Innovations in endoscopic technique and equipment continue to broaden the range of applications in spine surgery; however, these procedures have yet to gain widespread adoption due to the challenges of mastering endoscopic technique. Difficulties remain in achieving dexterity and precision of instrument control within the confines of a limited operating space, further compounded by the need to operate from a video image. The application of robotic technology has the potential of contributing significantly to the advancement of endoscopic spine surgery. History of Minimally Invasive Surgery The introduction of computer guidance and robotics into surgical procedures has been driven by the desire to make operations both less invasive and more precise. Minimally invasive surgical (MIS) techniques have become increasingly widespread in multiple surgical disciplines; specifically, the fields of general surgery, gynecology, orthopedics, thoracic surgery, and urology have all seen the adoption of minimally invasive techniques in the treatment of a variety of surgical conditions. The goal of MIS is to reduce a patient’s pain and recovery time by decreasing the trauma of large incisions required by conventional open surgery. Additional benefits include improved cosmesis, reduced convalescence and hospital costs, and less time away from productive work.1 The basic arrangement of an MIS procedure involves the introduction of cannulas into the abdominal or thoracic cavity through small incisions generally no larger than 1 cm. A video endoscope for imaging and an array of endoscopic operative instruments are then introduced through these cannulas. Although the adoption of minimally invasive techniques has occurred primarily since the 1990s, we can trace their origin to the earliest eras of Western medicine. As early as 400 BC, Hippocrates detailed the use of a speculum for examining the anorectum. Although endoscopy can trace its roots to the ancient Greeks, the first significant procedures were not performed until the past century. In 1901, the German surgeon George Kelling used a cystoscope to examine the abdomen of a dog after air insufflation.2 Several years later, Hans Christian Jacobaeus of Stockholm, Sweden, first reported a clinically significant laparoscopy procedure in which he used a simple laparoscope with a light source at the end, with air insufflation through the scope.2 Dr. Jacobaeus coined the term laparothorakoskopie. Over the next century, the techniques of laparoscopic surgery began to slowly mature. In 1920, Zollikofer of Switzerland advocated the use of carbon dioxide gas for insufflation instead of filtered air or nitrogen.2 In 1929, Kalk described several diagnostic and therapeutic laparoscopic procedures and advocated the use of a second puncture site to establish pneumoperitoneum. In addition, Janos Veress of Hungary and O. Goetz of Germany developed needles capable of establishing pneumoperitoneum.2 Today, the Veress needle continues to be used widely in laparoscopic techniques. The Modern Era of Minimally Invasive Surgery Following the pioneering advances of the early twentieth century, the last few decades have been marked by the progressive acceptance and adoption of minimally invasive techniques to multiple surgical disciplines. By the 1970s, gynecologists worldwide began to embrace laparoscopy for diagnostic and simple therapeutic uses. The adoption of laparoscopic techniques in the field of general surgery followed a decade later. The first laparoscopic appendectomy was performed by Semm in 1983, and the first laparoscopic cholecystectomy was performed by Philip Mouret of France in 1987.2 Since then, minimally invasive techniques have been applied to almost all surgical fields. With many recognized benefits, including smaller incisions, reduced postoperative pain, rapid recovery, and shorter hospital stay, such techniques have now become the standard of care for a variety of operations. Limitations of MIS Although MIS techniques have revolutionized many surgical procedures, the introduction of MIS has also brought with it certain unique complexities that are not present with conventional open surgery. Movement Limitations First, MIS instruments work through cannulas, or ports, in the body wall. These ports act as pivot points that consequently reverse the direction of motion of the instrument tip in relation to the motion of the instrument handle. For instance, to move the tip to the left inside the body cavity requires the surgeon to move his or her hands to the right outside the body, and so on. This reversal of motion creates nonintuitive control of the instruments that is mentally taxing, especially as the complexity of the surgical task increases. Second, the majority of MIS instruments consist of an end effector mounted to the tip of a long, rigid shaft. The endoscopic cannula allows these instruments to pivot around the fixed point within the body wall, but it restricts motion laterally. The six degrees of freedom of position and orientation (defined as motion along the x, y, and z axes and rotation about each of these axes) of open instruments is therefore reduced to 4 degrees of motion (i.e., pitch, yaw, roll, and insertion) for MIS procedures (Fig. 35–1). An additional two degrees of freedom could be restored to MIS instruments by constructing articulations at the distal end, past the location of the cannula pivot point (Fig. 35–2); however, the precise and dynamic