5 The radiologic evaluation of spinal mass lesions has been evolving since 1980. Refinements in magnetic resonance imaging (MRI) technology have paralleled those in the computer industry, allowing faster, higher-resolution scanning. Computed tomography (CT) has also improved. Helical acquisition and multidetector arrays have replaced planar imaging, allowing rapid three-dimensional (3D) imaging. Combined, these two modalities provide a thorough evaluation of the spine, obviating the need for biopsy in many cases. Despite these technologic improvements, spine physicians are often confronted with patients with a new mass associated with confusing or misleading imaging. Often the most difficult cases are “pseudotumors,” benign conditions mimicking a tumor of the spinal axis. This chapter reviews the available radiologic modalities, emphasizing the utility of each modality to obtain an appropriate differential diagnosis. A practical approach to spinal imaging, including problem-solving imaging algorithms and approaches to confusing imaging scenarios, is offered with appropriate case examples. Radiographic evaluation of the patient with a potential spinal axis tumor is directed toward establishing the presence of disease, characterizing the lesion, and obtaining a differential diagnosis. Among the available imaging modalities, spine physicians need to understand what each imaging procedure offers and need a protocol for evaluating new lesions. Radiographic studies should address several critical questions. First, does the imaging depict disease or a normal variant? Is there a study that will allow the lesion to be followed with a reasonable level of confidence? Is the lesion infectious rather than neoplastic? Is the lesion solitary or multiple, and are there lesions in other organ systems that may be more amenable to biopsy? What other imaging modalities may help answer the above questions? For all lesions, the three-compartment model is useful for limiting the differential diagnosis. As part of the initial evaluation, conventional radiographs are often obtained. Although insensitive for the detection of soft tissue abnormalities, radiography is readily available and offers information about osseous anatomy and alignment, bone sclerosis, and demineralization (Fig. 5-1). Primary bone tumor matrix also may be visualized; occasionally, this information can be useful for identification of chondroid tumors. Shortfalls of radiography include its limited contrast resolution and low sensitivity for vertebral bone loss. Approximately 50% or more trabecular bone must be lost for a vertebral lesion to be evident on a plain radiograph.1 FIGURE 5-1 (A) Anteroposterior image of the thoracolumbar spine shows sclerosis, a poorly defined pedicle, and scoliosis. These findings suggest the diagnosis of osteoid osteoma. (B) An axial CT better demonstrates the nidus of the lesion with mixed lysis and sclerosis. In 1971 Godrey Hounsfield introduced CT. Along with Allan Cormack, he won the 1979 Nobel Prize in Medicine. CT revolutionized diagnostic imaging by enabling two-dimensional (2D) axial anatomic depiction with spatial resolution in the submillimeter range. Earlier technology relied on a single detector array acquiring data in single-slice increments. Spatial resolution and volume of coverage were limited by the inherent inefficiency of step-and-shoot acquisition and the need for tube cooling. In 1988 Siemens Medical Systems introduced single-section, helical CT using slip-ring gantry technology.2 The patient is advanced through the gantry at a constant speed while the single source-detector assembly now rotates continuously. Rather than acquiring a series of 2D axial images, the acquisition data set is volumetric. Thus, CT becomes a true 3D modality capable of high-quality multiplanar and 3D reformatting. Artifact is reduced, patient coverage is increased, and scan time is reduced.2,3 In the 1990s, the introduction of multisection helical CT furthered the capabilities of volumetric CT. Multidetector arrays replaced the single detector, allowing a larger volume of data to be acquired with a thinner slice profile. Current-generation scanners use four data-acquisition systems, generating four channels of spatial data (quad section CT). At the same rotation speed of the gantry, comparable diagnostic quality images can be obtained with three times the volume coverage speed for quad compared with single detector-row CT.4 The improved efficiency of multisection CT permits faster scanning and reduces motion artifacts and the need for sedation in the pediatric population.5 Scanning with thinner collimation reduces partial-volume averaging and improves diagnostic accuracy. High-quality multiplanar reformatting is now used routinely for spinal imaging and CT myelography. The quality of 3D reconstruction is excellent and may aid preoperative planning. Algorithms that may further improve CT of the spine are being developed. When MRI characterizes an osseous lesion inadequately, CT of the spine should be considered. CT is particularly sensitive for evaluating cortical bone, for identifying pathologic fractures, and for characterizing the matrix of primary osseous tumors. CT may be superior to conventional radiography and MRI for detecting certain lesions.6 Myelography and CT myelography (CTM) have long been mainstays of spinal imaging, particularly for evaluating degenerative disease of the spine. Myelography can indirectly evaluate extradural lesions, display the relationship of bony structures to the thecal sac, and evaluate spinal dynamics in upright flexion and extension positions. However, its role in the evaluation of spinal mass lesions is limited and has been largely supplanted by MRI. Myelography is also invasive. It requires dural puncture, which exposes the patient to the risk of postdural puncture headache and other less common risks.7 Myelopathy may worsen in patients with a spinal block. In the era of MRI, CTM should be reserved for patients who are not candidates for MRI because they harbor an implanted device or metallic foreign body or when specific anatomic information about the relationship of bony structures to the thecal sac is needed before surgery. CTM also may help delineate an arachnoid or meningeal cyst that manifests as a mass. CTM may define the communication of the cyst with the remainder of the thecal sac. Since its introduction in the early 1980s, MRI has become the dominant imaging technique for evaluating the spine. MRI is noninvasive and associated with no ionizing radiation. It provides excellent anatomic detail and characterizes tissue in multiple planes. Clinical MRI uses high-power magnetic fields, typically in the range of 0.3 to 1.5 tesla (T); 1 T is ~20,000 times that of the earth’s magnetic field. The magnetic energy is used to align hydrogen (water) protons and causes them to precess (spin) like a child’s top. Water protons spin faster in a 1.5-T MRI system than in a 0.3-T system. Additional energy in the form of radiofrequency (RF) pulses disrupts the spinning protons. When the RF pulse is turned off, the protons return to the lower energy state (equilibrium), releasing measurable energy. This release of energy can be detected, amplified, and converted to form an image. Tissue contrast is determined by the number of protons in the sample (proton density) and by the local magnetic environment. Individual molecules actually have their own magnetism. For example, protons within fat respond differently to the same magnetic pulses than protons within muscle tissue. Thus, the return to equilibrium, reflected in the T1 and T2 time constants, varies for different types of tissue. Spatial encoding is achieved by varying the magnetic field in a linear fashion through the application of magnetic gradients. Imagine five tops in a row, each one turned slightly harder than its neighbor to the left. Each top would spin at a slightly different speed. The “gradient” slightly alters the magnetic field along the area being studied. The difference in the speed of the tops can be measured and used to define a point in space. The turning of gradients on and off is responsible for the banging noise experienced during a scan. A pulse sequence is the manner in which these gradients and RF pulses are applied to the area of concern. TR refers to repetition time—the time until the next pulse of additional energy is added. TE refers to the time to echo—the echo is the released energy used to form the image. Certain pulse sequences accentuate certain tissues’ signals compared with others (e.g., fat versus