28 Spinal Cord Injury in Children P. David Adelson, Ricky Madhok, and John Y. Lee Trauma continues to significantly impact our society and the cost of health care within the United States. Overall, outcomes following severe trauma have significantly improved due to the development of tertiary trauma centers and various trauma protocols, though trauma still remains the leading cause of death and disability in children.1 Although the incidence of head trauma is markedly greater in the pediatric population, spinal cord injury (SCI) can often be more devastating due to the permanent long-lasting sequelae, associated complications, and difficulty of total care and chronic management that extend into adulthood; many children will require a lifetime of supportive care and therapy. Due to the significant amount of resources needed to acutely and chronically care for spinal cord injured patients, there is a major financial impact and cost for the ~1,100 children that are injured each year. It has been estimated that the average acute care costs for the injured child, including emergency care, intensive care, acute care, and acute rehabilitation following injury are approximately $300,000. Annual chronic care costs have been estimated to be $50,000 to #100,000 for ongoing therapy and supportive care that will extend for the life of the child.2 To improve outcome, it is important to have a better understanding of the basic mechanisms of injury, treatment, and recovery. This chapter reviews the etiology, pathophysiology, radiological findings, and management options concerning SCI as well as future potential areas of treatment intervention as they relate to children. Despite preventive programs, the annual number of new SCI in both children and adults in the United States remains ~11,000 to 12,000. The overall frequency of SCI in children aged 1 to 15 years has been estimated between <1% and 13%.3–10 In one of the better studies of incidence of trauma and SCI in children, Kraus et al3 reported the annual incidence of SCI in children to be ~10%, or 1065 injured each year. These numbers likely represent an underestimation of the true incidence. Mild cases of SCI are frequently discharged immediately from the emergency department, and severe trauma cases that may have had an associated SCI and resulted in mortality at the scene are often not included in statistics of incidence. Similarly, birth trauma and whiplash injuries are usually not included in the reported statisitics.3,4 As a result, the characterization of SCI mostly reflects those patients that survived their injury to be hospitalized and could be clearly identified as having a clear SCI. Of note is that the annual incidence of SCI in children is greater than the incidence for paralysis due to polio during the peak years of 1964 to 1972.11 Further analysis of the statistics shows that SCI, like trauma overall, tends to be more common in males than in females, with a ratio of 1 to 2.3:1. In young children it has been reported the there is more of an equal incidence of SCI among both sexes.12 As well, older children, primarily those between the ages of 10 and 15 years, more commonly suffer an SCI than other-aged children though this may be due to the more common mechanism of injury, that of motor vehicle collision. The change in gender predominance with age has been observed in multisystem trauma as well. However, ~50% of children with multisystem trauma with SCI die prior to hospitalization. Of those with multisystem trauma and SCI who survive to be admitted to the hospital, 80% survive with varying degrees of neurological deficits, the majority with complete and permanent paralysis. The vast majority of pediatric SCIs are caused by motor vehicle collisions, with the child involved as a passenger, pedestrian, or bicyclist. This mode of trauma is responsible for anywhere between 25% and 66% of the reported SCIs. Falls are also a common cause of SCI with an incidence of between 10% and 40%.5,6,9,12–15 In some reported studies, sporting and recreational injuries are a more common cause of SCI resulting in an overall incidence of 4% to 20%,9 with several different etiologies grouped together, including horseback riding, motorcycling, gunshot wounds, trampolining, all-terrain vehicles, and other activities. The peak period for SCI in children is during the summer months, with another peak during the 2-week holiday period at the end of December into the beginning of January.15 Although often not included in the statistics on SCI, birth injuries may also contribute a significant proportion of spinal cord injured children.5,6,16 Because most birth SCIs are not accompanied by radiological abnormality,17 neurological deficits must be differentiated from peripheral nerve or brachial plexus injuries. With severe birth trauma, these children will frequently suffer injuries at multiple levels of the cord and inclusive of central and peripheral nerve systems.7,18–23 In one series by Ruge et al,6 birth injuries represented 4% of all SCIs in their series. Osenbach and Manezes,5 in analyzing a younger age group, showed that 16% of SCIs resulted from birth trauma. Some have suggested that perinatal deaths may be due to SCI where up to 10% have been attributed to upper cervical spine or cervicothoracic junction SCI.24 Shulman et al18 reported that an SCI during vertex delivery was quite rare, though in another series,7 one