control of these distal articulations would be difficult to coordinate without the assistance of computer control. FIGURE 35–1 Traditional 4-degrees-of-freedom endoscopic instrument. Haptic Limitations The long shafts of MIS instruments force a separation of the surgeon’s hands from the operative anatomy, which significantly decreases the amount of tactile sensation and force reflection available. The extended length of the instruments also significantly magnifies any existing hand tremor. Furthermore, the excursion of an instrument tip is highly dependent on its depth of insertion. For instance, an instrument that is shallowly inserted requires comparatively large hand movements to accomplish a given instrument movement inside the body; a deeply inserted instrument requires much less hand movement to sweep the instrument tip around. As a result, the dynamics of the instrument change constantly as it is inserted and retracted throughout a procedure. Overall, all these factors can lead to less precise and less predictable movements when compared with standard, open surgical instruments. FIGURE 35–2 Fully articulated 6-degrees-of-freedom robotic instrument. The introduction of an endoscope forces a surgeon to be visually guided by a video image instead of direct vision. The video monitor is often located on the far side of the patient, and the differences in orientation between the endoscope, instruments, and monitor require the surgeon to perform a difficult mental transformation between the visual and motor coordinate frame.3 This problem is further exacerbated whenever an angled endoscope is used. The majority of conventional endoscopes are built around a single lens train that is only capable of displaying images in a flat two-dimensional format. This removes much of the depth cues of normal binocular vision, complicating such tasks as dissection between tissue planes. Some stereoscopic vision systems exist, but their performance is limited in resolution and contrast due to the endoscope itself as well as the display technology. In addition to these limitations, conventional endoscopes often require a dedicated assistant to hold and manipulate them. The natural tremors and movements of the handling assistant are exacerbated by the magnified image. Robotics in Surgery The first reference to the word robot is thought to have appeared in the play Rossum’s Universal Robots by the Czechoslovakian playwright Karel Capek, which debuted in London in 1921. The word robot was derived from the Czech word robota, which refers to a serf or a person whose role is to perform forced labor.4 Since then, the term robot has been used to refer to a variety of electromechanical devices designed to perform specific tasks. The Robotics Industries Association has more recently defined an industrial robot as a “reprogrammable, multifunctional manipulator designed to move material, parts, tools, or other specialized devices through various programmed motions for the performance of a variety of tasks.” In contrast, Webster’s dictionary defines a robot as “a machine that looks like a human being and performs various complex acts (as walking or talking) of a human being.”5 For several decades robots have served in numerous and varied applications (e.g., manufacturing, deep-sea exploration, detonation of munitions, military surveillance, and entertainment). In contrast, the use of robotic technology in surgery is still a relatively young field. Surgical procedures have advanced rapidly since the 1980s because of the large technology base that has been developed in robotics research. Improvements in mechanical design, kinematics, and control algorithms that were originally created for industrial robots are directly applicable to many surgical applications.
35
Robotic Endoscopic Spine Surgery
Type of System | Definition | Example | ||
---|---|---|---|---|
Autonomous | System carries out treatment without immediate input from the surgeon | Cyberknife ROBODOC | ||
Surgical assist Teleoperators | Surgeon and robot share control | Aesop | ||
Input from the surgeon directs movement of instruments | Intuitive Surgical da Vinci system Computer Motion Zeus system |
The first recorded application of robotics in a surgical procedure was for computed tomography (CT)-guided stereotactic brain biopsy in 1987.6 Since then, technological advances have led to the development of several different robotic systems. These systems vary significantly in complexity and function.
Classification of Robotic Surgery Systems
Robots can interact with surgeons in many ways. One classification of robots is in terms of the level of autonomy exercised by the robot (Table 35–1). One class operates autonomously in that the robots carry out a preoperative plan without any immediate control from the surgeon. The tasks performed are typically focused or repetitive, but they require a degree of precision not attainable by human hands. The second class of robots operates as surgical assist devices, meaning that the surgeon and robot share control. In the final class of robots, every function is explicitly controlled by the surgeon. The hand motions of the surgeon at the control console are tracked by the electronic controller, then relayed to the slave robot in such a manner that the instrument tips perfectly mirror every movement of the surgeon. Because the control console is physically separated from the slave robot, these systems are referred to as teleoperators.
