Postoperative Spinal Imaging
Ashwini D. Sharan
Hana Choe
Laura Snyder
The goal of this chapter is to present a surgeon’s approach to management of the postsurgical spinal trauma patient and inherent complications. The abnormal postoperative findings that require further investigation are reviewed, such as spinal deformity, nonunion, graft-failure, pseudoarthrosis, and instability. Also, unexpected neurologic deficits or unresolved pain mandate an evaluation in an acute, subacute, or chronic setting. In many instances, imaging studies are helpful in confirming the existence of postoperative complications such as hematomas, infection, pseudomeningocele, or arachnoiditis. Other chronic sequelae such as syringomyelia, myelomalacia, and spinal cord tethering are discussed in Chapter 11.
Immediate Postoperative Evaluation
Before the patient leaves the operating room, graft position and operative levels must be confirmed radiographically. Radiographs in both anteroposterior (AP) and lateral views are excellent for grossly assessing spinal alignment, hardware placement, and positioning of interbody and graft material (Figs. 10-1, 10-2 and10-3). Radiographs also provide a good, reliable, and reproducible means to follow normal sagittal balance, kyphosis, and translation. An abnormality in any one of these measures may warrant further intervention. Malpositioned hardware may be surgically modified before concluding the procedure, or may lead to supplemental imaging in the immediate postoperative period (Fig. 10-4). Immediate postoperative radiographs serve as a reference point in the event of hardware failure or delayed posttraumatic deformity.
All postoperative spine patients must be neurologically assessed while in the surgical recovery area to determine that there has been no deterioration in preoperative neurologic status (i.e., new motor weakness or sensory disturbance). In addition, spine surgery patients are commonly assessed in the operative suite for inadvertent neurologic damage by monitoring motor and sensory evoked potentials. These physiologic parameters are followed closely during surgery and may influence surgical technique to avoid further neurologic injury.
Assessment of Fusion/Graft Stability
Whenever possible, during interval follow-up, the fused segment of the spine should be evaluated with radiographs obtained with the patient upright and compared with those performed immediately after surgery. Weight bearing is an important part of the postoperative assessment as it can provide early indication of progressive deformity and
instability at the fusion site. The Cobb angle (Fig. 10-5) is usually used as a measure of the curvature of the spine at the fracture site. The angle between intersecting lines drawn perpendicular to the top of the top vertebrae above the fracture site and the bottom of the bottom vertebrae is the Cobb angle. A minor change in the angle may suggest progression of kyphosis and the need for surgical intervention and further stabilization. Moreover, extreme kyphosis, inadequate correction of kyphotic deformity, or subluxation has been cited to cause strut graft failure. Graft fracture, malposition, and dislodgment are obvious evidence of failure of the surgical construct. Furthermore, the vertebral body onto which the graft is seated can fracture, especially in the setting of pre-existent osteoporosis. If this occurs when the fracture is
minimally displaced and the graft position is still satisfactory, immobilization with a halo vest and close observation may be all that is required. If no further displacement occurs and there is no kyphosis, the fracture is likely to heal spontaneously. However, many times, strut graft failure results in the implantation of a longer graft and extending the fusion one more level. If questions remain regarding the quality of the bone or stability of the construct, supplemental posterior stabilization is recommended.
instability at the fusion site. The Cobb angle (Fig. 10-5) is usually used as a measure of the curvature of the spine at the fracture site. The angle between intersecting lines drawn perpendicular to the top of the top vertebrae above the fracture site and the bottom of the bottom vertebrae is the Cobb angle. A minor change in the angle may suggest progression of kyphosis and the need for surgical intervention and further stabilization. Moreover, extreme kyphosis, inadequate correction of kyphotic deformity, or subluxation has been cited to cause strut graft failure. Graft fracture, malposition, and dislodgment are obvious evidence of failure of the surgical construct. Furthermore, the vertebral body onto which the graft is seated can fracture, especially in the setting of pre-existent osteoporosis. If this occurs when the fracture is
minimally displaced and the graft position is still satisfactory, immobilization with a halo vest and close observation may be all that is required. If no further displacement occurs and there is no kyphosis, the fracture is likely to heal spontaneously. However, many times, strut graft failure results in the implantation of a longer graft and extending the fusion one more level. If questions remain regarding the quality of the bone or stability of the construct, supplemental posterior stabilization is recommended.
