The clinical assessment of muscle tone is an integral part of the neurologic evaluation of patients with movement disorders. “Rigidity” and “spasticity” are terms used to describe different patterns of increased resistance to passive joint movements (1). Rigidity implies pathology of the extrapyramidal system, particularly the basal ganglia and frontal lobes. Spasticity implies pathology of the upper motoneuron system or corticospinal tracts (2). Increased resistance to passive movement can also arise from dystonia, peripheral nervous system disorders, and muscle contraction due to failure to relax. Nonneural causes, such as joint stiffness or ankylosis, tendon shortening, and altered passive properties of muscle—for example, muscle shortening due to fibrosis with contracture—also may contribute to a clinical impression of increased muscle tone.
RIGIDITY
Rigidity is a continuous and uniform increase in muscle tone, felt as a smooth resistance throughout the range of passive movement (3). It is usually present in all directions of movement—for example, during both flexion and extension of the wrist. The relative uniformity of rigidity in all directions is referred to as “lead pipe rigidity.” Where tremor is superimposed on a background increase in tone, a ratchet-like quality of resistance to limb manipulation called “cogwheel rigidity” is felt (1). This is commonly seen in Parkinson’s disease (PD), when the cogwheeling has a frequency of 5 to 8 Hz, corresponding to the frequency of postural tremor (4). However, it is not diagnostic and may be encountered in any condition with rigidity and tremor. Incomplete relaxation and background voluntary contraction in patients with a postural or action tremor may also produce cogwheeling.
Rigidity is commonly assessed in the upper limbs at the wrist and elbow, in the neck and trunk, and at the ankle. The patient is instructed to relax and not resist the passive movements. It is often useful to repeat this instruction during the course of the examination. In the upper limb, most examiners apply quasi-sinusoidal movements of around 0.5 to 2 cycles per second (5,6). Assessment of rigidity at the neck should be performed with the patient supine. Rigidity of the trunk and proximal muscles can be detected by assessing the ease of rotating the shoulders from side to side with the patient standing, and at the same time, rigidity of the shoulders is assessed by the freedom with which the upper limbs swing during trunk rotation. Rigidity in the legs is often difficult to assess because of the weight of the lower limb. Wartenberg’s pendulum test may be useful if there is doubt. With the patient seated on the examination table, the relaxed lower legs are raised to the horizontal in the sagittal plane and then released simultaneously. On the more affected side, the leg can be observed to drop at a slowed but uniform rate when compared with the opposite side (7).
Rigidity can be attenuated with drowsiness or relaxation so that it may be absent or inconsistent at rest, even in advanced PD (8), but it is readily reinforced or activated by proximal voluntary movement of the opposite limb (Froment’s maneuver). In basal ganglia (extrapyramidal) disease, rigidity is often more pronounced in proximal and axial muscles. Extensor rigidity of neck muscles is a striking feature of progressive supranuclear palsy (PSP) (PD), and neck rigidity with anterocollis suggests the diagnosis of multiple system atrophy.
SPASTICITY
The increase in muscle tone in mild-to-moderate spasticity is velocity-dependent (9). Hypertonia may be detected only following the application of fast muscle stretches to the affected limb, but it is not detected during slow movements. This characteristic should be sought specifically in the examination. Spasticity is commonly assessed at the elbows, knees, and ankles. At the elbow, fast flexion or extension movements may elicit a sudden increase in tone, whereas resistance to slow, passive movements is normal. There may be a supinator catch if the forearm is suddenly rotated. For the lower limb, the thigh of the extended leg is lifted quickly while the patient is supine, observing whether the heel is lifted from the bed, suggesting increased tone at the knee. Increased resistance to sinusoidal movements at the knee and ankle may be present, although this is often difficult to assess at the knee because of the weight of the lower leg. Wartenberg’s pendulum test may demonstrate that the legs drop in a series of excursions with a catch between each movement, and they rotate about an axis instead of falling in a purely sagittal plane. A critical feature of the hypertonia of spasticity is the clasp-knife phenomenon (10,11), which is best seen in the lower limbs. As the knee is flexed from an extended posture, the resistance to passive movement is initially marked but then suddenly gives way. The clasp-knife phenomenon has a directional preponderance, owing to the tendency of spasticity to affect the extensors more than the flexors in the lower limbs, and the flexors more than the extensors in the upper limbs.