Autonomous Systems
ROBODOC
Orthopedic surgery was one of the first areas of surgery to adopt the use of robotics. In comparison with soft tissue, bones deform minimally during cutting and are more amenable to image-guided technology. The result is that robotic procedures can result in far better agreement with a preoperative plan than the analogous manual procedure.
The ROBODOC system began development in 19867 to address potential human errors in performing cementless total hip replacement. In this procedure, the hip joint is disarticulated, and the femoral head and acetabular cup are resected. The acetabular cup is replaced by a metal and polymer prosthetic. The femoral implant consists of a long metal shaft that is inserted into a deep cavity that must be milled along the longitudinal axis of the femur. These femoral implants were originally cemented in place; however, long-term data showed that the cement was prone to cracking, loosening, or causing osteolysis that would ultimately lead to failure of the implant. Newer cementless implants have a porous metal surface and rely on natural bone ingrowth for fixation. This ingrowth requires close proximity between the implant and the bone surface, so long-term success is highly dependent on a tight fit between the two.
The ROBODOC system provides two advantages over the manual procedure. First, several clinical trials have confirmed that the robotically milled femoral pockets are more accurately formed.7,8 Second, the preoperative CT images that are used to plan the bone-milling procedure allow the surgeon to optimize the implant size and placement for each patient.
Cyberknife
Another excellent example of an autonomous robotic system is the Cyberknife(Accuracy, Inc., Sunnyvale, CA). The system was first developed in 1994 as a noninvasive means to align treatment beams precisely for frameless stereotactic radiosurgery of brain tumors.9 There are three fundamental differences from conventional frame-based radiosurgery.10 First, the treatment site is referenced to such internal radiographic features as skeletal anatomy or implanted fiducials rather than a stereotactic frame. Second, it uses real-time radiography to establish the position of the lesion during treatment, then dynamically brings the treatment beam into alignment with the observed position of the treatment site. Third, treatment beams may be aimed independently without a fixed isocenter, and changes in patient position are compensated for by adaptive beam pointing.
In practice, treatment begins with a contrast CT scan of the region of interest for preoperative planning and also for reference in the targeting process. Magnetic resonance imaging (MRI) scans can further be obtained and fused with the CT images to provide a composite study. Radiotherapy treatment plans are then formulated by a team of neurosurgeons, radiation physicists, and radiation oncologists.
The delivery of an image-guided radiosurgery treatment follows a “step-and-shoot” sequence. Once the patient is placed in a position approximating the preoperative CT scan, the imaging system acquires a pair of alignment radiographs to determine the initial treatment site in the robot coordinate system. The robotic arm then moves the x-ray linear accelerator through a series of predetermined positions, or nodes, surrounding the patient. At each node, the robotic arm stops and reacquires a new pair of images from which the target position is redetermined. After the position of the target is confirmed, the arm adapts beam pointing to compensate for any movement, and the linear accelerator delivers the preplanned radiation dose for that direction. The complete process is repeated for each node. In the typical case, the system will deliver 6 to 30 Gy at the tumor margin, distributed among 100 intersecting beams. Remeasurement intervals between delivery doses are between 20 and 40 seconds.9
The Cyberknife system is completely autonomous in that once the treatment plan is formulated, it carries out its travel path from beginning to end without human input. Corrections to beam point are calculated entirely “on the fly” based on its imbedded computer algorithm. Although an attendant physician is always present to supervise the treatment session, the computer algorithm rarely requires intervention.
Since 1994, the system has been used to treat more than 1000 patients with benign and malignant intracranial tumors11 as well as 16 patients with spinal cord lesions.10 Studies using a dosimetric phantom have shown the system to be highly accurate in its targeting, with an observed root mean squared radial error of 1.8 mm, comparable to a typical stereotactic frame-based radiosurgical system.9,12
Surgical Assist Devices
The second classification of surgical robotic systems currently in use is the surgical assist device. One example is a robotic system for bone cutting in knee joint replacement procedures.13 The surgeon grasps the cutting tool at the end of a low-impedance robot manipulator and moves the tool to reshape the bone to fit the prosthetic joint. The robot monitors the surgeon’s actions and permits free motion in the appropriate cutting region, but applies forces to prevent motion into regions where bone should not be removed. This allows the surgeon to supervise and control the robot, using human sensing and judgment, while it also provides active constraints that increase safety and accuracy of the cutting process.