third of the injured children were delivered either vertex or suffered their SCI secondary to a persistent transverse lie. In the same study, the other two thirds of infants with SCI were born via breech. SCIs that occur during breech delivery have been attributed to a severe traction of the trunk with an immobile head and neck25 and, at times, may be due to neck hyperextension. A difficult forceps delivery with shoulder dystocia not only can cause a brachial plexus injury but can contribute to a cervical SCI26 and may be the etiology of injury in some of these children.17 Birth trauma cannot always be cited as the cause because in ~20% to 25% of children born with an SCI, there is reportedly a normal, uneventful delivery.18 In these cases, it is hypothesized that the etiology for the resultant injury include either or both mechanical and focal ischemic injury. In children, because the vertebral column is quite elastic secondary to it being for the most part cartilaginous at birth and the lack of significant mechanical protection of the dura during delivery, the spinal cord is particularly vulnerable to rupture once the dura has been compromised. Other potential contributing mechanisms include hyperextension of the fetal head during vaginal delivery, which was cited in one series of children with SCI who were diagnosed with persistent hyperextension in utero, with 77 of the children breech in presentation and 11 in transverse lies. Of the 57 children delivered vaginally, 21% suffered an SCI; in contrast, among the 31 patients delivered via cesarean section, there were no SCIs.27 It was recommended by these authors that cesarean sections be performed for children with persistent hyperextension noted on in utero imaging. The proportion of complete versus incomplete injuries varies between different series and often depends on the level of injury. Although most series report that the cervical region is the predominant site of all SCIs,4,5,28–30 there are a few series reporting the thoracolumbar spine to be the most prevalent site.31,32 In addition, some reports have noted that more complete injuries occur with cervical-level injuries, whereas others have reported that, because they require greater forces to cause injury, thoracolumbar injury more commonly results in complete loss of neurological function. Kewalramani et al4 reported that, although 64% of injuries in their study occurred in the cervical region, the majority of patients who presented with complete injuries suffered thoracolumbar trauma. In contrast, Anderson and Schutt9 reported that two thirds of the patients with thoracolumbar SCI were incomplete. Hachen32 reported that 66% of the injuries were complete; 100% were complete when they occurred in the thoracic spine, and only 40% were complete when the injury involved the cervical or lumbar spinal regions. Others have shown that the majority of patients presenting with complete injuries suffered thoracolumbar injury rather than cervical injury.28 Also, when dealing with SCI, there are frequently multiple levels of injury in up to 16% of cases.12 One other aspect to the above discussion is that the location of SCI injury often varies based on age at injury. In the birth to 8-year age range, the cervical spine is the most commonly injured site, with upper cervical spine injuries occurring more frequently in younger children (e.g., infants and toddlers), whereas in older, school-age children and adolescents, lower cervical spine injuries are more common. In the series by Ruge et al,6 injuries to the C1-C2 region occurred in over half of those children under 3 years of age; in older children (4 to 12 years of age), levels other than C1-C2 were involved in 92% of cases. In children older than 8 years, thoracolumbar injuries tended to occur more commonly.6,12 Similarly, Osenbach and Menezes33 reported that in the birth to 8-year age range, 79% of injuries involved the cervical spine, whereas in 9- to 16-year-olds, only 54% involved the cervical spine. Despite the different findings in different series with regard to level of injury and likelihood of complete injury, age at injury is also a significant risk factor for complete injury, with younger children more often suffering a cervical injury that results in a complete injury as compared with older children. Burke7 reported that 86% of SCIs in children were complete injuries and occurred mostly in children who were younger than 13 years of age. Pang and Wilberger reported that, although only 21% of all children with an SCI presented with complete neurological deficits, complete deficits were found in 50% of children younger than 8 years of age.14 As is well known, the predilection for cervical injury and the more serious nature and proportion of injury in young children is likely due to the spinal column anatomy and biomechanics at that age. With growth and maturity, it is believed that there is better protection of the spinal cord by the surrounding structures. Different mechanisms of SCI create unique pathological and neurological deficits between adults and children and children of different ages, though the most likely significant contributing factor is the inherent differences in the spinal column between the different ages. These differences make each age group more susceptible to different injuries in comparison even from the same mechanism of injury due to the exposure of the spine to