water). T1- (short TR, short TE) and T2- (long TR, long TE) weighted sequences form the foundation for most clinical MRI. Gradient-echo (GRE) pulse sequences are often used in spinal imaging. GRE is highly sensitive to heterogeneity in a magnetic field, accentuating soft tissue-bone interfaces (e.g., disc-osteophyte interface) and hemorrhage. Faster pulse sequences such as fast spin-echo (FSE) T2-weighted imaging reduce scan time and limit motion artifact. These gains must be balanced by poorer fat suppression, which may obscure bone marrow abnormalities, and by diminished magnetic susceptibility effects, reducing the conspicuity of hemorrhage. Despite these limitations, FSE T2-weighted imaging has become a popular sequence for spinal imaging. The addition of a frequency-selective, fat-suppression pulse results in T2-weighted sequences in which osseous and soft tissue lesions, such as edema and tumor, appear bright with respect to marrow and soft tissue fat.8 By virtue of its heavy T2-weighting, FSE often gives pleasing images with myelographic effect; however, intrinsic spinal cord lesions may not be visualized as well.9 Shorttime inversion recovery (STIR) sequences are common in clinical practice and may aid evaluation of spinal cord parenchyma.10 STIR can be thought of as a T2-weighted sequence with fat suppression resulting from the addition of an initial inversion pulse. STIR is excellent for evaluating marrow and soft tissue edema, tumor, and spinal cord lesions. The major disadvantage of STIR is lengthy scan times, even at low resolution. Combining the advantages of STIR with the speed of FSE produces the FSE inversion recovery (FSEIR) sequence. This technique is as effective as STIR for detecting vertebral metastasis.11 Contrast agents such as gadolinium-diethylenetriamine pentaacetic acid (Gd-DTPA) also may be used for spinal imaging, particularly to evaluate lesions within the epidural space and spinal cord. These agents change the local magnetic environment, altering the signal released from hydrogen protons. Care must be used when imaging lesions primarily involving bone. On noncontrast T1-weighted sequences, the contrast between the low-intensity signal from a lesion and the high-intensity signal from marrow fat typically is good. Contrast administration can decrease the conspicuity of enhancing vertebral lesions by increasing signal from a lesion to match that from marrow fat. Adding fat saturation to a postcontrast sequence can mitigate this effect. This is referred to as “pseudonormalization” (Fig. 5-2). Diffusion-weighted imaging (DWI) uses differences in the microscopic motion of water (self-diffusion or brownian motion) between normal and pathologic tissues to generate contrast. The more movement, the lower the signal generated from a volume of tissue. DWI successfully images acute cerebral infarction and has been applied to various other central nervous system (CNS) diseases such as multiple sclerosis (MS) and cerebral abscesses. Recently, the technique has been applied to the spine to distinguish pathologic from benign vertebral compression, and results have been promising.12–14 However, at present, the application of DWI to the spine is limited. FIGURE 5-2 Pre- (A) and postcontrast (B) T1-weighted MRIs of the thoracic spine show a metastasis (arrow) that becomes less visible (pseudonormalization) after contrast is administered. Contrast is seldom indicated for evaluation of the bony spine. Open MRI often uses magnetic field strengths below 0.5 T. There are several advantages. Capital costs are lower than conventional MRI systems. Patients accept open MRI more readily and experience less claustrophobia. Finally, it accommodates larger patients. The disadvantages relate primarily to the relationship of its signal-to-noise ratio (SNR) to the strength of the magnetic field. The SNR increases in proportion to field strength. For a low-field system to achieve an SNR comparable to that of a high-field system, imaging time must be prolonged, thereby increasing the potential for motion artifact. The diminished SNR is particularly evident with FSE T2-weighted sequences and may adversely affect the detection of spinal cord lesions. The quality of T1-weighted sequences is adequate; however, enhancement with gadolinium is proportionate to field strength and is thus diminished. A higher dose of contrast is required to obtain a comparable contrast effect. The axis of the open magnet is often oriented vertically rather than horizontally. This configuration unfavorably affects the geometry of the RF coil, reducing the quality of thoracic and lumbar images. We recommend that spine studies be performed on high-field systems, particularly if spinal cord pathology is in question. Skeletal scintigraphy has been a mainstay of bone imaging for many years. Typically using a polyphosphate such as methylene diphosphonate (MDP) labeled with technetium-99m (Tc-99m), scintigraphy is a sensitive, albeit nonspecific, means of detecting bone lesions such as primary and metastatic tumors, infections, and fractures. Whole-body scintigraphy is possible for screening and represents an advantage compared with MRI and CT; however, areas of abnormality must be correlated to more specific imaging modalities. Anatomic characterization in the spine is improved greatly with single photon emission computed tomography (SPECT), which can also detect lesions not apparent on planar images.15 Uptake of Tc-99m-MDP depends on blood flow and osteoblastic activity. Therefore, highly aggressive lesions (osteoclastic activity far outstrips osteoblastic activity) or those with predominantly medullary rather than cortical bone involvement can be associated with false-negative studies (e.g., metastatic neuroblastomas and multiple myelomas). MRI is more sensitive than scintigraphy for detecting disease within the vertebral medullary space.16,17 Whole-body, fast MRI techniques that may replace scintigraphy for the evaluation of metastatic bone tumor evaluation are being developed.18–20 Scintigraphy with gallium 67 is useful for imaging spinal tumors and inflammation whereas indium 111 or Tc-99m-labeled white blood cells are useful for imaging acute to subacute infection. Tumor-specific agents include thallium 201, Tc-99m sestamibi (MIBI),21,22 and ra-dioiodinated metaiodobenzylguanidine (MIBG); the latter is used to evaluate neural crest tumors such as neuroblastomas.23 Positron emission tomography (PET) uses a positron emitter such as 18F labeled to radiopharmaceuticals that mimic endogenous molecules such as deoxyglucose (fluorodeoxyglucose, FDG). Malignant tumors have increased glucose metabolism. This feature is exploited to detect lesions and to stage and monitor tumor recurrences.24 PET bone scans using an agent such as 18F-la-beled NaF may detect bone metastasis more accurately than conventional bone scintigraphy. The clinical role of PET in the evaluation of primary musculoskeletal tumor and metastatic bone disease, however, awaits further clarification.22 The role of angiography is limited in the evaluation of spinal tumors. Hypervascular lesions such as atypical vertebral hemangiomas, giant cell tumors (GCTs), and some metastases may be evaluated and embolized before surgery. Spinal vascular malformations cannot be excluded with noninvasive imaging modalities; angiography is required. Sonography is used to screen neonates and infants for congenital lesions of the spinal canal. Tethered cord, thickening of the filum terminale, spinal lipoma, and diastematomyelia are but a few conditions that are well visualized before the neural arch ossifies.25 Intraoperative sonography also plays a key role in the resection of intramedullary spinal cord tumors. Intraoperative sonography can help localize tumors, characterize cysts, place the myelotomy, and monitor tumor resection.26 Traditionally, spinal tumors are categorized by compartment based on two important anatomic demarcations: the spinal cord and the dura mater. Lesions outside the dura mater involve the extradural compartment. Lesions within the dura mater are separated into intradural extramedullary and intramedullary lesions. Lesions of the cauda equina are below the spinal cord and considered intradural extramedullary. This categorization is somewhat artificial in that lesions such as dumbbell schwannomas can involve two spaces simultaneously. Identical pathology also can involve all three spaces. Nonetheless, this model can help formulate a differential diagnosis (Table 5-1) and describe the anatomic relationships of a lesion when combined with imaging features (Figs. 5-3 to 5-5).