Utility of Dynamic Imaging in the Postoperative Period
Dynamic images are functional radiographs obtained in at least two extremes of normal physiologic motion (e.g., flexion and extension) to provide critical information regarding presence stability. Dynamic images are of limited usefulness in the immediate postoperative period for several reasons: (a) surgical manipulation of the spine is associated with pain and muscle spasm, which limit the normal range of movement and act as a brace to stabilize the spine; and (b) patients are commonly immobilized with external orthotic devices such as a halo vest, hard cervical collar, or thoracolumbo-sacral orthotic (TLSO) brace. Thus, dynamic imaging may only be useful many months later. Sequential flexion-extension x-ray films are useful for the assessment of strut graft failure.
Computed Tomography in the Postoperative Spine
Computed tomography (CT) scans are informative postoperatively particularly after deployment of instrumentation to precisely define hardware positions in relation to the pedicles or lateral masses. In general, spinal hardware malposition does not correlate with neurologic complications; however, identification of an osseous breech warrants
investigation and sometimes intervention. A medial breech of the pedicle from inaccurate pedicle screw placement can potentially compromise the spinal canal and contents (spinal cord or cauda equina), and an inferior cortex pedicle breach places the nerve root at risk for injury. A lateral breech of a cervical lateral mass screw might encroach upon the vertebral artery, while a lateral breech of a thoracic pedicle screw may compromise the aorta. A screw that is inadvertently placed through an adjacent disc space may result in injury to that disc. These findings may be visible on radiographs, but a CT scan with reconstruction of the images gives better anatomical delineation of the hardware as well the relationship of the hardware to the normal anatomy. Moreover, the higher in-plane resolution afforded with modern multidetector CT equipment actually minimizes the image degradation commonly associated with imaging of metallic hardware. If a clinically significant malposition is identified, then the patient may need to return to the operating room to have the hardware repositioned.
investigation and sometimes intervention. A medial breech of the pedicle from inaccurate pedicle screw placement can potentially compromise the spinal canal and contents (spinal cord or cauda equina), and an inferior cortex pedicle breach places the nerve root at risk for injury. A lateral breech of a cervical lateral mass screw might encroach upon the vertebral artery, while a lateral breech of a thoracic pedicle screw may compromise the aorta. A screw that is inadvertently placed through an adjacent disc space may result in injury to that disc. These findings may be visible on radiographs, but a CT scan with reconstruction of the images gives better anatomical delineation of the hardware as well the relationship of the hardware to the normal anatomy. Moreover, the higher in-plane resolution afforded with modern multidetector CT equipment actually minimizes the image degradation commonly associated with imaging of metallic hardware. If a clinically significant malposition is identified, then the patient may need to return to the operating room to have the hardware repositioned.
Magnetic Resonance Imaging in Postoperative Spine Trauma
Magnetic resonance imaging (MRI) is used in the examination of both the early and late postoperative spine when the patient has recurrent or persistent symptoms or new deficits. When decompression of the spinal cord is the goal of surgical intervention, a postoperative MRI is a necessary assessment tool to evaluate the adequacy of decompression, the integrity of spinal cord and canal anatomy, as well as to gain insight into the patient’s functional prognosis. High bandwidth MRI techniques tend to decrease metallic artifact after arthrodesis and improve image quality. Even with these image improvements, there is often sufficient artifact from the instrumentation to obscure soft tissue details in the neural foramina (Fig. 10-6).