different biomechanical forces. In the infant, the head is a disproportionately large mass attached to a relatively weak neck. Forces of acceleration and deceleration, particularly in a restraint situation, can create a large movement of rotation at the fulcrum of the weak neck,34–36 resulting in high torque forces15 putting the upper cervical spine at high risk for significant trauma. It is thought that the fulcrum of cervical movement may also be located higher in younger children as compared with adolescents and adults.34 Other factors that may contribute to a more significant and the increased likelihood of a complete injury in the pediatric population include the morphology of the facets and vertebral bodies, ligamentous laxity, and uncovertebral joint maturity in children. The facets in children morphologically are more horizontally oriented, which is more pronounced in the upper vertebral bodies, making them more susceptible to vertebral/facet slippage, particularly in the upper cervical spine during forceful translation.37 In addition, the anterior vertebral bodies in children are more often wedge shaped (forward), which also predisposes them to greater mobility and less stability with hyperflexion or hyperextension movements.36,38,39 Ligamentous laxity is another important factor in the biomechanical profile of the pediatric spine. This unusually lax ligamentous system, inclusive of the joint capsules,40 contributes to the increased susceptibility to horizontal shearing due to weakness, elasticity, and deformability of the ligaments.38 This can be noted particularly at C2-C3 and C3-C4, where pseudosubluxation up to 4 mm is a normal variation in 40% to 50% of cases.37 Lastly, the relative immaturity of the upper uncovertebral joints (particularly at the levels C2-C4) also make them more susceptible to looseness and potential shearing/slippage.14 These factors individually and in combination make the child more predisposed to translational types of injuries of the cervical spine and spinal cord that can occur oftentimes without overt fractures. It is thought that by the age of 8 years, the pediatric spine has matured sufficiently to help prevent some of these more severe injuries. Hyperflexion, rotation, traction, hyperextension, vertical load, flexion rotation, and shearing are all mechanisms of injury that may cause an SCI and, depending on the force and direction as well as the combination of injuries, can result in different types of pathological findings (e.g., hyperflexion and traction can result in a tearing or shearing of the spinal cord).23 Hyperflexion or hyperextension and dislocation are the more common etiologies of injury in children because of the high elasticity of their ligaments.13 A combination of hyperextension and hyperflexion can occur, as in whiplash injuries in SCI seen following nonaccidental trauma from the child being shaken.14,41 Cervical SCIs from diving accidents are most often as a result of flexion and compression with an axial load. It has been hypothesized that forward bulging of the ligamentum flavum during forcible extension results in SCI without radiographic abnormality (SCIWORA).17 Subluxation following cervical injury occurs in approximately three quarters of the patients, with a significant proportion suffering a fracture.27 Older children and teenagers more often have fractures of the vertebral bodies and disruption of the posterior elements,5,6,9,12 whereas, as noted earlier, younger children more often have fractures that involve the cartilaginous end plate injuring the active growth zone or pure ligamentous injury.38 Hadley et al12 described four radiographic patterns for injury in children: (1) fracture involving the vertebral body or posterior elements only (~40%), (2) fracture with subluxation (33%), (3) subluxation without fracture (10%), and (4) SCIWORA (10%). In their series, younger children tended to more commonly develop subluxations without fracture or SCIWORA, whereas older children were more likely to have a fracture and fracture with subluxation. Pathologically there is a vast literature following experimental SCI. Light microscopic histological evaluation of animal models of SCI has shown that immediately following SCI there are typical patterns of injury. With minor compressive forces to the spinal cord, damage is limited to various portions of the central gray area with sparing of the surrounding white matter tracts. Hemorrhage and an inflammatory reaction, marked by the infiltration of polymorphonuclear leukocytes (PMNs), can be seen as early as 2 to 6 hours following injury, depending on the animal model, even in the mildest of injuries. This early PMN infiltration is followed by a persistent macrophage population in the region of injury up to 60 days after the trauma. Demyelination of axon fibers can be localized initially and then become widespread in the chronically injured animals; often the integrity of these demyelinated axons is preserved.42 More severe induced traumas to the spinal cord can lead to a total disruption of the central gray matter in conjunction, with an inflammatory reaction that can also occur diffusely in the white matter tracts resulting in central necrosis and cystic cavitation of the central cord.43 In addition, trans-synaptic degeneration of caudal motor neurons can be visualized on electron microscopy following spinal cord transection.44 Interestingly, in