Radiologic Imaging of Tumors of the Spine, Spinal Cord, and Peripheral Nerves
Imaging Techniques
Radiography
Computed Tomography
Myelography
Magnetic Resonance Imaging
Scintigraphy
Angiography
Sonography
Compartmental Approach
Extradural | Intradural extramedullary | Intramedullary |
Degenerative process | Schwannoma (NF2) | MS |
Hemangioma | Neurofibroma (NF1) | Astrocytoma |
Metastasis | Meningioma | Ependymoma |
Myeloma | Metastasis | Hemangioblastoma |
Lymphoma | Arachnoid cyst | Cavernous malformation |
Chordona | Paraganglioma | |
ABC | Ependymoma | AV dural fistula |
Giant cell | Embryonal tumors | Syrinx |
Osteoid osteoma | Metastasis | |
Osteoblastoma | ||
Osteochondroma |
ABC, aneurysmal bone cyst; NF1, neurofibromatosis type 1; NF2, neurofibromatosis type 2; MS, multiple sclerosis; AV, arteriovenous.
Extradural Tumors
Tumors of the extradural compartment primarily consist of osseous vertebral lesions and metastases as well as a smaller number of nonosseous lesions involving the epidural space. Several conditions can mimic extradural tumors (Fig. 5-3; Tables 5-1 and 5-2). Most lesions in this compartment represent either a metastatic or hematopoietic malignancy. Primary bone tumor accounts for less than 5% of extradural tumors.27
Normal and Abnormal Bone Marrow
Evaluating the osseous spine requires a thorough understanding of the changes in MRI signals from normal bone marrow with age, normal variants, and pathologic marrow processes (Table 5-2). At birth, vertebral marrow is hematopoietic (red marrow) and uniformly dark on T1-weighted sequences. During the next three decades and well into adulthood, red marrow is converted to fatty or yellow marrow. The T1-weighted signal from vertebrae increases as marrow fat increases FSE T2-weighted signal to a lesser degree. Yellow marrow becomes darker on fat-suppressed T2-weighted or STIR sequences. The conversion of vertebral marrow is patchy or accentuated around the basivertebral plexus centrally or at the periphery of the vertebral body.28 A bright T1-weighted vertebral signal usually indicates a benign process, such as normal marrow conversion, hemangioma, or radiation therapy. Normal adult red marrow should not enhance after Gd administration.
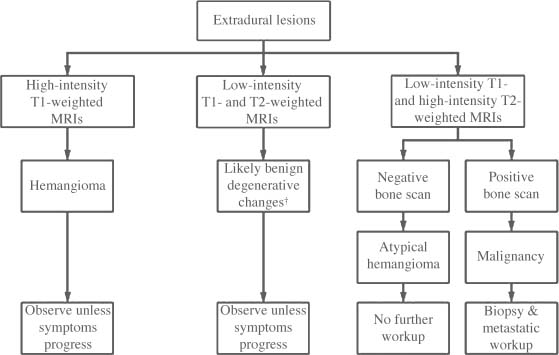
FIGURE 5-3 Clinical evaluation of extradural lesions. †A bone island is more likely than sclerotic metastasis.
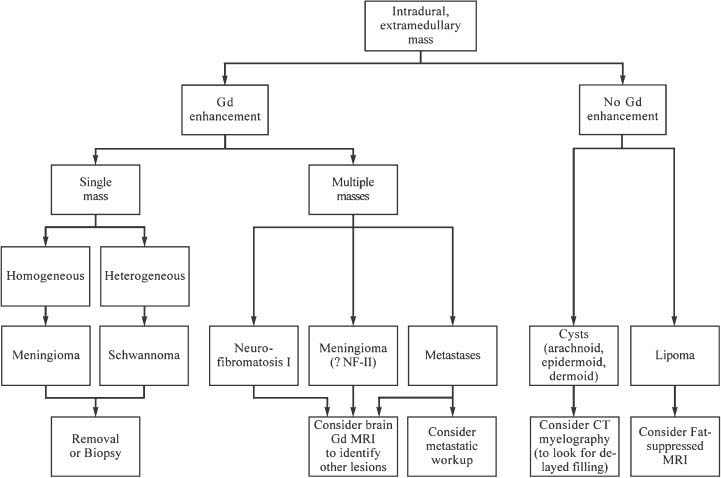
FIGURE 5-4 Clinical evaluation of intradural extramedullary lesions. GD, gadolinium; FSE, fast spin echo.
FIGURE 5-5 Clinical evaluation of intramedullary lesions. Gd, gadolinium; MS, multiple sclerosis; VHL, von Hippel-Lindau; f/u, follow-up.
Infection/inflammation Pyogenic Tuberculosis Hydatid disease Destructive spondyloarthropathy Neuropathic Dialysis Brown tumor Bone island C1–C2 pseudotumor |
When marrow proliferates, hematopoietic cells replace marrow fat (Table 5-3). Typically, these conditions are diffuse and indistinguishable from one another on imaging. Yellow marrow can convert to red as a normal variant, or the conversion can reflect increased hematopoietic demand such as from anemia, high altitude, and chronic obstructive lung disease. Most commonly, mild marrow reconversion is an incidental finding in obese adult women, typically smokers.29 Early in the proliferative process MRI may be normal. Eventually, the marrow appears heterogeneous, with patchy decreased signal intensity on T1-weighted sequences.
Proliferative Marrow Disorders | Marrow Replacement Disorders |
Benign Reconversion Myelofibrosis Mastocytosis Myelodysplastic syndrome Malignant Leukemia Multiple myeloma Amyloidosis Waldenström’s macroglobulinemia | Benign Primary bone tumors Osteomyelitis Malignant Metastases Lymphoma Primary bone tumors |
Adapted from Kaplan P, et al. Musculoskeletal MRI. Philadelphia: WB Saunders, 2001:30–37, with permission from Elsevier Science.