AESOP
The most widely used example of this type of robot is AESOP (Automatic Endoscopic System for Optimal Positioning; Computer Motion, Inc., Goleta, CA) (Fig. 35–3). In essence, AESOP is a voice-activated robotic endoscope holder and manipulator. It allows a surgeon to attach a scope to a robotic arm that provides a steady image by eliminating the natural movements inherent in a live camera holder. The surgeon is then able to reposition the camera by voice commands. In addition, the surgeon has the ability to preset camera positions, enabling rapid repositioning of the image to different areas of the operative field. Today, AESOP is used in many different surgical disciplines, including general surgery, gynecological surgery, cardiothoracic surgery, and urology.14,15
FIGURE 35–3 AESOP Robotic Endoscope Holder (courtesy of Computer Motion, Goleta, CA).
To date, several studies have evaluated the effects of such robotic surgical assist devices as the AESOP system on such specific parameters as operative time and operative outcomes. Overall, these studies appear to conclude that although such camera manipulating assist systems do not significantly alter operative times, patient length of stay, or operative morbidity, they do provide the subjective sense that there is less inadvertent movement of the laparoscope.16 In addition, the studies conclude that such systems may be beneficial in that they decrease the need for an operative assistant assigned to hold the laparoscope, thereby enabling “solo” laparoscopic surgery in some cases.
Teleoperators
In the realm of true operative procedures, there currently are only two systems commercially available: the da Vinci Surgical System by Intuitive Surgical, Inc. (Sunnyvale, CA) and the Zeus system by Computer Motion (Goleta, CA).
Although these systems are popularly referred to as surgical “robots,” this is a misnomer, because the term robot implies autonomous movement. In neither da Vinci nor Zeus does the system operate without the immediate control of a surgeon. A better term may be computer-enhanced telemanipulators. For the sake of consistency with published literature, however, this chapter will continue to refer to such systems as robots.
The integration of computer technology into both the da Vinci and Zeus systems helps to resolve many of the limitations of MIS. By scanning the surgeon’s hand motions, information is relayed to the instruments to move them in the corresponding direction and orientation. Intuitive nonreversed instrument control is therefore restored, while also preserving the noninvasive nature of the MIS approach.
The presence of a computer control system allows one to filter out inherent hand tremor, thus making the motion of the instrument tips steadier than it is with the unassisted hand. In addition, the system allows for variable motion scaling from the surgeon’s hand to the instrument tips. For instance, a 3:1 scale factor maps 3 cm of movement of the surgeon’s hand into 1 cm of motion at the instrument tip. In combination with image magnification from the video endoscope, motion scaling makes delicate motions easier and more precise.17
In both systems, the instruments are also engineered with articulations at the “wrist” distally that increases their dexterity compared with simpler MIS tools. The da Vinci system alone possesses instruments capable of the full 6 degrees of freedom of the human wrist.
The da Vinci System
The da Vinc system is made up of two major components18 (Figs. 35–4, 35–5, 35–6, 35–7). The first is the surgeon’s console, which houses the visual display system, the surgeon’s control handles, the user interface buttons, and the electronic controller. The second component is the patient side cart, which consists of two arms that control the operative instruments, and a third arm, which controls the video endoscope.
FIGURE 35–4 The Intuitive Surgical da Vinci robotic surgical system (courtesy of Intuitive Surgical, Sunnyvale, CA).
FIGURE 35–5 An array of fully articulated, six-degrees-offreedom robotic endoscopic instruments (courtesy of Intuitive Surgical, Sunnyvale, CA).
The operative surgeon is seated at the surgeon’s console, which can be located up to 10 m away from the operating table. Within the console are located the surgeon’s control handles, or masters, which act as high-resolution input devices that read the position, orientation, and grip commands from the surgeon’s fingertips. They also act as haptic displays that transmit forces and torques back to the surgeon’s hand in response to various measured and synthetic force cues. This control system also allows for computer enhancement, enabling motion scaling and tremor reduction.