experimental models of SCI, the injured animals continue to improve despite this overwhelming secondary response, cytotoxic accumulation, and ongoing destructive changes occurring within the spinal cord throughout the subacute period.45 Following a concussive or compressive force to the spinal cord, there is an immediate death of a large percentage of the neural bodies residing in the local central gray matter. Typically, some proportion of the axons traversing the surrounding white matter survive the acute injury but are at risk for disruption due to the surrounding milieu and impact of the secondary injury.45 In the acute period following the SCI, the secondary injury cascade is initiated, profoundly influencing the ultimate functional outcome. This cascade of events includes an inflammatory response via the arachidonic acid cascade, the release of excitatory amino acids (glutamate and aspartate), and the lipid peroxidation of cell membranes by various species of oxygen-free radicals.9,46–48 Changes in local blood flow, due to tissue edema as well as to the actions of various vasoactive inflammatory mediators, may lead to further ischemic injury to the cord.49 Finally, the initiation of apoptotic changes in neurons and glial cells occurs, leading to continued cell death for days and even weeks following the SCI.50 The contribution of this pathophysiological cascade or secondary injury to the ultimate histopathological and functional outcome of the patient or animal is the subject of intense interest and debate. An understanding of these events is crucial because many future potential therapies for acute SCI represent attempts to modify or ameliorate the destructive effects of these secondary processes. The histopathological changes seen following human SCI appear to be similar to those described in the animal models,42,45,51 with the exception of the spinal column injuries and “solid cord lesion” patterns of injury. In a histopathological analysis of 12 children who had died with SCI, Auldermaur38 found a split in the cartilaginous end plate of the vertebral bodies without vertebral body fracture. This was also reported by Hachen,32 who showed that the splitting of the cartilaginous end plate was often within the growth zone of the child’s vertebral body. Although intervertebral disk herniation or extensive fractures were uncommon, several patients had an unstable ligamentous injury and capsular rupture. Because the immature spine progressively ossified throughout childhood, it was suggested that young children were more susceptible to avulsions and epiphyseal separations rather than true fractures.38 This has also been hypothesized as the etiology for odontoid fractures that commonly occur through the basal synchondrosis into the body of C2 and below the level of the superior facets in children.15 In children, type II and III injuries of the dens routinely heal with spontaneous fusion and conservative treatment with immobilization, unlike the adult where operative fusion is often necessary.15 It was also noted that death in association with SCI may be due either to a vertebral artery injury resulting in quadriplegia or to marked cervical instability and cord injury.13,32 Other associated injuries found at autopsy in children who have suffered significant spinal trauma can also include spinal, epidural, and subdural hematomas, and notable were the associated head injuries (22%) that most likely contributed to mortality.5 Towbin et al21 also showed multiple spinal cord pathological findings in children at autopsy, including spinal cord contusion, infarct, laceration, transection, dural disruption, vertebral artery injury, and epidural and subdural hematomas. Spinal cord transection or anatomical discontinuity between proximal and distal segments of the spinal cord is frequently associated with mortality and in one study, occurred in 8 of 17 patients who died with clinically complete SCI,52 whereas a compressive mass lesion was responsible for the injury in 16 of 22 patients. Laceration of the cord secondary to a gunshot wound was noted in six deaths. The majority of patients in this series suffered a contusion-type injury and developed a hematomyelic cavity with disruption of the gray matter and relative preservation of the white matter tracts. Histological examination of all the patients revealed a pattern of extensive demyelination with relative preservation of the axon fibers, especially small fibers in the dorsal columns. Other types of injuries include spinal cord necrosis secondary to hyperextension and spinal cord transection, most often occurring at the mid to lower cervical or upper thoracic region, following birth injuries. In these birth injuries, there is often cord destruction and associated vascular injury or dissection contributing to worsened injury. Another contributing mechanism for the pathology following birth injury is the application of longitudinal traction resulting in rupture of the cord.14,52 For chronic compressive lesions, there is an associated extensive demyelination, again with preservation of axon fibers.52 Disruption of the white matter tracts located at the central portion of the lateral columns with the preservation of local gray matter, as is seen in a small subgroup of these patients, has been described as a solid cord lesion. The clinical and magnetic resonance imaging (MRI) characteristics in these