FIGURE 5-6 T1-weighted MRI of the thoracic spine demonstrating discs brighter than the vertebral bodies, which indicates nonspecific marrow replacement. This can be seen with tumor (for example, lymphoma) or red cell hyperplasia. This patient has myelodysplasia.
Metastatic disease can also cause marrow replacement (Table 5-3). Although typically multifocal and well defined, the changes within the marrow may be diffuse and less conspicuous. T1-weighted and STIR sequences are best for imaging these findings. A useful indicator is the “hyperintense disc sign.”30 Except for infants, discs should appear hyperintense relative to vertebral marrow on T1-weighted sequences. Marrow darker than disc on T1-weighted sequences indicates replacement of the normal fat (Fig. 5-6). Contrast is seldom needed when bony metastases are suspected. Bright lesions on precontrast T1-weighted sequences are almost always benign.
Primary Benign Extradural Tumors
Hemangiomas
Hemangiomas are the most common benign tumor of the vertebral column, occurring in 11% of spines at autopsy.31 Hemangiomas are composed of a variable vascular stroma within an osseous matrix of bone erosion interspersed with thickened vertical trabeculae. The result is a “corduroy” appearance on plain radiography and a “honeycomb” or “polka-dot” appearance on CT Multiple lesions occur in as many as one third of cases. About 60% are found in the thoracic spine. Hemangiomas are more common with senescence and are typically confined to the osseous margins of the vertebral body, posterior elements, or both. Soft tissue extension is rare.
The typical hemangioma is well defined and associated with hyperintense T1-weighted signals, reflecting adipose tissue within the stroma.32 T2-weighted hyperintensity reflects edema as well as signal from fat related to the FSE imaging technique. Atypical imaging features characterized by hypointense T1-weighted signal and intense contrast enhancement may portend an aggressive clinical course.33 Such cases represent less than 1% of vertebral hemangiomas, but symptoms from vertebral collapse or lesion extension into the epidural space can cause significant pain, radiculopathy, and myelopathy (Fig. 5-7). These lesions appear to have a more prominent angiomatous stromal content. They may be expansile and mimic vascular metastatic disease such as renal cell and thyroid carcinomas or primary vascular tumors such as hemangioendotheliomas, hemangiopericytomas, and angiosarcomas.34 Aggressive lesions should be evaluated with CT to detect the honeycomb or polka-dot pattern of thickened trabeculae. Occasionally, angiography is needed to demonstrate tumor vascularity, and the mass may also be embolized. Rare cases of nonosseous extradural and intradural capillary and cavernous hemangioma have also been described.35,36
Osteochondromas
Osteochondromas are the most common benign bone tumors; however, the spine is the site of only 1 to 4% of solitary lesions and 7 to 9% of lesions in the autosomal-dominant syndrome of hereditary multiple exostosis. The cervical spine accounts for 50% of spinal osteochondromas, with C2 most commonly affected.37 Spinal lesions are usually solitary, even in hereditary multiple exostosis, and usually arise from the posterior elements, particularly the spinous process. Lesions that project posteriorly are often large and can cause cosmetic deformity. Typically, lesions projecting into the spinal canal are small at presentation and manifest with myelopathy, radiculopathy, spinal stenosis, or cauda equina syndrome.
The radiographic hallmark of osteochondromas is a sessile or pedunculated projection in continuity with the cortical and medullary portions of the parent bone. A thin cartilaginous layer caps the apex of the lesion. Radiography is relatively insensitive for the diagnosis of spinal lesions. CT is ideal for characterizing lesions and pre-operative planning. MRI demonstrates continuity of the bright yellow marrow of the medulla and hypointense cortex of the parent bone, with a low-to-intermediate T1-weighted and hyperintense T2-weighted cartilaginous cap. MRI is also ideal for assessing the effect of lesions involving the spinal canal on the spinal cord.
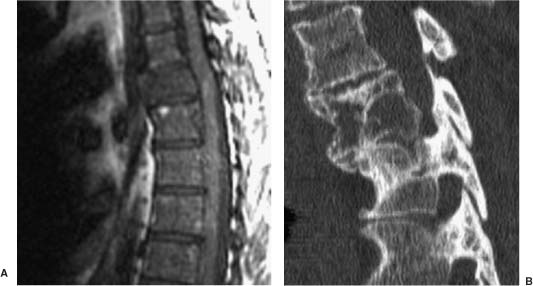
FIGURE 5-7 (A) T1-weighted MRI shows expansile hemangioma of the thoracic spine. (B) CT scan shows benign-appearing bone with striations rather than destruction. CT is often valuable for characterizing confusing bony lesions.
Lesions that grow after skeletal maturation, that are painful, or that have a cartilaginous cap more than 1.5 cm thick should be suspected of malignant transformation to chondrosarcoma. Other signs of malignant transformation include irregularity of the surface of the lesion, radiolucency within the lesion, erosion or destruction of adjacent bone, and the presence of a soft tissue mass.
Osteoid Osteomas
Osteoid osteomas account for ~6% of benign spinal tumors. Only 10% of osteoid osteomas arise in the vertebral column, more than 75% of which involve the posterior elements.38 Typically, these lesions manifest in the second decade with a 2:1 to 3:1 male-to-female predilection. The lumbar spine is most commonly affected, followed by the cervical spine. The classic symptom is pain accentuated at night and relieved by antiinflammatory agents. Scoliosis occurs in as many as 63% of patients and should raise concerns about osteoid osteomas in a child with back pain and scoliosis.
The radiographic hallmark of osteoid osteomas is a central, radiolucent vascular nidus surrounded by variable bone sclerosis. The nidus has smooth margins, measures less than 2 cm, and may calcify centrally. Radiography is often negative or shows nonspecific sclerosis of the pedicle (Fig. 5-1). Skeletal scintigraphy can reveal intense nidal uptake, sometimes with a rim of lesser uptake corresponding to the surrounding bone sclerosis—the double-density sign. Further evaluation with thin-slice CT is indicated to confirm the diagnosis. MRI may show decreased signal intensity on T1- and T2-weighted sequences in regions of marked bone sclerosis or calcification, whereas the signal intensity of T2-weighted sequences is increased in regions of marrow edema. Contrast enhancement of the nidus is intense, and an enhancing reactive soft tissue mass can mimic malignancy or infection.39
The primary differential consideration is chronic osteomyelitis with Brodie’s abscess, which has less well-defined, broader lucent margins without enhancement. Treatment of these lesions is shifting to a percutaneous approach combined with thermal ablation.40
Osteoblastomas
Osteoblastomas are related to osteoid osteomas but have distinctive clinical and radiographic features.38 Representing only 1% of all primary bone tumors, osteoblastomas nonetheless account for 10% of all osseous spinal tumors. They usually manifest in the second to third decades but have been reported in almost all ages. The associated pain tends to be duller than that associated with osteoid osteomas. Neurologic symptoms such as paraparesis or paraplegia may occur in almost one third of patients. Scoliosis complicates as many as half of the cases, particularly in males. Osteoblastomas have a predilection for the cervical and lumbar spine, followed by the thoracic and sacral levels. Typically, the posterior elements are involved. Although extension into the vertebral body is common, isolated vertebral body involvement is rare. Osteoblastomas average 3 to 4 cm, and sacral lesions are usually larger.