Postoperative Complications in Spinal Trauma
Patients with spinal trauma are subject to the additional comorbidities associated with multisystem trauma. Thus, while the average length of stay for all causes of hospital admissions is 4.9 days, the average length of initial hospitalization following spinal cord injury (SCI) in acute care units is 19 days. This extended hospitalization for the typical SCI patient is attributed to the severity of the initial injuries and the inherent comorbidities associated with loss of normal physiologic functions.
Acute Complications
The most devastating complication of spinal trauma is the development of a new neurologic deficit. When the
neurologic deficit occurs immediately following the surgical intervention or shortly thereafter, the etiology is likely due to iatrogenic spinal cord injury, ischemia, or bleeding. The deficit may result from direct injury to the spinal cord or nerve roots or may occur secondary to a vascular injury, such as injury to the vertebral artery. Sudden onset of spinal cord compression from a hematoma or retained disc or bony fragment can also produce late neurologic complications. Direct surgical causes of neurologic injury include malalignment of the vertebral column, malposition of the hardware, anesthetic-related fluctuations in the blood pressure, or a postoperative hematoma/fluid collection (Fig. 10-7).
neurologic deficit occurs immediately following the surgical intervention or shortly thereafter, the etiology is likely due to iatrogenic spinal cord injury, ischemia, or bleeding. The deficit may result from direct injury to the spinal cord or nerve roots or may occur secondary to a vascular injury, such as injury to the vertebral artery. Sudden onset of spinal cord compression from a hematoma or retained disc or bony fragment can also produce late neurologic complications. Direct surgical causes of neurologic injury include malalignment of the vertebral column, malposition of the hardware, anesthetic-related fluctuations in the blood pressure, or a postoperative hematoma/fluid collection (Fig. 10-7).
Hypotension and Ascending Spinal Cord Injury
Harrop et al. (1) found that 12 of 186 or 6% of patients with American Spinal Injury Association Impairment Scale (ASIA) A complete spinal cord injuries worsened in the first 30 days after injury. Progression of the injury characteristically occurred along a temporal continuum and was classified into three discrete time intervals: early, delayed, and late deterioration. Early deterioration (<24 hours) is typically related to traction and immobilization. National spinal cord injury data suggest that the use of standardized resuscitative protocols have improved the incidence of early deterioration in victims of spinal cord injuries. In contrast to early deterioration, delayed deterioration occurs between 24 hours and 7 days from time of injury, often as a result of sustained hypotension in patients with fracture dislocations.
SCI patients are predisposed to hypotension due to loss of normal vasomotor tone in the peripheral arterioles and subsequent pooling of blood in the peripheral vasculature. Therefore, the first line of treatment for vasomotor hypotension is volume resuscitation. Given that there is evidence for secondary ischemic injury in SCI patients, maintaining appropriate blood pressure to perfuse the injured spinal cord is paramount. Less well known is the optimal pressure to sustain perfusion to the spinal cord, and there is no clear way to measure spinal cord perfusion (2). Vale et al. (3) treated a series of patients with acute SCI with fluid and vasopressors to achieve a mean arterial pressure (MAP) of 85 mm Hg for a minimum of 7 days and reported favorable neurologic outcomes. However, the chosen MAP was arbitrary and the study uncontrolled; thus, the optimal value remains unknown.
Acute SCI, in particular cervical SCI, patients are especially prone to hemodynamic instability (2). This occurs when sympathetic fibers exiting the spinal cord in the thoracic region are interrupted. Parasympathetic outflow becomes unopposed, which results in cardiac arrhythmias and hypotension. The most common arrhythmia seen is bradyarrhythmia, which further exacerbates hypoperfusion and loss of sympathetic tone. Arrhythmias appear to be most common within the first 14 days after injury and are more common in neurologically complete injuries (4).