FIGURE 35–6 At the surgeon’s console, alignment of the visual axis to the master controls creates the illusion that the surgeon’s hands are operating virtually within the patient (courtesy of Intuitive Surgical, Sunnyvale, CA).
FIGURE 35–7 Arrangement of the da Vinci system within an operating room (courtesy of Intuitive Surgical, Sunnyvale, CA).
The image of the operative site is projected to the surgeon through a high-resolution stereo display system that uses two medical-grade cathode ray tube (CRT) monitors to display a separate image to each of the surgeon’s eyes. The surgeon’s brain then fuses the two separate images into a virtual three-dimensional construct. The image plane of the stereo viewer is superimposed over the range of motion of the masters, which restores visual alignment and hand-eye coordination. In addition, because the image of the endoscopic instrument tip is overlaid on top of where the surgeon senses his or her hands, the end effect is that the surgeon feels that his or her hands are virtually inside the patient’s body.
Since its inception in 1995, the da Vinci system has received generalized clearance for surgery under European CE guidelines; in the United States it has received clearance for general, thoracic surgery, and urologic procedures such as the radical prostatectomy. In addition, the da Vinci system recently received FDA clearance for cardiac procedures involving a cardiotomy. To date, thousands of surgical procedures in multiple disciplines have been performed using the da Vinci system.19–21
The Zeus System
The Zeus system (Computer Motion, Goleta, CA) is a telemanipulator system that consists of a surgeon’s console and three robotic arms (Figs. 35–8, 35–9, 35–10). The surgeon operates from a console several feet away from the operating table. There the surgeon uses handheld manipulators to control the two robotic arms and surgical instruments, a foot pedal to activate the computer driven system, and voice commands to direct a camera controlled by an AESOP arm.22 Like the da Vinci system, the Zeus system offers tremor reduction and motion scaling.
FIGURE 35–8 The Computer Motion Zeus robotic surgical system (courtesy of Computer Motion, Goleta, CA).
The Zeus system consists of three modular, freestanding robotic arms that are attached to the operating table. This design allows the system to be oriented to many different configurations. The Zeus system also features 3.5 to 5.0 mm instruments, several of which are capable of increased articulation through the Zeus Microwrist. This joint provides the instrument with an additional degree of freedom at the wrist, giving a total of 5 degrees of freedom. The Zeus system also features the ability to accommodate a variety of visualization options (3D and 2D) and scope sizes. Although these options are available, 3D visualization does not appear to be a core feature of this system. The Zeus system is similarly compatible with a variety of instruments from several off-the-shelf manufacturers.
FIGURE 35–9 The surgeon’s console with its video display and master controls (courtesy of Computer Motion, Goleta, CA).
FIGURE 35–10 The Zeus system as arranged in an operating room (courtesy of Computer Motion, Goleta, CA).
The Zeus system has also received generalized clearance for surgery under European CE guidelines. In the United States, the Zeus system has received FDA clearance for general laparoscopy and is currently undergoing FDA trials for thoracic and cardiac procedures. To date, the Zeus system has been used to perform multiple operations in many surgical disciplines throughout the world.22–24
Application to Endoscopic Spine Surgery
Procedures such as thoracoscopic diskectomy, vertebrectomy, and interbody fusion can all be performed using existing endoscopic equipment; however, mastery of these techniques in an endoscopic environment is challenging. Compared with open surgery, few would argue with the assertion that significant sacrifices are made in terms of the maneuverability and dexterity of the instrument tips, the precision and delicacy of dissection, and the sheer ease with which procedures may be accomplished.