Radiographically, osteoblastomas are radiolucent with expansile, lobular borders and sclerotic margins. In more than half of the cases, CT shows mineralization of the nidus. In some cases, the matrix appears cartilaginous. Sclerosis of the surrounding bone varies. Many lesions exhibit aggressive, cortical destruction. Osteoblastomas show intense uptake on bone scintigraphy. MRI depicts soft tissue components, edema, and mass effect. Otherwise, the signal is intermediate to low on T1-weighted and intermediate to high on T2-weighted imaging, depending on the degree of mineralization and sclerosis.
Giant Cell Tumors
Giant cell tumors are composed of osteoclastic giant cells that arise in skeletally mature individuals. They manifest between 20 and 50 years and are most common in females.41 They account for 5 to 10% of all primary osseous tumors, ~7% of which arise in the spine. Most spinal GCTs arise in the superior or lateral aspects of the sacrum. The vertebral body is the most common site within the mobile spine. Less commonly, vertebral body lesions may extend into the posterior elements. GCTs are benign in 90 to 95% of cases but can be locally aggressive. Sacral lesions can cross the sacroiliac joint, and vertebral lesions can extend into the disc space and adjacent vertebrae. Local recurrence is common due to the difficulty of obtaining gross total resection. Histologically benign lesions can metastasize. Malignant conversion typically occurs after radiation of benign lesions. Spinal lesions become symptomatic with pain, limitation of mobility, and radiculopathy.42
The radiographic hallmarks include osteolysis and expansion without a marked sclerotic border. A significant soft tissue component is often present, and vertebral collapse can ensue. Bone scintigraphy reveals homogeneous uptake or may show a nonspecific “ donut sign” as a rim of hyperintensity surrounding central photopenia. CT shows the lack of mineralizing matrix and may reveal a thin, sclerotic border surrounding the soft tissue component, reflecting intact periosteum. Secondary aneurysmal bone may form due to hemorrhage and may be evident on CT or MRI as fluid-fluid levels. Differentiation from true aneurysmal bone cysts (ABCs) is aided by the presence of solid soft tissue elements.38 MRI reveals intermediate T1-weighted signal with hyperintense hemorrhagic regions and hypointense cystic regions. Solid regions are often hypointense on T2-weighted images, reflecting a high collagen content. This feature can help distinguish sacral GCTs from other common sacral lesions such as chordomas, metastases, and myelomas, which tend to be hyperintense on T2-weighted sequences. GCTs are usually hypervascular lesions with solid portions showing intense contrast enhancement on CT or MRI. Angiography with preoperative embolization can help decrease blood loss during surgery.
Aneurysmal Bone Cysts
Aneurysmal bone cysts are nonneoplastic processes characterized by sometimes rapid and aggressive bone expansion and accompanied by multiloculated blood-filled spaces. Their origin is attributed to previous trauma or a “ tumor-induced anomalous vascular process.”43 In as many as a third of the cases, a preexisting tumor, particularly a GCT, can be identified. Most ABCs (80%) manifest in patients younger than 20 years with a slight female predominance, and 12 to 30% involve the spine. The mobile spine is the most common and the sacrum the least common site.
Solid components (typically fibrous tissue, reactive bone, and giant cells) sometimes occur within the septa surrounding the cystic spaces. Completely solid lesions may occur and have a propensity for the spine.38 Spinal ABCs are centered in the posterior elements in 60% and in the vertebral body in 40% of the cases.
Radiographic features include expansion and remodeling of bone with a thin bone rim and internal bony septations. ABCs can extend to paravertebral spaces and other vertebral levels. Bone scintigraphy may produce peripheral uptake, the “ donut sign.” CT and especially MRI show the characteristic multilocular, cystic, and expanded mass with fluid-fluid levels. Maintaining the patient supine for at least 10 minutes before imaging is useful for sedimentation.38 High Tl-weighted signal may reflect hemorrhagic components, and high T2-weighted signal characterizes the cystic elements.
The differential diagnosis of fluid-fluid levels in the presence of a solid component includes GCTs, osteoblastomas, osteosarcomas, fibrous dysplasia, and chondroblastomas. Radiographically, ABCs with a solid component cannot be reliably differentiated from theselesions. Angiography shows peripheral hypervascularity, and embolization may be useful preoperatively or as primary therapy.
Primary Malignant Extradural Tumors
Primary malignant tumors include the common lesions derived from hematopoietic tissue within the vertebrae such as myelomas and uncommon lesions such as sarcomas. With the exception of Ewing’s sarcomas and primitive neuroectodermal tumors (PNETs), these lesions tend to occur in an older population compared with benign extradural lesions.
Chordomas
Chordomas account for 2 to 4% of primary bone tumors and occur anywhere notochordal remnants are found from the clivus through the coccyx. The pattern of spinal involvement parallels the detection of notochordal remnants in autopsy studies: 50% arise in the sacrum, 15% in the mobile spine, and the remainder in the spheno-occipital skull base.27 Most patients become symptomatic in the fourth through seventh decades of life. As opposed to the equal gender distribution of skull base lesions, spinal lesions show a male predominance of 2:1 to 3:1.
The clinical manifestations and pattern of involvement vary with spinal location. Sacral lesions tend to involve the sacrococcygeal region, sparing S1 and S2.33 Owing to slow growth and anatomic considerations, sacral lesions tend to be large at presentation. Consequently, they often cause pain, rectal compression, and less commonly, neurologic symptoms. Lesions in the mobile spine usually arise at the junction of the anterior two thirds and posterior third of the vertebral body (site of notochordal remnant). Compared with lesions elsewhere, they tend to manifest at a younger age and to be smaller. The duration of symptoms also tends to be briefer. Neurologic dysfunction from spinal cord or nerve root compression is common. Vertebral lesions are more likely to metastasize than sacral or skull base lesions. Their likelihood of local recurrence is also greater because it is difficult to achieve complete resection.