injuries are consistent with a central cord-type syndrome.20 MRI technology has also been quite useful to follow the longitudinal changes in spinal cord pathology, including hemorrhage and its resolution, syrinx formation, inflammation, and edema. The majority of patients with SCI will have some level of radiographic abnormalities, with over 75% suffering vertebral injury, with the neural arch involved in ~13%.28 As a result, a significant amount of information can be obtained simply from the initial plain films. The extent of many of these injuries can be further elucidated with multiplanar neuroimaging (i.e., computed tomography (CT) or MRI). CT provides excellent osteoarticular imaging and can further define the bony anatomy and alignment through three-dimensional reconstruction software. CT and CT myelogram, however, are limited in their visualization of soft tissues and soft-tissue injury. With the introduction of MRI, Betz et al53 showed that MRI was more sensitive than CT in detecting subacute and chronic injuries of the spinal cord. MRI could not easily assess acute SCI, though it may be useful in the acute setting to identify ligamentous injury, particularly posteriorly, with high-signal abnormalities on T2 imaging (Fig. 28-1). MRI is relatively insensitive in picking up acute hemorrhage within the spinal cord. T2-signal abnormalities within the spinal cord may be detected early and probably represent cord edema. It may also be helpful for screening the level of injury, spinal column alignment, and extrinsic spinal cord compression. MRI has also been useful in the evaluation of posttraumatic syrinx, spinal cord pathology, and the physiological status of the spinal cord after the acute period. Chronic evaluation of these patients using MRI in children may also reveal the finding that there is often damage to the growth plates within the vertebral column following severe spinal trauma, which may explain why many of these children failed to obtain a normal vertebral height due to asymmetric growth and occasional scoliosis. With the increased use of new technologies such as perfusion MRI, diffusion-weighted MRI, and MR spectroscopy, it is widely believed that further understanding of the physiology and pathophysiology of the injured spinal cord will be better elucidated. Figure 28-1 T2 sagittal magnetic resonance imaging (MRI) of a 12-year-old involved in a motor vehicle collision, with transient paraparesis and dysesthesias in the hands. Plain x-rays and computed tomography were unremarkable. MRI did not show any particular pathology within the spinal cord or canal. There was only subtle T2 signal change in the posterior interspinous ligaments (arrow). First described by Pang and Wilberger,14 SCIWORA was initially defined as a syndrome of traumatic myelopathy without vertebral column disruption as visualized on plain spine films, myelography, CT (with or without myelography), or flexion-extension films. It excluded injuries secondary to penetrating trauma, electrical shock, obstetric complications, and those associated with congenital anomalies. Since that initial description, the incidence of SCIWORA has been reported to occur in 5% to 70% of cases14,32,33,54 in a wide range with a mean incidence of ~40%. The frequency of its diagnosis, however, more often depends on clinical awareness and the extent of the radiographic investigation. Several reviews of SCI in children more recently have found that the true incidence of SCIWORA is likely 15% to 20%,4,54 the lower incidence of cases of SCIWORA likely representing improved imaging capability and the increased use of MRI. The majority, two thirds, of these types of injuries occur in young children, less than 8 to 10 years of age,4,12,14 less commonly in adolescents, and rarely in adults.13,14,55 SCIWORA is frequently a difficult problem to diagnose due to the lack of radiographic abnormalities. The prevalence of SCIWORA in children when compared with adults is related primarily to the biomechanics of the pediatric spine, which is more flexible, allowing for greater motion without overt damage as previously discussed. The pattern of neurological injury is closely related to the mechanism of injury as well as age. The inherent elasticity and hypermobility of the pediatric spine allow for a transient subluxation at the time of injury, with the elastic recoil returning the spine to a relatively normal alignment at the time of presentation.14,56,57 It has also been suggested that hyperextension in young children may cause bulging of the soft pediatric cervical disk into the spinal canal, resulting in narrowing and compression of the ventral spinal cord. Radiographic cadaveric studies have demonstrated that cervical disks have the potential under hyperextension to dislocate and spontaneously reduce without any later radiographic evidence. MRI has demonstrated loss of continuity along the posterior longitudinal ligament (PLL) as evidence of posterior disk herniation, intradiskal hemorrhages, and hemorrhages and edema within ligaments. As well, the infant spine is more susceptible to distraction injury, as much as 2 inches, without radiographic evidence, whereas the spinal cord may rupture after only 0.25 inch. of distraction. After the age of 8 years, many of the unique pediatric anatomical features have matured to an adult orientation, correlating with the reduced incidence of SCIWORA in older children.