There are several distinct and compelling advantages associated with the use of the surgical robot, which suggests that this particular technology is capable of significantly enhancing current operative technique. Unlike conventional instrumentation, which requires manipulation in reverse, the proportional movement of the robotic device allows the instruments to follow the movement of the surgeon’s hands directly. The intuitive control of the instruments is particularly advantageous for the novice endoscopist. In addition to mimicking the surgeon’s movements in an intuitive manner, the robotic instruments offer six degrees of freedom plus grip, two more than conventional instruments. This technology permits a large range of motion and rotation that follows the natural range of articulation of the human wrist, and may be particularly helpful when working space is limited. The electronic control system is capable of filtering out hand tremors as well as motion scaling, whereby gross hand movements at the surgeon’s console may be translated to much finer movement of the instrument tips at the operative site. The 3D vision system adds a measure of safety and surgical control beyond what is available with the traditional endoscope. The 3D display improves depth perception, and the ability to magnify images by a factor of 10 allows extremely sensitive and accurate surgical manipulation. The alignment of the visual axis with the surgeon’s hands in the console further enhances hand-eye coordination to a degree uncommon in traditional endoscopic surgery.
Future Directions
Although the current robotic systems represent great strides in technology, the possibilities for innovation are virtually endless.
The use of a video image that is processed through a computer system rather than direct vision allows for the overlay of any number of images or information. For instance, vital signs and other patient data may be projected directly in front of the surgeon’s eyes while he or she is operating. A 3D image of a tumor may be directly overlaid on top of the operative field as the dissection is performed. Virtual models of heart valves, orthopedic implants, or vascular conduits may be test fitted before the costly objects are requisitioned.
Because the computer systems may be made aware of both the patient’s anatomy and the position of the operative instruments, a virtual “safety envelope” may be defined. The system can then track the surgeon’s hand movements and prevent inadvertent damage to collateral tissue.
One of the “holy grails” of robotic surgery is to endow the systems with true force reflection and haptic feedback; however, the presence of the numerous mechanical joints inherently imparts additional friction to the entire kinematic chain. It is therefore difficult to distinguish friction that originates from the robotic system and forces from living tissue. This limitation will be overcome with the development of newer computer algorithms and microsensors that can be positioned at the tips of the instruments.
The control handles of such a system as the da Vinci both sense a surgeon’s hand movements and are electronically powered and can relay force information back to the surgeon. Tissue tension can be delivered, as in conventional surgery, as can any range of biological data. For instance, the pulsations of a diminutive artery can be enhanced and magnified such that it is palpable to the surgeon at the console. Other variables that are not in the average realm of human perception (e.g., oxygen tension, temperature, and density) may also be conveyed, as demonstrated by the National Aeronautics and Space Administration Smart Probe project.25
The fact that robotic systems can track a surgeon’s hand movements brings with it the ability to record that wealth of data. Thus, every nuance of a master surgeon’s performance, as well as the visual information from the operation, may be preserved. All that information may then be replayed in its entirety for those in training. Rather than stumble through an operation step by step, a novice may be able first to mimic, then to perform, an operation as it was meant to be. This “player piano” model may be invaluable in surgical education and could change the manner in which future generations learn to operate.
Computer systems are also much more facile than the human mind at processing complex coordinate frames of reference. For example, the operative instruments can be programmed to always align with the axis of view of the endoscope. Thus, wherever the endoscope is angled, it would appear to the surgeon that he or she is positioned at the end of the endoscope. For instance, an angled endoscope inserted into the mouth and directed back toward the nasopharynx could establish a vantage point for the operative instruments such that one could seem to operate through the back of the patient’s head.
A much-popularized idea is the concept of telesurgery, whereby a surgeon can perform an operation from a distance by means of a remote interface. This concept, first conceived for military applications, would allow for the delivery of surgical care to remote or inhospitable areas. It also allows a surgeon the ability to perform operations far beyond his or her immediate geographical vicinity. The world’s first transatlantic laparoscopic cholecystectomy has been performed remotely, in which a surgeon located in New York operated on a patient in Strasbourg, France.26 This concept, however, is still severely limited by the capability of current bandwidth as well as the speed of light. A less ambitious application is telementoring, whereby an experienced specialist can observe and advise a surgical team operating in a remote location. A growing number of procedures have already been accomplished using this technology.27
The advent of minimally invasive surgery has brought with it a wealth of potential benefits for patients and the health care system; however, the inherent limitations of operating in an endoscopic setting pose significant challenges for the surgeon, and this is only magnified as procedures become more complex (e.g., those encountered in spine surgery). The incorporation of robotic and computer technology has the potential to contribute significantly to the advancement of this area. As the technology continues to be refined, its ultimate acceptance will demand that issues of cost, training, safety, efficacy, and clinical utility all be addressed. There remains much work to be done, yet also much good to be gained.