Radiologically, chordomas are midline, destructive lesions. A myxoid soft tissue component, associated with low attenuation on CT, is often present. Sacral lesions are expansile and typically have a large presacral soft tissue component with amorphous tumoral calcification. Vertebral lesions are less often associated with calcification, but vertebral collapse is common. The lesion may involve adjacent disc space and vertebral levels, mimicking infection. Involvement of the posterior elements is rare. Cervical lesions may have prominent epidural and paravertebral components associated with foraminal enlargement, mimicking a nerve sheath tumor.44 MRI is superior to CT for preoperative planning and for demonstrating soft tissue and osseous involvement, epidural tumor, spinal cord compression, and compromise of the vertebral artery. Typically, chordomas appear as low-intensity signals on T1-weighted images and as a very high intensity signal on T2-weighted sequences with heterogeneous enhancement.
Chondrosarcomas
Chondrosarcomas are the second most common nonlymphoproliferative primary spine tumor in adults; 5 to 10% of chondrosarcomas originate in the spine, most within the thoracic spine.27 Many lesions are exophytic, arising from osteochondromas. Most are low grade, manifest between 30 and 60 years of age, and are associated with a good prognosis. The male-to-female predominance is 2:1 to 3:1. Most arise from either the posterior elements or from a combination of posterior element and vertebral body. Only 15% involve the vertebral body alone. Radiographically, bone destruction is intermixed with a chondroid matrix that appears as rings and arcs. Stippled calcification is present in 70% of radiographs. Soft tissue extension is common, and about one third of lesions extend into the disc and involves an adjacent vertebral level. MRI demonstrates lobular chondroid matrix associated with low-to-intermediate T1-weighted and heterogeneous T2-weighted signal. On T2-weighted sequences, nonmineralized regions of tumor are very bright, and mineralized tumor is dark. Osteochondromas with chondroid matrix calcifications should be suspected of malignant transformation to chondrosarcoma.33
Ewing Sarcomas and Primitive Neuroectodermal Tumors
Ewing sarcomas are the most common nonlymphoproliferative primary malignant osseous tumors of the spine in children.38 About 5% of Ewing sarcomas arise in the spine, typically the sacrum, followed by the lumbar and thoracic spine. Spinal involvement usually reflects metastasis from a primary lesion elsewhere in the body. Pathologically and radiographically, PNETs are almost identical to Ewing sarcoma. Both demonstrate small round blue cells with abundant intervening collagen. Immunohistochemistry is needed to differentiate the two lesions.27
Clinical findings include pain, local tenderness, a palpable mass, and neurologic deficits. Imaging demonstrates lytic or permeative vertebral body lesions associated with focal or diffuse sclerosis. Vertebral collapse and vertebra plana can occur, mimicking Langerhans cell histiocytosis. Vertebral lesions can also mimic other tumors such as aggressive hemangiomas, lymphomas, metastases, ABCs, and GCTs.45 Paraspinous soft tissue involvement is typical. Purely extraosseous lesions can occur with epidural involvement through a neural foramen, mimicking a nerve sheath tumor.46 MRI is the ideal modality for evaluating changes in marrow and soft tissue, but the imaging features are nonspecific. Although rare, Ewing sarcomas and PNETs should be considered in the differential diagnosis of extradural spinal lesions in children and young adults.
Osteosarcomas
Primary osteosarcomas represent less than 5% of primary malignant spinal tumors.38 Secondary involvement of the spine by metastasis is much more common. Spinal osteosarcomas typically manifest in the fourth decade. They can arise 5 to 25 years after radiation therapy or, rarely, as a result of Paget’s disease
Radiographically, spinal osteosarcomas tend to be osteoblastic, creating an “ivory” vertebral body. Lytic components may be present, but rarely is the lesion purely lytic. Vertebral collapse occurs, but disc space invasion is rare. CT or MRI can delineate a soft tissue mass, and enhancement can guide a biopsy.47 On both T1-weighted and T2-weighted sequences, the signal intensity of mineralized matrix is low. Nonmineralized components are hyperintense on T2-weighted sequences.
Hematopoietic System Disease
Hematopoietic system disease includes the relatively common multiple myelomas, including plasmacytomas, as well as the less common lymphomas and leukemia.
Myeloma
Myeloma is a malignant proliferation of monoclonal plasma cells. It accounts for ~1% of all malignancies in the United States. Myeloma has several clinical forms, including multiple myeloma, solitary plasmacytoma, generalized myeloma, plasma cell leukemia, and extraskeletal myeloma. Multiple myeloma is the most common form and accounts for almost half of all vertebral column tumors.48 Its incidence increases with age (median age at diagnosis, 62 years). Multiple myeloma is rare among those younger than 30 years.49 The male-to-female distribution is 3:1.
Most patients have an asymptomatic monoclonal gammopathy of undetermined significance (MGUS) for years before multiple myeloma manifests. Most patients with MGUS do not develop multiple myelomas. However, there is no test to distinguish benign from malignant monoclonal gammopathy.50 MGUS and lytic bone lesions should suggest progression to multiple myelomas.
Imaging is directed toward detection of osteolytic lesions, quantification of disease burden, and evaluation of complications such as vertebral collapse. Radiography has been the primary diagnostic modality and is an integral part of the staging system proposed by Durie and Salmon.51 Punched-out lytic lesions are well depicted by radiography and CT. Other findings include diffuse osteopenia, fractures, and rarely, osteosclerosis.
MRI is more sensitive than radiography and may detect lesions in asymptomatic, radiographically occult MGUS.52 A heterogeneous pattern termed “ mosaic” or “variegated” has been described. On T1-weighted images focal disease appears iso- or hypointense to muscle and exhibits variable hyperintensity on T2-weighted images. Lesions mimic metastatic foci but tend to have higher T2-weighted signal and more homogeneous enhancement. Enhancement does not increase lesion detection but helps show epidural disease. Diffuse disease may appear as homogeneous decreased T1-weighted vertebral signal (lower than disc spaces) associated with diffuse enhancement and increased T2-weighted signal.
Various MRI patterns of involvement help predict prognosis. In one study, 24% of patients with advanced (stage III) multiple myeloma had normal MRIs, 44% had the focal pattern, and the remainder had diffuse disease. Patients with the diffuse pattern have a poorer prognosis than those with either a normal or focal pattern. Patients with normal MRI findings respond better to therapy.53 The variegated pattern appears to have a more indolent clinical course than diffuse disease.54 Vertebral collapse occurs more often with the diffuse pattern and with focal disease associated with more than 10 lesions.
MRI also may be useful for following therapy. As expected, clinical response correlates with decreased abnormality of the marrow, including diminished enhancement. Conversion of a diffuse pattern to a normal or focal pattern corresponds to a partial clinical response.55 Bone scintigraphy is insensitive to changes associated with multiple myeloma and should not be used for screening or following this disease.
