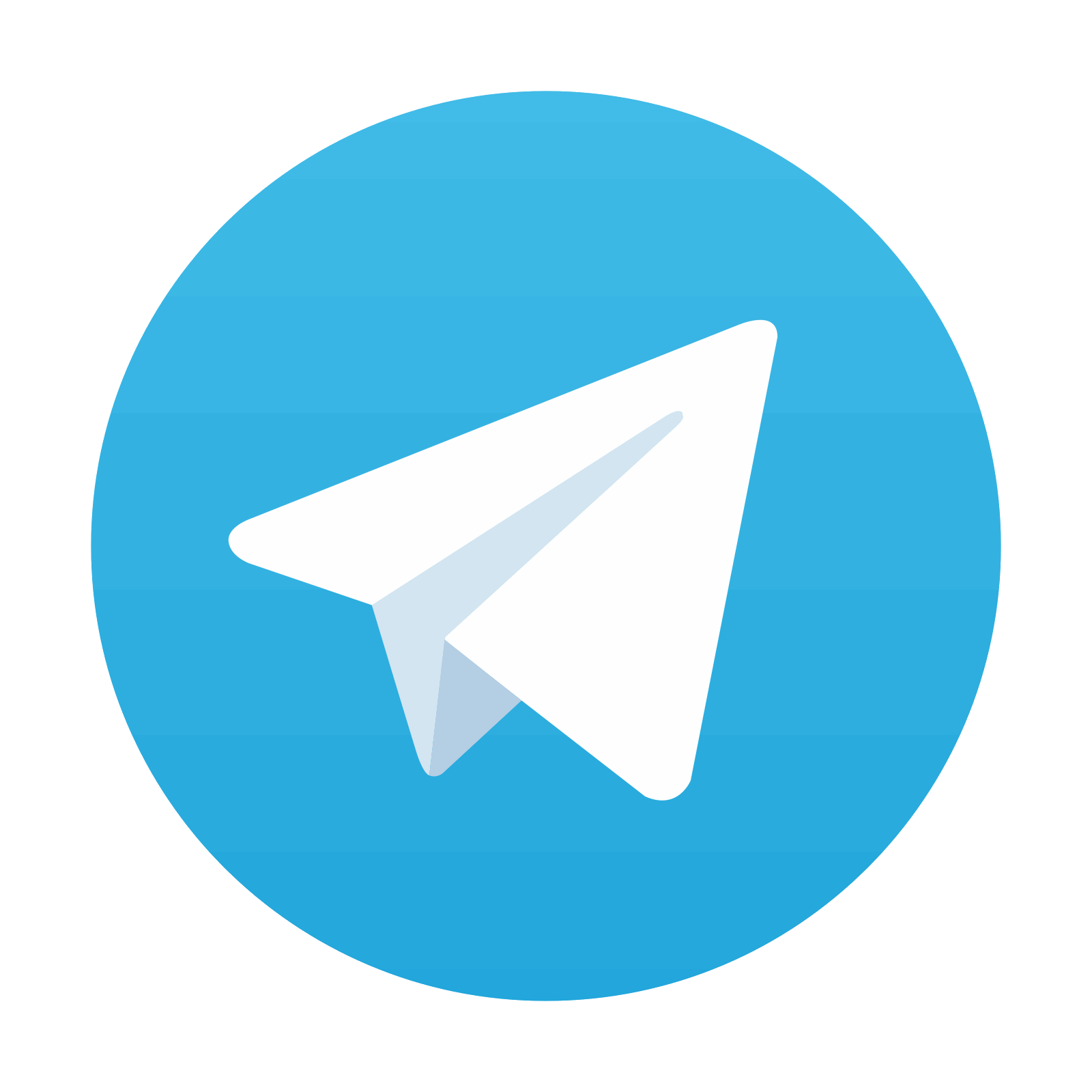