5. Webster M. Online dictionary, Merriam Webster. Available at: http://www.m-w.com/netdict.htm. Accessed June 9, 2003.
6. Young RF. Application of robotics to stereotactic neurosurgery. Neurol Res. 1987;9:123–128.
< div class='tao-gold-member'>
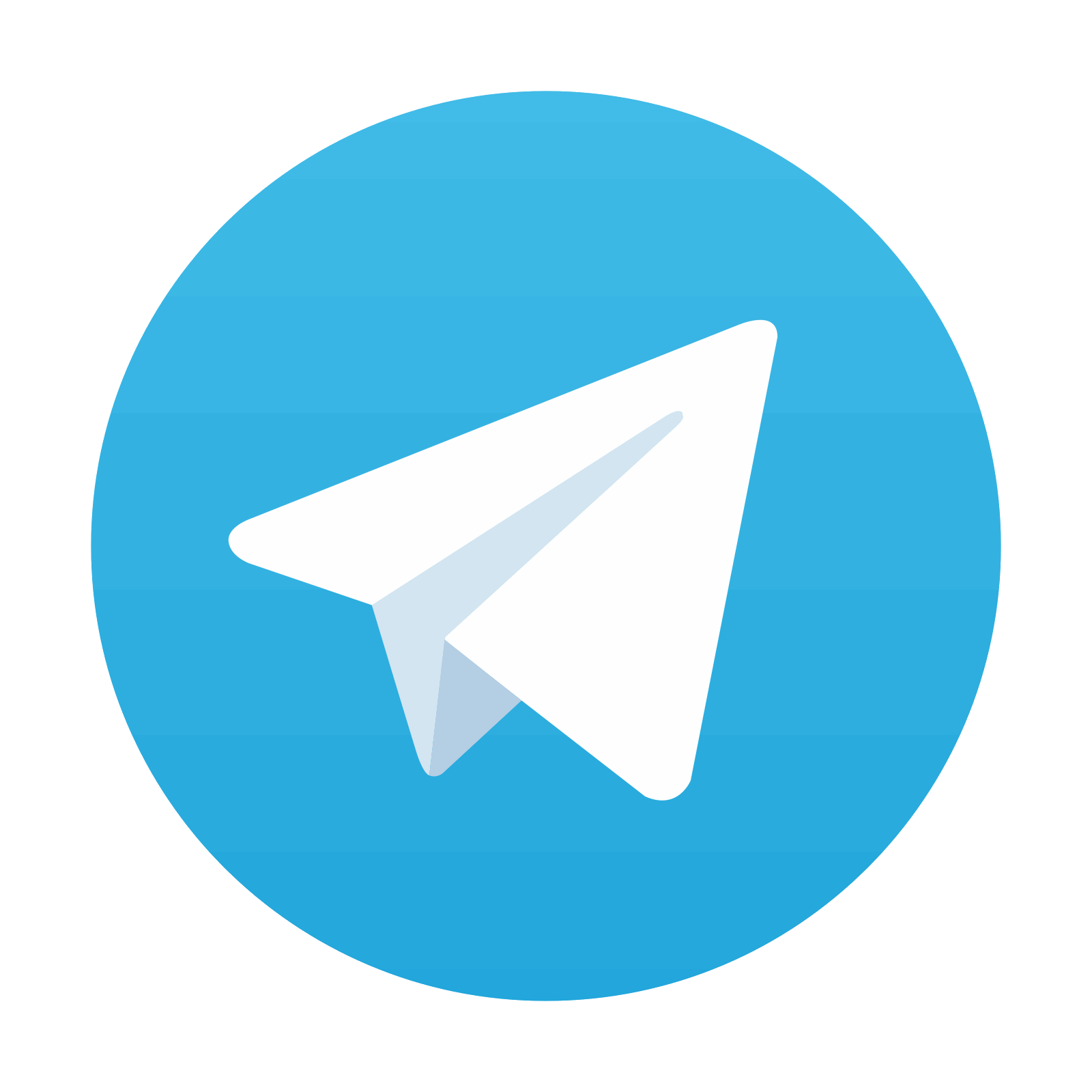