Ascending spinal cord injury is the rare instance in which a patient spontaneously ascends in their neurologic level of injury. In the acute period, this can occur within the first few days after the initial injury. Though many etiological factors have been implicated in ascending spinal cord injury, the cause frequently remains elusive. One factor that is consistently implicated has been the maintenance of blood perfusion in the first 48 to 72 hours after injury and particularly during surgery. Intraoperative events that cause new deficits are rare and may be monitored during surgery with electrophysiological motor and sensory feedback. Further, strict adherence to minimum blood pressure or MAP parameters with aggressive fluid or cardiac vasopressor administration can often prevent untoward events. Anecdotal reports have described adverse changes in intraoperative electrophysiological monitoring that returns to baseline levels with aggressive fluid resuscitation and maintenance of MAPs to at least greater than or equal to 85 mm Hg.
Postoperative Hematoma
It is extremely difficult to obtain absolute hemostasis in the postsurgical bed. Tissue changes from the initial traumatic injury and surgical dissection will cause venous blood to drain from epidural veins, decorticated bony edges, and manipulated muscles. Hemorrhages of this type
typically do not progress to symptomatic hematomas as a small bleeding vein will likely tamponade from the pressure of the surrounding soft tissue structures. Nevertheless, there will always appear to be some blood in the postoperative surgical bed, and delayed epidural hematomas can develop. Risk factors identified in the development of epidural hematomas include prior surgery with resultant scarring, multilevel laminectomies, ankylosing spondylitis, and preoperative coagulopathy (5, 6).
typically do not progress to symptomatic hematomas as a small bleeding vein will likely tamponade from the pressure of the surrounding soft tissue structures. Nevertheless, there will always appear to be some blood in the postoperative surgical bed, and delayed epidural hematomas can develop. Risk factors identified in the development of epidural hematomas include prior surgery with resultant scarring, multilevel laminectomies, ankylosing spondylitis, and preoperative coagulopathy (5, 6).
A new neurologic deficit, whether immediate or delayed, mandates follow-up imaging on an emergent or urgent basis, in accordance with the acuity of the deterioration. The outcome of spinal epidural hematomas is purportedly influenced by two factors: the accuracy of the diagnosis and the time interval between the onset of symptoms and surgical decompression (7). The more rapid the decompression, the better the therapeutic outcome. Imaging confirmation of an epidural hematoma is best verified with MRI. In instances where MRI is not available or is contraindicated, myelography with postmyelography CT is the best second imaging approach. In certain instances of high clinical suspicion and when the change in neurologic status is substantial, surgical exploration without imaging may be favored over delays imposed by emergent imaging. In one study, emergent surgical decompression of a spinal epidural hematoma within 12 hours of progression to complete paraplegia provided full resolution of the symptoms, while symptom duration of 72 hours or greater did not improve after surgical intervention (8). Although most epidural hematomas will occur within 24 hours of the surgery, in one report, hematoma developed .3 days after the index procedure (9). The most common presenting symptom was severe, sharp pain radiating down to the extremities with neurologic deterioration. In all cases, MRI was used to document the presence of an epidural hematoma. Surgical evacuation with improvement in symptoms occurred in 4 out of 6 patients (9).
It should be noted that we have seen CSF leaks underlying an epidural hematoma which the patient was clinically completely asymptomatic (Fig. 10-8). Thus, the radiographic relevance of a postoperative hematoma must be made in light of the clinical scenario.
