Hypothalamic hormone
Main pituitary hormone
Target organs
Target hormones
CRH
Increases ACTH
Adrenal gland
Increases cortisol, DHEA, aldosterone, 17-hydroxyprogesterone
GHRH
Increases GH
Liver
Increases IGF-1
Somatostatin
Inhibits GH
GnRH
Increases LH/FSH
Ovaries, testes
Increases progesterone, estradiol, testosterone
TRH
Increases TSH
Thyroid, bone
Increases T3, T4
Serotonin and opiates
Increase prolactin
Mammary gland
Increases PTHrP
Dopamine
Inhibits prolactin
Oxytocin
Uterus, mammary gland, ovary, testes, hippocampus, amygdala
Increases prolactin, LH, FSH, oxytocin, ANP
Inhibits GH, TSH, ADH
ADH
Kidneys, CNS
Increases ACTH secretion
3.1 Anterior Pituitary
The cells of the anterior lobe constitute 80 % of the pituitary gland by weight. The hypothalamic neurons that produce SRIF, GHRH, CRH, TRH, and GnRH send their axons through the median eminence to release these hormones into the hypophyseal portal circulation, which surrounds the pituitary stalk and penetrates the anterior lobe of the pituitary. These hypothalamic neurohormones stimulate and modulate the anterior pituitary cells to secrete GH, ACTH, TSH, LH, and FSH. Four glycoproteins—LH, FSH, TSH, and human chorionic gonadotropin (hCG)—share structural homology, having evolved from a common ancestral gene [1]. They all share an alpha subunit, whereas the beta subunit of each hormone is unique. SRIF is produced in the hypothalamus and works in concert with GHRH to modulate GH secretion from the pituitary. Approximately 50 % of cells of the anterior pituitary are somatotrophs that produce GH [2]. These cells are predominantly located in the lateral wings of the anterior lobe, but they can also be scattered in the median wedge. Prolactin-secreting lactotrophs account for 15 % of the cells in the anterior pituitary. Lactotrophs are randomly distributed throughout the lobe, but most are in the posteromedial and posterolateral portions [2]. Corticotrophs comprise 15 % of anterior pituitary cells; they mostly cluster in the central mucoid wedge but are also scattered in the lateral wings [2]. Thyrotroph cells comprise approximately 5 % of functional anterior pituitary cells and are situated predominantly in the anteromedial areas of the gland [1]. Finally, gonadotrophs, which produce LH and FSH, represent 5–15 % of the human anterior pituitary cell population [3].
3.1.1 Luteinizing Hormone and Follicle-Stimulating Hormone
The gonadotropins LH and FSH are produced by gonadotroph cells of the anterior pituitary in response to pulsatile secretion of GnRH from the hypothalamus [3]. GnRH is a 10-amino acid peptide that is released in synchronized pulses from hypothalamic nerve endings into the hypophyseal portal system every 30–120 min [4]. GnRH half-life is about 2–4 min [5]. The gonadotropins regulate gonadal steroid production and secretion and are responsible for germ cell development and the secretion of peripheral sex hormones. Although LH and FSH are co-secreted by the gonadotroph cells, their secretion is governed separately and their serum levels are not always concordant. The regulation of LH and FSH secretion is complex and corresponds to developmental age- and gender-specific feedback mechanisms. Several factors feed back to the hypothalamus, ultimately converging their signaling to the GnRH neurons, which integrate these stimuli to drive gonadotropin secretion.
Feedback inhibition of the gonadal axis is sexually dimorphic, but in general it is mediated by sex steroids and gonadal peptides that are secreted in response to LH and FSH secretion. In men, LH secretion is mediated by testosterone and by estradiol after aromatization of testosterone, at the level of the pituitary and hypothalamus [6, 7]. FSH secretion in men is controlled by both steroid hormones and inhibin B, a peptide secreted by germ cells in response to gonadotropins, through direct GnRH inhibition [8]. In men, LH induces intratesticular testosterone synthesis in the Leydig cells, and FSH mediates spermatogenesis in concert with testosterone.
In women, the feedback mechanisms responsible for the menstrual cycle and ovulation vary according to the phases of the menstrual cycle. In the early follicular phase, estradiol, progesterone, and inhibin A levels are low, leading to increased GnRH pulsation and a consequent small rise in FSH [9]. As the follicular phase progresses, increasing levels of estradiol feed back to the pituitary and hypothalamus to suppress FSH and LH secretion [10]. Mid-cycle, peripheral suppression of gonadotropins shifts to a transient positive feedback effect resulting in the LH surge [11]. The LH surge causes ovulation and leads to increased progesterone and inhibin A secretion with increased feedback inhibition to the gonadotrophs [12]. As the luteal phase progresses, peripheral progesterone and estradiol levels fall, resulting in the onset of menses and the release of FSH inhibition, allowing the cycle to begin again.