The basic clinical characteristics of hypertonia following lesions of the descending motor pathways at various sites from the cerebral hemispheres to spinal cord are similar, with the exception of flexor spasms, which are a prominent feature of spinal lesions. Spasticity is usually accompanied by other signs that together comprise the upper motoneuron syndrome. Perhaps the most important of these is an increase in the tendon jerks, a phenomenon recognized by Charcot (12). Reflex threshold is lowered, and irradiation of tendon reflexes throughout a limb or to the contralateral lower limb (as in the crossed adductor reflex) is caused by spread of the vibratory stimulus, transmitted through muscle and bone, recruiting distant reflexes. Other features include altered cutaneous reflexes with Babinski’s sign (an extensor plantar response), loss of superficial abdominal reflexes, spontaneous and stimulus-induced flexor spasms, clonus, and paresis. Clonus refers to the repetitive movements of a limb elicited by rapid muscle stretch following a tendon tap or during manipulation of the limb caused by reverberating brisk spinal stretch reflexes. Paresis is usually in a “pyramidal” pattern affecting extensors in the upper limbs and flexors in the lower limbs. Even in the absence of weakness, there may be loss of the capacity to perform fractionated movements of the digits, evident as a slowness or clumsiness of sequential touching of the fingertips with the thumb, or rapid movements of the distal limb, such as foot tapping.
Spasticity typically evolves in the days and weeks after an upper motoneuron injury. During the interval before the appearance of spasticity, muscle tone is often flaccid with depression of tendon reflexes. The duration of this interval varies according to the level of the lesion. For example, increased tone in the affected limbs may appear a few days after a capsular stroke, while flaccidity may persist after a spinal cord lesion for weeks or even months. The changes responsible for the generation of spasticity are poorly understood. Once spasticity is established, the chronically shortened muscle may develop physical changes, such as shortening and contracture that further contribute to muscle stiffness (13).
DIFFERENTIATING RIGIDITY FROM SPASTICITY
Although from the preceding discussion it may appear that differentiating rigidity from spasticity is a straightforward matter, the precise differences in the pathophysiology of hypertonia in the two conditions are poorly understood. The examination of tone is rarely undertaken without knowledge of the history, and this often influences the way in which muscle tone is evaluated in an individual. There are a number of other factors that potentially confound the distinction between rigidity and spasticity. For example, rigidity or spasticity may occur in lesions of the frontal lobes or degenerative diseases such as Steele–Richardson–Olszewski syndrome (PSP) (14) or multiple system atrophy (15), all of which may affect basal ganglia and frontal (extrapyramidal) connections as well as the pyramidal system.
To further complicate the issue, dystonia may be associated with hypertonia. Dystonia was first used by Oppenheim (16) to describe a motor disorder characterized by variable hyper- and hypotonia. Although dystonia is often present only on action in mild or early cases, it can be associated with a sustained abnormality of resting posture with increased resistance to passive movement in advanced or secondary cases. Dystonia can be a prominent feature of juvenile or early-onset PD, and advanced PD, especially as the effects of medication wear off (end-of-dose dystonia) (17). Separation of the rigid and dystonic components of hypertonia in parkinsonism is as much by convention as by science. There are no objective studies that separate the two, and thus, the boundary between hypertonia in rigidity and dystonia is perhaps even more blurred than that between rigidity and spasticity.
OTHER CAUSES OF HYPERTONIA
Diseases of the frontal lobes and their connections may also produce another, particular form of hypertonia. Frontal lobe hypertonia is characterized by increasing opposition and resistance to movement with increasing force of movement, called “gegenhalten or paratonia.” The examiner suspects the patient is not fully relaxed, and contrary to instructions to relax, manipulation of the limb leads to a progressive increase in tone. If gegenhalten is marked and there are associated frontal lobe release signs, such as grasp reflexes and mutism, or striatal signs, the distinction between hypertonia of frontal lobe origin and that associated with basal ganglia disease is straightforward. However, if the associated physical signs are minor, this distinction can be difficult. This raises the question of the mechanisms of hypertonia in different diseases whose primary pathologic change lies in the basal ganglia or the frontal lobes or both, as in corticobasal degeneration. It is not known how the mechanisms responsible for hypertonia in each of these situations differ, and in view of the rich interconnections between frontal lobe motor areas and the basal ganglia, there may be some similarities.