Subacute Complications
Infection
The diagnosis and management of a postoperative infection is complex. A constellation of clinical findings supplemented by radiographic evidence can suggest the diagnosis, but frequently a patient will need a tissue biopsy to identify the pathogen. The incidence of infection in the postoperative spinal trauma patient has been documented to be as high as 9.4% (10). The immobilized postsurgical patient is susceptible to urinary tract infections secondary to Foley catheters and urinary stasis, prolonged ventilator dependence, pneumonia, and atelectasis with prolonged bed rest, deep venous thrombosis, and wound infections. A patient’s nutritional status is a key factor in the risk of development of an infection. The clinical parameters most
commonly used to assess the nutritional status of surgical patients are the serum albumin level and the total lymphocyte count (TLC). Serum albumin is an index of visceral protein mass, and decreased levels are associated statistically with poor wound healing, postoperative infectious, complications, mortality, and immune suppression (11). he total lymphocyte count is a marker of immune competence and is decreased in malnourished patients. Malnutrition depresses immunity by impairing chemotaxis and phagocytosis. Serum albumin <3.5 g/dL and TLC <1,500 to 2,000 cells/mm3 are considered by most authors to represent clinical malnutrition. Moreover, SCI patients tend to be in a highly catabolic state after spinal cord injury, which exacerbates the malnutrition and sets the stage for poor wound healing and postoperative wound infections.
commonly used to assess the nutritional status of surgical patients are the serum albumin level and the total lymphocyte count (TLC). Serum albumin is an index of visceral protein mass, and decreased levels are associated statistically with poor wound healing, postoperative infectious, complications, mortality, and immune suppression (11). he total lymphocyte count is a marker of immune competence and is decreased in malnourished patients. Malnutrition depresses immunity by impairing chemotaxis and phagocytosis. Serum albumin <3.5 g/dL and TLC <1,500 to 2,000 cells/mm3 are considered by most authors to represent clinical malnutrition. Moreover, SCI patients tend to be in a highly catabolic state after spinal cord injury, which exacerbates the malnutrition and sets the stage for poor wound healing and postoperative wound infections.
About 75% of spinal cord injury patients (primarily young, otherwise healthy men), become malnourished in the postoperative period as studied with serum albumin and TLC parameters. Further, postoperative complications occurred only in patients who were malnourished (p = 0.001) and not in those nutritionally replete. There was also a statistically significant relationship between nutritional status and the need for prolonged (>24 hours) intubation (p = 0.016) whereby all patients with prolonged intubation were malnourished.
Interestingly, Galandiuk et al. (12) showed that the use of high-dose methylprednisolone (MPS) as part of the treatment for SCI was not related significantly to the incidence of postoperative complications and did not increase the number of complications experienced by patients during their hospitalizations. However, pneumonia
was both more frequent and severe in MPS-treated patients. Though MPS treated patients did have an initially higher earlier boost, in some host defense parameters, they rapidly declined and the subsequent response was both blunted and delayed. In addition, the length of hospital stay was longer in MPS-treated patients than in patients not treated with steroids (44.4 days versus 27.7 days, respectively; p = 0.065) and 79% of patients treated with MPS had pneumonia compared with an incidence of 50% for patients that did not receive the treatment (p = 0.614).
was both more frequent and severe in MPS-treated patients. Though MPS treated patients did have an initially higher earlier boost, in some host defense parameters, they rapidly declined and the subsequent response was both blunted and delayed. In addition, the length of hospital stay was longer in MPS-treated patients than in patients not treated with steroids (44.4 days versus 27.7 days, respectively; p = 0.065) and 79% of patients treated with MPS had pneumonia compared with an incidence of 50% for patients that did not receive the treatment (p = 0.614).