Other principal factors in gonadotropin feedback include stimulation of GnRH development and action by kisspeptin, via interaction with the orphan G protein-coupled receptor 54 (GPR54), and leptin, a product of adipose tissue that has stimulatory effects on GnRH pulsation [13, 14]. With the onset of menopause, gonadotropins rise in response to the decreased peripheral production of sex steroids.
Several pathophysiologic and physiologic factors can influence gonadotropic function or lead to gonadotropin deficiency. Deficiencies can be either congenital or acquired and can vary in severity from mild to complete gonadal dysfunction. Central congenital causes include a variety of genetic mutations involved in migration or function of GnRH neurons and the development and function of the gonadotroph cells. Functional causes are numerous; they include excessive exercise, stress, malnutrition, obesity, chronic illness, depression, and medications including opiates and antidepressants. Tumors, infiltrative diseases of the pituitary and hypothalamus, trauma, and malignancies also can lead to hypogonadotropic hypogonadism.
3.1.2 Thyroid-Stimulating Hormone
TSH is a glycoprotein hormone comprising a heterodimer of noncovalently linked alpha and beta subunits [1]. TRH induces TSH alpha and beta subunit gene transcription [1]. TRH is derived from pro-TRH by prohormone convertases. Once it is released from the paraventricular nucleus of the hypothalamus to the median eminence, it reaches the pituitary and stimulates the synthesis and release of TSH [15]. TSH binds to the TSH receptor, a seven-transmembrane glycosylated G protein-coupled receptor, resulting in thyroid follicular growth and thyroid hormone secretion from the thyroid gland [16]. TRH has a half-life of 2–6 min, and TSH can be seen as quickly as 30 min after administration of TRH [17]. Daily TSH production is about 100–400 mIU, with a half-life of 30–50 min [17]. TSH secretion is pulsatile, with pulses occurring every 2–3 h. Peak TSH secretion occurs between 11 PM and 5 AM [1]. TSH stimulates the thyroid to secrete the prohormone thyroxine (T4) and, to a lesser extent, the hormone triiodothyronine (T3) [18]. Most T3 is generated by peripheral conversion of T4 by intracellular iodothyronine deiodinases [18]. In normal conditions, production of T3 and T4 exerts a negative feedback control on the level of the hypothalamus and the pituitary [19]. T3 inhibits TRH gene transcription, as well as inhibiting TSH synthesis and release [2].
Several biological phenomena can affect TSH secretion. TSH production rises with age, but this phenomenon can be confounded by many situations that inhibit TSH secretion in the elderly, such as decreased exercise, reduced caloric intake, exposure to glucocorticoids, traumatic brain injury, and psychiatric depression [20]. Pharmacologic doses of glucocorticoids can inhibit basal TSH secretion and abolish the circadian TSH rhythm [21]. Furthermore, in severe and prolonged nutritional restriction, as in anorexia nervosa, increased cortisol secretion may contribute to the suppression of TSH release [21]. In humans, food deprivation results in several neuroendocrine responses [22]:
Increasing ACTH and cortisol
Decreasing thyroid hormone concentrations, thereby slowing metabolic rate
Increasing GH concentration, thereby mobilizing energy stores
Decreasing insulin growth factor 1 concentration, thereby slowing energy-demanding growth-related processes
Decreasing reproductive hormone concentrations, thereby reducing fertility and preventing pregnancy
Leptin, discovered as a factor produced by adipose tissue, induces satiety and is an indicator of the body’s nutritional status [17]. Leptin is a 167-amino acid peptide that is produced predominantly in the adipose tissue but is also expressed in the placenta, ovaries, mammary, epithelium, bone marrow, and lymphoid tissues [22]. Leptin replacement during fasting prevents the starvation-induced changes in the hypothalamic-pituitary-thyroid axis [22]. Several studies have shown that leptin is involved in regulating the release of TRH from the paraventricular nucleus, as low levels of circulating leptin are accompanied by a decrease in TRH mRNA [23]. Fasting and infection upregulate type II deiodinase expression, resulting in a local increase of T3, which may explain a reactive decrease in TSH observed during fasting or inflammatory diseases [2]. In contrast, chronic fatigue syndrome, morbid obesity, and diabetes type 2 may increase TSH secretion [20].