Hypertonia also may be seen in patients with disease of the spinal cord in which unrestrained anterior horn cell discharge leads to α-rigidity. Isolation of the anterior horn cells from normal interneuron inhibitory control is the presumed mechanism. Other signs of spinal cord disease are invariably present. Continuous motor unit activity leading to stiffness of axial muscles, particularly the thoracolumbar paraspinal muscles and muscles of the anterior abdominal wall, presents a striking picture in the stiff-person syndrome. A robust feature that aids identification of this syndrome is board-like stiffness of the anterior abdominal wall, an exaggerated lumbar lordosis, the result of continuous abdominal and paraspinal muscle contraction and stimulus-sensitive muscle spasms. Continuous motor unit and muscle fiber discharges due to peripheral nerve hyperexcitability in Isaacs’ syndrome or acquired neuromyotonia cause muscle stiffness mimicking hypertonia. Clues to this include widespread fasciculations, myokymia, absent tendon reflexes, and, in some cases, other signs of a peripheral neuropathy. Finally, primary muscle disease can occasionally limit the range of limb motion, which suggests hypertonia. Myotonia may give rise to the complaint of stiffness during voluntary movement, usually due to delayed muscle relaxation, which may mimic hypertonia, though resistance to passive movement is normal. Rare congenital myopathies or muscular dystrophies with associated muscle contracture or hypertrophy also may limit the range of passive limb movement and be interpreted as hypertonia.
ANATOMICAL CORRELATES OF RIGIDITY AND SPASTICITY
Basal ganglia structures are clearly implicated in the pathophysiology of rigidity, since rigidity is a cardinal feature of nigrostriatal dopamine deficiency in PD. Moreover, rigidity is abolished following surgical lesions of the posteroventral globus pallidus, subthalamic nucleus, or ventrolateral thalamus in patients with PD. However, the changes in the basal ganglia are secondary rather than causal, since the structures named are structurally intact in PD and there are no reports of isolated rigidity following basal ganglia lesions. Bilateral lesions of the lentiform nucleus can cause parkinsonism, but this is rare and dystonia is more common (18). In contrast, unilateral lesions of the substantia nigra can cause typical parkinsonism with rigidity on the contralateral side. Rigidity with minimal other features of parkinsonism can develop in patients with a dopaminergic deficit due to exposure to neuroleptic medication or a metabolic defect in the production of dopamine. Therefore, loss of dopaminergic modulation of basal ganglia function appears to be the underlying basis of rigidity rather than loss of specific neural pathways (see Physiologic Correlates of Rigidity). Rigidity with abnormal posturing of the arm and wrist is increasingly recognized as early or presenting features of cortical neurodegenerations (for example, tauopathies such as corticobasal degeneration) affecting frontal motor areas and the basal ganglia. However, there is no precise clinicopathologic proof that involvement of the premotor cortex is responsible for the rigidity (since the disease process is inevitably widespread by the time of postmortem examination) (personal observations).
Lesions of descending motor pathways at any level from the cerebral hemispheres to the spinal cord can result in spasticity and the upper motoneuron syndrome. However, there is surprisingly little certainty as to which neural pathways are responsible for generating spasticity. It is worth noting Hughlings Jackson’s dictum that following a lesion of the nervous system, the negative features, or loss of function, inform us of the function of the structures that have been destroyed, whereas the positive abnormalities reflect the function of the structures that remain.
There are two striking and, at first, seemingly contradictory observations about spasticity that arise from lesion analysis:
1. Spasticity does not develop following lesions restricted to the primary motor cortex or lesions of the corticospinal (pyramidal) tracts at the level of the medulla, but it does develop with combined lesions of the primary and premotor cortex or lesions of the corticospinal projections above the level of the medulla. In subhuman primates, selective damage to area 4, the origin of a significant portion of the pyramidal tract, results in a contralateral flaccid paralysis, greatest in distal and least in proximal muscle groups (19). With the passage of time, strength is recovered, though it does not return to normal, and fractionated movements of the digits remain significantly impaired. Increased muscle tone is not a prominent feature. Cortical lesions that involve the premotor cortex, area 6 anterior to area 4, result in impaired postural control of the contralateral limbs. Spasticity and paresis develop with combined lesions of areas 4 and 6. Whether these observations can be applied to humans has been the subject of debate over many years. The effect of selective lesions has rarely been reported in humans. Hemisphere lesions involving the corona radiata or internal capsule producing hemiplegia probably interrupt projections from both area 4 and area 6, since descending motor pathways lie in close proximity at these sites. Flaccid paresis with loss of skilled and dexterous finger movements has been described after pure lesions of the medullary pyramid (20) or selective lesions of the spinal cord (21), apparently without major changes in muscle tone.