Solitary plasmacytoma occurs in 5% of patients with plasma cell neoplasms. They occur as a single tumor containing monoclonal plasma cells identical to those in multiple myeloma. Serum or urine M-protein is seldom present. As many as 60% of lesions occur in the spine, typically in the thoracic spine. Lesions preferentially involve the vertebral body. Patients present a decade earlier than those with multiple myelomas. Solitary plasmacytoma can manifest with vertebral collapse and spinal cord compression.56 Lesions are lytic, often with thickened vertical cortical struts or bony ridges.
T2-weighted images reveal homogeneously hyperintense signal enhancement. A pattern of curvilinear hypointensity extending into the lesion has been likened to the appearance of cortical sulcation and termed the “mini brain” by Major et al.57 In their experience, this appearance of vertebral solitary plasmacytoma was pathognomonic. Lesions of atypical solitary plasmacytomas have a soap-bubble appearance that mimics hemangiomas, or they appear purely lytic, mimicking metastasis.33 If additional lesions are present on MRI, evolution to multiple myelomas should be excluded.56 Treatment for solitary plasmacytoma is radiation therapy. About half of the patients progress to overt multiple myelomas within 3 to 4 years.
Lymphomas/Leukemia
Most lymphomatous involvement of the spine is related to hematogenous metastasis from extraspinal sites. Non-Hodgkin’s lymphoma (NHL) accounts for ~85% of spinal lymphomas. NHL may be indistinguishable from other metastatic cancer, myeloma, leukemia, and other osseous tumors.58 The bone marrow is infiltrated in 25 to 40% of patients with NHL and in 5 to 15% of patients with Hodgkin’s disease.54 One to 6% of patients with NHL have no extraspinal tumor, a variant known as primary spinal epidural lymphoma.59 In these cases epidural tumor typically extends three to four vertebral segments and compresses the spinal cord. The vertebrae are involved in half the cases. There may be continuity with paraspinal disease via the intervertebral foramina. Lesions tend to exhibit uniform signal intensity and enhancement. The appearance is similar to that of metastatic carcinoma, myeloma, and tuberculosis.
The radiographic appearance of osseous lymphoma ranges from a lytic/permeative pattern to sclerosis. T1-weighted sequences reveal diffuse, heterogeneous marrow replacement, whereas T2-weighted sequences show increased signal and contrast enhancement. Occasionally, lymphomas have a putty-like appearance wrapping around cortex that appears intact. The bone may appear normal on CT, but MRI often reveals diffuse involvement of the marrow. Other small cell tumors share this appearance, but it is useful for distinguishing lymphoma from entities associated with cortical destruction such as Ewing’s and osteogenic sarcoma.54 MRI can help direct a bone marrow biopsy. Patients who undergo a negative blind biopsy whose marrow appears abnormal on MRI have a poorer prognosis than comparable patients whose marrow appears normal.60 MRI also can guide the harvest of bone marrow for transplantation to uninvolved sites.
On MRI leukemia typically exhibits a diffuse proliferative pattern in marrow that mimics reconversion. It appears hypointense on T1-weighted images and minimally increased on T2-weighted images. On STIR sequences, the signal intensity of marrow is increased compared with muscle. MRI is very sensitive for detecting leukemic changes and is useful for evaluating complications such as chloroma.54 Chloroma, or granulocytic sarcoma, is a solid tumor of granulocyte precursor cells that arise in bone marrow. It spreads to extraosseous sites and may extend into the spinal canal, compressing the spinal cord or nerve roots. Chloroma is found in ~5% of patients with acute myelogenous leukemia. STIR sequences and Gd can highlight these lesions and help differentiate chloroma from other leukemic complications such as osteomyelitis and hematoma.29 MRI also can help monitor acute lymphocytic leukemia patients for a relapse.61
Secondary Malignant Extradural Tumors
Osseous metastases are present in as many as 70% of patients with primary malignant neoplasms at autopsy. The axial skeleton accounts for almost 40% of these lesions.62 Spinal metastases are usually hematogenous and follow the distribution of bone marrow.48 Common primary tumors to metastasize to the spine include lung, breast, prostate, kidney, and lymphoma in adults and Ewing’s sarcoma and neuroblastoma in children. Most lesions are osteolytic. Osteoblastic lesions occur with prostate and occasionally breast cancer. Back pain is the most common manifestation, and spinal cord compression occurs in 5% of patients who die of metastatic disease. Paraspinal and epidural tumor involvement may occur, usually from extension of an osseous lesion. Vertebral collapse is common and a great source of morbidity in these patients. Imaging is aimed at diagnosis, staging, evaluating complications, and following treatment.
Radiography and CT of osteolytic metastasis demonstrate cortical destruction. The margins involving the vertebral body are poorly defined, and the pedicle and neural arch are often involved. Expansile lesions may be associated with renal cell and thyroid carcinoma. Sclerotic metastases have heterogeneous sclerosis with ill-defined margins. In contrast, bone islands have homogeneous sclerosis and well-defined spiculate margins. Other radiographic features of metastasis include absence of a pedicle, loss of the vertebral body posterior cortical line, and an unexplained paraspinal soft tissue mass.63
MRI is the most sensitive modality for detecting and characterizing metastatic spine disease. Typically, single or multiple hypointense lesions are identified on T1-weighted images. Except for infrequent sclerotic metastasis, most lesions are hyperintense on T2-weighted images. Fat suppression is useful for FSE T2-weighted and postcontrast images. Gd is useful if epidural extension is suspected. Epidural extension often appears bilobed because the posterior longitudinal ligament adheres to the dorsal vertebral body at the midline. Hemangiomas, Paget’s disease, and bone islands can mimic metastasis on MRI; CT is useful in such cases.