3.1.3 Prolactin
Prolactin (PRL) is a 199-amino acid polypeptide that circulates in blood in various sizes, including 23-kd monomeric prolactin, 48- to 56-kd dimeric prolactin (“big prolactin”), and polymeric complexes larger than 100 kd, referred to as “big, big prolactin” [1]. The monomeric form is the most bioactive form. PRL secretion differs from the secretion of other anterior pituitary hormones in that it is not regulated by negative feedback from peripheral hormones. PRL is regulated by tonic inhibition from dopaminergic neurons from the hypothalamus. Dopamine is secreted into the portal system and inhibits PRL through binding to type 2 dopamine (D2) receptors on the lactotroph cell surface. Normal daily PRL production ranges from 200 to 500 μg/day per square meter of body surface area [24]. It is secreted in 4–14 secretory pulses over 24 h, each lasting 67–76 min [25, 26]. The half-life of PRL ranges from 26 to 47 min. The highest levels occur during sleep, and the lowest levels occur between 10 AM and noon [24]. Levels are higher in women than in men, are higher during ovulation, and fall with age in both men and women [20]. After menopause, PRL secretion during nighttime falls by 40 % [20].
Lactotroph cells of the anterior pituitary are the main source for circulating PRL, but other sources of production include lymphocytes, skin fibroblasts, brain, breast, decidua, prostate, and adipose tissue cells [27]. The main function of PRL in humans is to stimulate milk production, but it is also involved in mammary development, melanin synthesis, and parental behavior [1]. During pregnancy, increasing estrogen secretion stimulates the growth and proliferation of the lactotrophs; as a result, PRL secretion increases [27]. The size of the pituitary doubles during pregnancy, owing to hyperplasia and hypertrophy of lactotrophs [27]. The absolute number of lactotrophs does not change during pregnancy, however, and the lactotroph hyperplasia resolves within several months after delivery [1]. During pregnancy, PRL, together with estradiol, progesterone, placental lactogen, insulin, and cortisol, cause mammary gland growth and development [27]. Prolactin prepares the mammary gland for postpartum lactation, but the high estrogen concentration during pregnancy inhibits the lactation effect in the mammary gland while the woman is still pregnant [27]. Then, as estrogen levels fall after delivery, the initiation of lactation can occur [27]. After delivery, suckling is essential for the continued secretion of PRL; if the woman does not breast-feed, PRL levels can return to normal within 7 days [1]. Suckling stimulates neurons of the hypothalamus to secrete opioids, which in turn decrease dopamine secretion in the hypothalamus and relieve the lactotroph cells from dopaminergic inhibition [27]. During lactation, mobilization of bone is critical to ensuring availability of large amounts of calcium exported into milk [28]. PRL helps this to occur by indirectly allowing for the secretion of parathyroid hormone-related peptide (PTHrP). PRL causes dilatation of the mammary gland alveoli, which in turn synthesize serotonin in the mammary epithelial cells [28]. Serotonin then controls the expression of PTHrP in the mammary glands [28]. PTHrP is secreted in large quantities from lactating mammary glands, causing bone resorption [28]. Elevated PRL during and immediately after pregnancy also inhibits ovulation by suppressing LH and FSH secretion [27]. Similarly, feedback to the hypothalamus from pathologic hyperprolactinemia of any etiology can inhibit GnRH-mediated LH and FSH secretion, thereby inducing a hypogonadotropic hypogonadism [29].
Beyond elevations seen during pregnancy, PRL production is stimulated by many other factors, including psychological and physical stress, breast stimulation, hypoglycemia, estrogen, use of dopamine-2-receptor antagonist medications, sexual activity, hyperthermia, sleep, food intake, opiates, and exercise [1]. Some hormones that stimulate secretion include TRH, oxytocin, ghrelin, vasoactive intestinal polypeptide (VIP), epidermal growth factor, serotonin, angiotensin II, estradiol, oxytocin, galanin, and GHRH at high doses [27]. Estrogen increases PRL secretion mainly by reducing expression of lactotroph dopamine receptors [2]. In rats, a short feedback loop for PRL has been described, with PRL increasing tyrosine hydroxylase activity in the tuberoinfundibular neurons [1]. Other than dopamine, growth factor B1, noradrenaline, gamma-aminobutyric acid (GABA), serotonin, histamine, somatostatin, chromogranin, orexin-A, cortistatin, nitrogen oxide, and endothelin-1 also inhibit prolactin secretion [27].