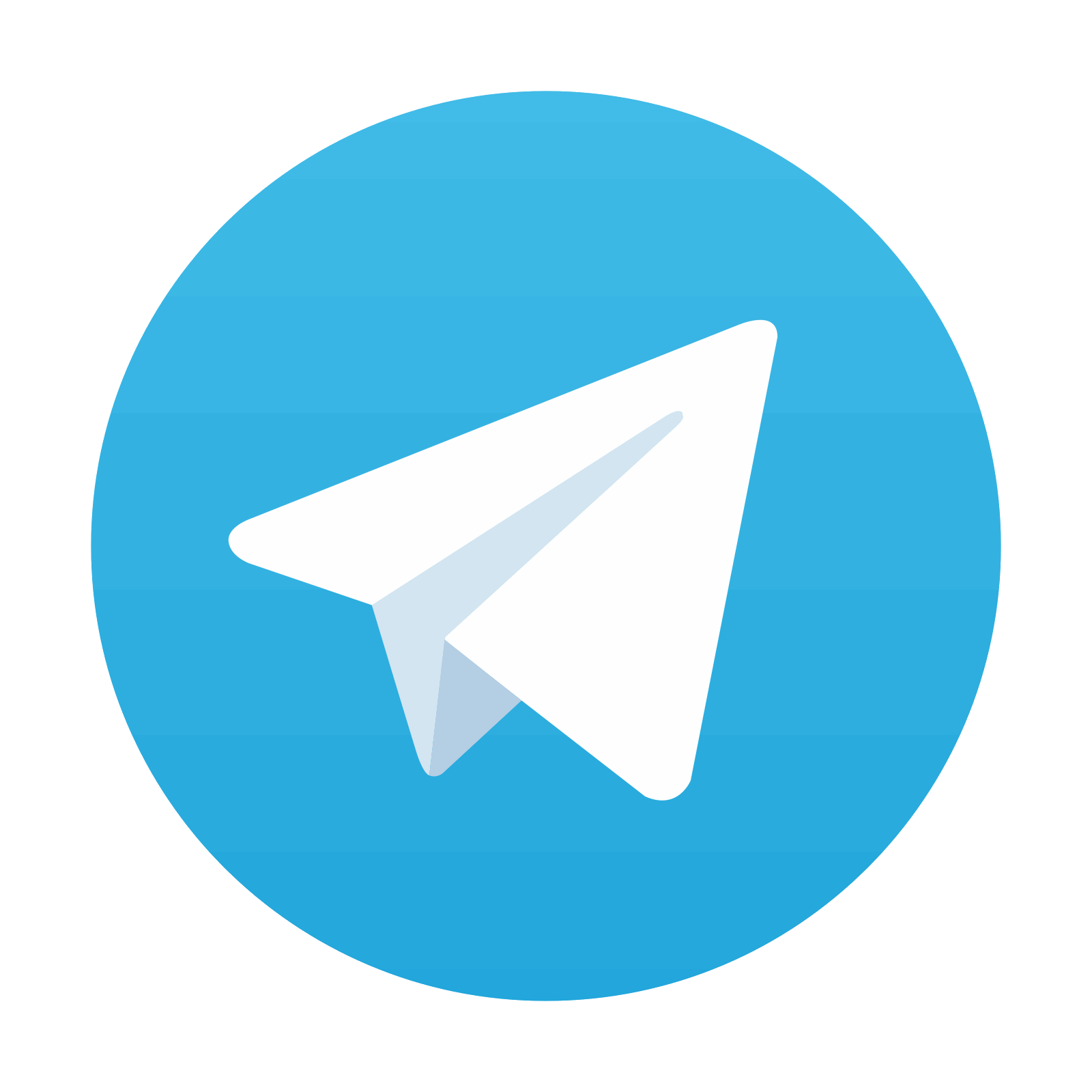
Stay updated, free articles. Join our Telegram channel

Full access? Get Clinical Tree
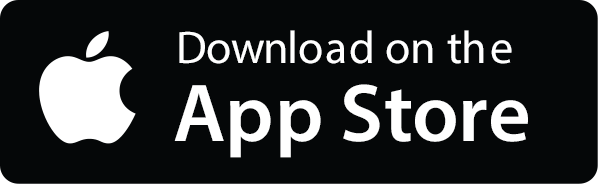
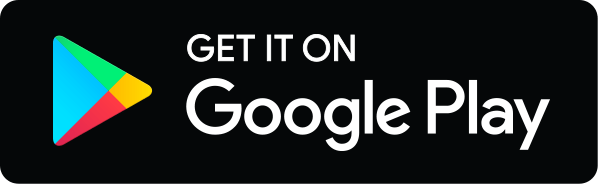