Vertebral collapse often presents a diagnostic dilemma. Collapse is typically secondary to benign osteoporosis; however, chemotherapy, steroids, and radiation therapy can accentuate osteoporosis. About a third of patients with known malignancy have vertebral collapse related to benign osteoporosis.64 Imaging, particularly MRI, attempts to distinguish benign from malignant vertebral collapse. With time a benign vertebral collapse demonstrates a fatty marrow pattern. An acute or subacute fracture is more difficult to differentiate. The signal intensity of fatty marrow may decrease due to edema (osteoporotic fractures) or cellular replacement. Marrow features favoring benign vertebral collapse include abnormal signal intensity limited to the vertebral body without pedicle extension, and a horizontal bandlike edema pattern reflecting a fracture line.65 A hypointense, horizontal fracture line extending more than half the anteroposterior dimension of the vertebral body is sensitive and specific for benign vertebral collapse. Benign fractures typically have an angulated posterior body in contrast to the bulging posterior wall associated with metastatic collapse. The absence of paravertebral or epidural soft tissue favors benign vertebral collapse. The presence of other vertebral lesions favors malignant vertebral collapse, although benign vertebral collapse can be multiple. Contrast within the vertebral body is nonspecific. Using the preceding criteria, MRI is 79 to 94% accurate in distinguishing benign from malignant vertebral collapse.66 Indeterminant lesions may be biopsied or reevaluated in 6 to 8 weeks. Although technically difficult, (DWI) MRI of the spine shows promise in distinguishing benign from malignant vertebral collapse.12–14 On DWI, malignant cells restrict diffusion, resulting in a hyperintense signal. Acute osteoporotic vertebral collapse is associated with increased extracellular water (edema) and therefore with a lower signal on DWI. DWI also may be useful for following the response of a metastatic vertebral tumor to therapy.67
Neuroblastomas
In children the most common tumors to metastasize to the extradural spine are neuroblastomas and their less aggressive variant, ganglioneuroblastomas. These tumors derive from neuroblasts, primordial neural crest cells that form the sympathetic nervous system.68 The median age at diagnosis is 22 months, and 95% manifest by 10 years of age. Neuroblastomas and ganglioneuroblastomas arise anywhere in sympathetic tissue. More than 90% arise in the adrenal medulla, extraadrenal retroperitoneum, or posterior mediastinum. They spread contiguously or metastasize hematogenously to the vertebral column. CT demonstrates calcification in 80 to 90%. The involved neural foramina may widen. MRI is best suited for evaluating the typically dumbbell-shaped epidural spread (Fig. 5-8). Once in the spinal canal, tumor can spread within the epidural space to multiple levels. Neuroblastomas and ganglioneuroblastomas tend to be hypointense on T1-weighted and hyperintense on T2-weighted images. Larger lesions may exhibit cystic necrosis and hemorrhage. Prognosis is best determined by age and stage. Younger patients usually have a better prognosis than older patients.
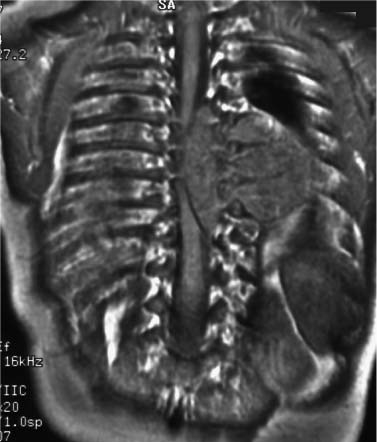
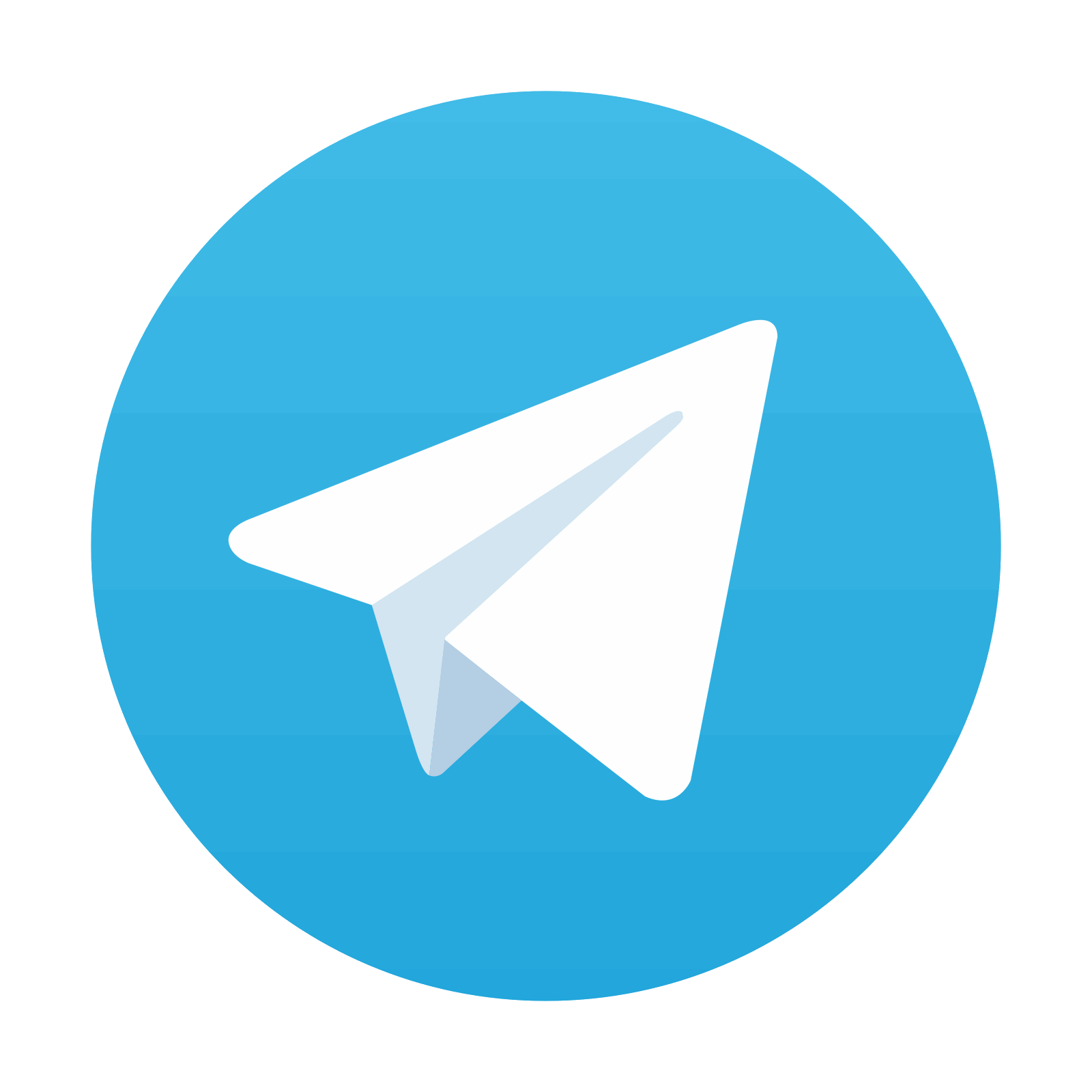
Stay updated, free articles. Join our Telegram channel

Full access? Get Clinical Tree
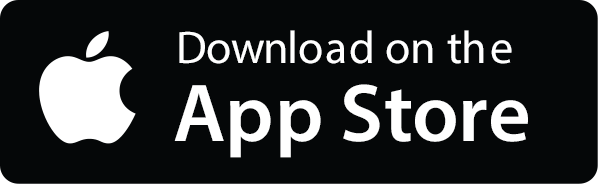
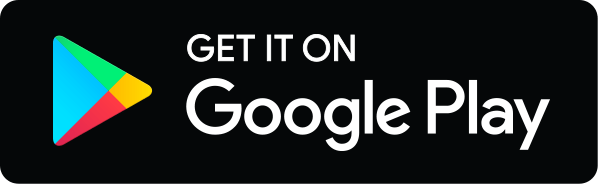