Abnormal elevations of PRL secretion can arise from prolactinomas (benign pituitary adenomas derived from lactotroph cells) or can be due to non-prolactinoma tumors located in the sellar and parasellar regions through the so-called stalk effect, in which PRL is secreted from nontumor cells when dopaminergic inhibition is interrupted. Drugs, inflammation (hypophysitis), neurogenic chest wall stimulation, and reduced PRL clearance are other causes of hyperprolactinemia. PRL deficiency is rare and can be due to trauma or inflammatory or genetic causes.
3.1.4 Growth Hormone
Somatotroph cells from the anterior pituitary secrete GH, a hormone principally responsible for linear growth during development, but with many other metabolic functions. GH circulates primarily as a 22-kD, 191-amino acid, single-chain peptide bound to GH binding protein. GH secretion is normally episodic and exhibits a diurnal rhythm with approximately two thirds of the total daily GH secretion occurring at night, triggered by the onset of slow-wave sleep [1]. Normal GH secretion is characterized by secretory episodes separated by troughs of minimal basal secretion during which GH is undetectable [1].
GH secretion is regulated by hypothalamic secretion of growth hormone-releasing hormone (GHRH) and somatostatin (SRIF) [30]. Several hypothalamic factors influence SRIF and GHRH secretion; the secretion of these hormones in concert determines the pulsation frequency and amplitude of GH secretion [31]. The somatotroph cell responds to signaling from GHRH, SRIF, and growth hormone secretagogues to secrete GH. SRIF, a cyclopeptide consisting of 14–28 amino acids, is expressed in many tissues throughout the body, including the central nervous system, hypothalamus, gastrointestinal tract, and the pancreas [32, 33].
Once in circulation, GH binds to GH receptors to stimulate production and secretion of insulin-like growth factors (IGFs), of which IGF-1 is the principal target growth factor [31]. IGF-1 circulates bound to IGF-binding proteins and participates in feedback inhibition of GH secretion via inhibition at the level of the pituitary and stimulation of SRIF release [34].
GH concentration is high in fetal circulation, falls during early childhood, and then rises again at the onset of puberty [1]. Daily production of GH in the prepubertal state is 200–600 μg, which then rises to 1000–1800 μg at the pubertal peak [31]. Secretion of GH then proceeds to decline progressively throughout adulthood [35]. Adiposity that accompanies the aging process accounts for a significant component of the decline in GH output with increasing age [36]. Basal and stimulated GH levels can be reduced in patients with obesity, especially visceral adiposity [37]. Aged adults secrete less GH than do young individuals during fasting, exercise, or sleep, in response to nearly all secretagogues [20].
IGF-1 is produced mainly in the liver in response to GH and is a mediator of GH effects on protein anabolism [1]. IGF-1 strongly stimulates protein synthesis and inhibits protein breakdown [38]. This action is especially relevant in situations of starvation, in which GH and IGF-1 secretion both limit catabolism of protein and instead encourage the mobilization of fat stores [39]. GH has been shown to promote fat metabolism by enhancing lipolysis and fatty acid oxidation [38]. In this situation, elevation of free fatty acids (FFAs) then acts as a negative feedback to suppress further GH secretion [40].
Ghrelin, which is produced in the gastric mucosal neuroendocrine cells and in the hypothalamus, binds to the GH secretagogue receptor (a receptor that was discovered before the endogenous ligand ghrelin) to induce GH release [41, 42]. Ghrelin concentrations have been shown to be decreased in situations where GHRH expression is muted, such as in cases of glucocorticoid excess, hyperthyroidism, and food deprivation [23]. Ghrelin specifically is involved in encouraging appetite and increased food intake [43]. Once ghrelin levels rise in anticipation of food intake, GH is produced, which then exerts some insulin-like effects, stimulating amino acid uptake and protein synthesis [44]. However, in opposition to insulin, GH suppresses glucose oxidation and utilization while enhancing hepatic glucose production [38]. It has long been known that there is a relationship between glucose metabolism and GH secretion [45]. This relationship has been capitalized upon to develop testing for GH deficiency with insulin-induced hypoglycemia and testing for acromegaly with suppression of GH with glucose administration [45, 46].