2. Spasticity develops below the level of a spinal cord lesion despite not occurring with corticospinal (pyramidal) tracts at the level of the medulla. These findings can be reconciled as follows: it is not loss of the corticomotoneuronal projection to the spinal cord per se that leads to the development of spasticity, but instead, loss of or imbalance in additional spinal projections from brain stem structures such as reticulospinal or vestibulospinal pathways.
The flexed-limb posture after an upper motoneuron lesion is thought to reflect increased tone in flexor muscle groups and weakness of extensor muscles, although the latter has been questioned (22). This pattern of muscle activity does not appear to depend on reflexes driven by afferent feedback from sensory receptors in the affected limb, since dorsal root section has no effect on limb posture in the monkey (23). Voluntary mass movements of hemiplegic limbs and reflex mass movements—for example, yawning—indicate that descending inputs from brain stem motor centers gain access to spinal motoneurons in upper motoneuron lesions. The origin of these inputs is not known. Residual corticospinal fibers and vestibulospinal (24) and reticulospinal (25) pathways are all capable of influencing the posture and degree of spasticity of hemiplegic limbs.
PHYSIOLOGIC CORRELATES OF RIGIDITY
There have been many studies of reflex activity in patients with rigidity. Many differ from the clinical assessment of rigidity in one or both of two crucial factors. First, rigidity is elicited by the application of relatively large, amplitude muscle stretches. Second, rigidity is assessed with the patient adopting a specific motor set, that of attempted relaxation of the limb or joint that is being manipulated. The relevance to the clinical phenomenon of rigidity of any abnormal reflex activity that is elicited under differing conditions must be questioned. With this caveat in mind, the physiology of rigidity will be reviewed.
THE REFLEX ORIGIN OF PARKINSONIAN RIGIDITY
Rigidity can be abolished by dorsal root section (26) and by the intramuscular injection of nonparalytic doses of local anesthetic (27). These studies provide strong evidence that rigidity is of reflex origin, with the afferent limb originating in muscle.
TONIC STRETCH REFLEX ACTIVITY
During passive movements of the wrist or elbow, patients with parkinsonian rigidity develop muscle bursting at the frequency of the perturbation rather than rigidity being due to unmodulated tonic muscle contraction (5,28). The timing of this bursting varies from subject to subject, but it is primarily the stretch-related activity that is responsible for rigidity (29). Enhanced tonic stretch reflex activity has also been noted following linear stretches (30,31).
PHASIC STRETCH REFLEXES
The monosynaptic stretch reflex is not responsible for parkinsonian rigidity. Charcot (12) recognized that the tendon jerks are normal in PD. He wrote of the “essential distinction between rigidity and pyramidal or spinal hypertonicity—the absence of reflex accentuation in rigidity” (12). This dictum has subsequently been confirmed with quantitative studies (32,33). H reflexes also have generally been reported as being normal in PD.
Lee and Tatton (34,35) were the first to report enhanced long-latency stretch reflexes in parkinsonian patients and suggested this abnormality might correlate with rigidity. Although these studies did not control for background levels of muscle contraction, Tatton et al. (36) later showed that heightened reflex responsiveness could not be attributed merely to inadequate relaxation or increased supraspinal drive to β-motoneurons. However, the constancy of the relationship between long-latency stretch reflexes and rigidity has subsequently been questioned (37–40).
One factor that rarely has been taken into account when interpreting studies of long-latency stretch reflexes in PD is that most recent studies have controlled for background EMG activity by asking subjects to maintain a low-level tonic contraction. As noted, rigidity is examined clinically by passive movement with the subject attempting to relax the limb. In normal subjects, long-latency stretch reflexes are modulated by alterations in motor set (41,42), and such modulation can be abnormal in PD (43). Therefore, the lack of a consistent correlation between long-latency stretch reflex amplitude and rigidity may be due merely to a failure to measure the two under comparable conditions.
SEGMENTAL AND SUPRASPINAL INFLUENCES
The tonic vibration reflex (TVR) is qualitatively normal in PD (44), although when the torque generated by this reflex is compared with controls, it is higher in patients and correlates with the degree of rigidity (33
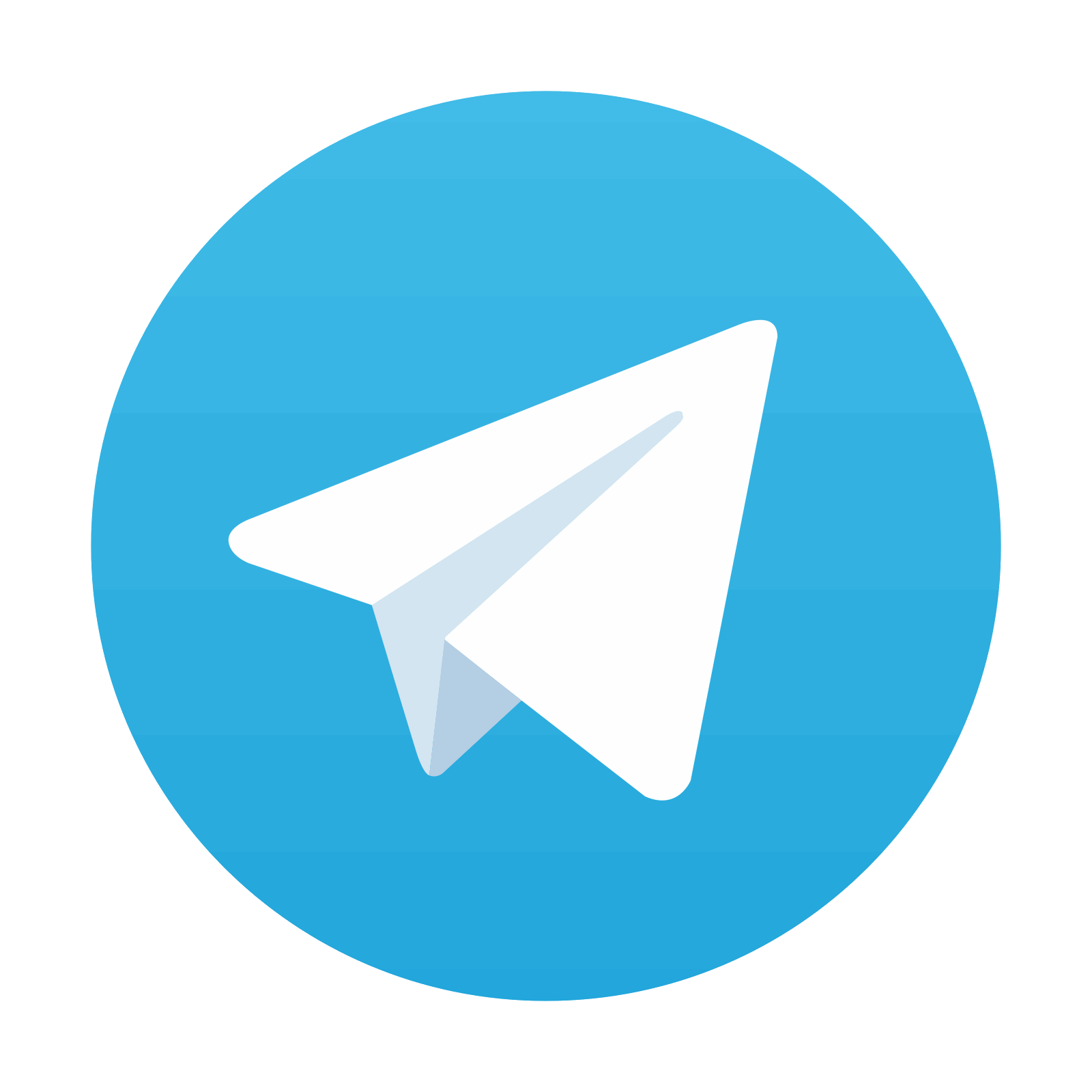
Stay updated, free articles. Join our Telegram channel

Full access? Get Clinical Tree
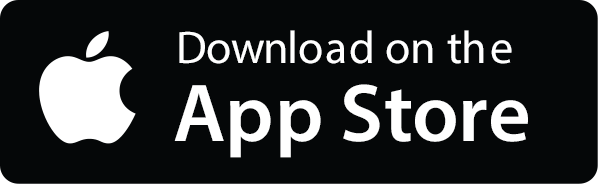
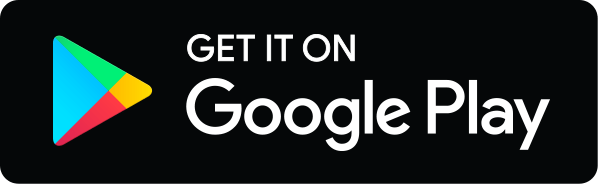