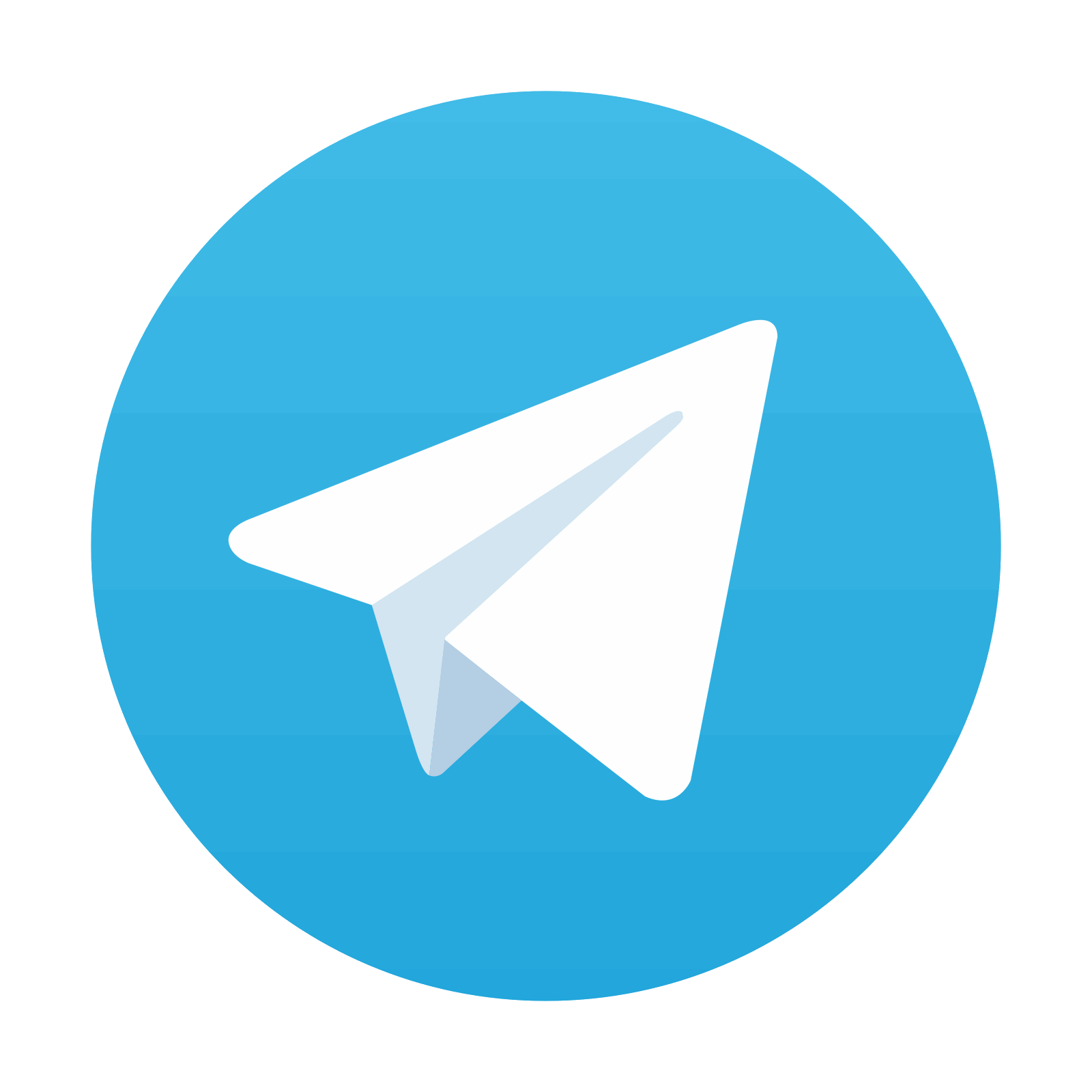
Stay updated, free articles. Join our Telegram channel

Full access? Get Clinical Tree
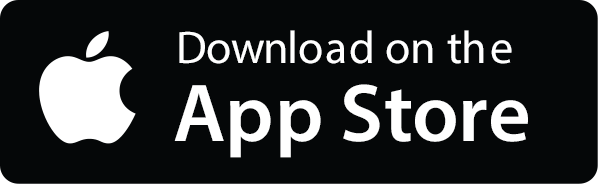
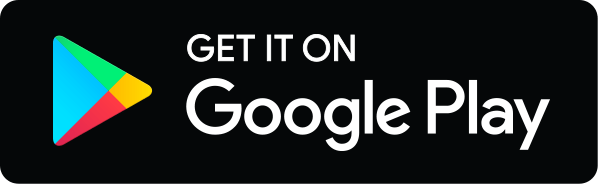