Epidemiology and Demographics
Mechanism of Injury
Spinal Cord Injury due to Birth Trauma
Extent of Injury
Biomechanics and Pathology
Biomechanics
Pathology and Pathophysiology of Acute Spinal Cord Injury
Imaging Findings
Spinal Cord Injury Without Radiographic Abnormality
Frankel Grade | Function |
A | Complete paralysis |
B | Sensory function only below injury level |
C | Incomplete motor function below injury level (1or 2/5) |
D | Fair to good motor function (3 to 4/5) below injury level |
E | Normal function below injury level |
The neurological findings immediately following a SCI-WORA can be variable,14 with presentations consistent with complete, anterior cord, Brown-Séquard, central cord, or partial cord injury syndromes. The incidence of complete SCI following SCIWORA varies between series: 10% to 92%. 4,6,7,9,33,58 Younger children less than 8 years of age with SCIWORA will most often present with a Frankel grade A to C injury (Table 28-1), representing either a complete or a severe SCI,14 as compared with older children (58% to 92% vs 15% to 50%),6,12,14 especially when the site of injury is the thoracic spine (82%).6 Complete injuries are more likely to be in the cervical region in adolescents. Overall, cervical and lumbar injuries are more often associated with incomplete neurological deficits (63% and 75%, respectively), whereas lumbar injuries tend to be incomplete in all age groups of children. Younger children (e.g., infants and toddlers) with SCIWORA are more susceptible to high cervical injuries, with SCIWORA occurring frequently in the occiput -C2 region.12,38
SCIWORA may also occur in delayed fashion (up to 4 days after the injury).14 In the delayed syndrome, there is a rapid deterioration to complete neurological dysfunction that is most often irreversible. In the original description, ~50% had a delayed onset of their neurological decline,14 with some type of neurological sequelae acutely that partially or completely resolved prior to their subsequent decline. Others have reported a less frequent occurrence of this delayed injury (up to 22%), though whether this is due to improved imaging and therapeutics remains unclear.6,12,33,55 It was originally hypothesized that this delay in onset was due to progressive secondary injury14 or a vascular or ischemic type injury resulting in spinal cord infarction.59 MRI has helped to further delineate the pathological and physiological changes in this delayed neurological deterioration.53
MRI has also been helpful in predicting outcomes after SCIWORA. Complete interruption of the cord or a string appearance that can follow a distraction-type injury, or major diffuse hemorrhage involving <50% of the cord, can easily be imaged and are associated with a poor prognosis. In contrast, a minor cord hemorrhage (<50%) will initially present with moderate to severe neurological dysfunction and is often associated with partial recovery. When there is only edema present or no imaging abnormality on MRI, patients will most often have a good to excellent outcome.60–62
Management
The overall management of a child with an SCI does not differ significantly from the adult. The goal of management of neural injury, whether spinal cord or brain, is to prevent further injury and loss of neurological function by reducing the incidence of secondary insults (e.g., hypotension, hypoxia, etc.) and the resultant secondary damage as well as movement of a potentially unstable spine and further manipulation injury to the injured cord. It is of paramount importance that any initial management of SCI consists of immobilization, constant monitoring, and assessment of the entire spinal axis to prevent any further compromise of an already injured spinal cord. Simultaneous with this immobilization, the initial evaluation and resuscitation of these patients begin at the scene of the injury, with a constant reassessment and resuscitation continuing throughout the child’s hospital course. The basic tenets of the trauma treatment protocol do not deviate in SCI and must include the evaluation and intervention for the patient’s airway, breathing, and circulation. It is always important to consider the often multiple and significant systemic injuries that have also occurred following the primary injury/ trauma that may have a significant impact on the viability of the injured spinal cord. Secondary insults in particular, such as hypoperfusion, hypoxia, or hyperthermia, of the injured spinal cord may lead to worsened secondary injury with further and permanent neurological damage. It is therefore important that any management protocol include the parameters specifically delineated to maintain systemic support of oxygenation, blood pressure, and temperature, to name a few.
Early Resuscitation Management
Following SCI, particularly to the cervical spinal cord, hypoventilation and hypoxemia from the lack of neural control are of particular concern. These patients often require early intubation and assisted ventilation depending on the severity of their respiratory insufficiency with a low threshold for intubation. Assisted ventilation to maintain proper ventilation and oxygenation is necessary and should be maintained throughout the hospital course until sufficient respiratory drive and function have been restored. Although neurological complications caused by intubation are rare as long as the cervical spine is immobilized, fiberoptic intubation is preferred63,64 when feasible. In some cases, an emergent cricothyroidotomy may be required for immediate airway access, particularly when there is an upper cervical SCI or if there is severe facial trauma that does not allow for a quick, effective intubation.
Because many patients with an SCI will also have associated injuries such as a concurrent head or intra-abdominal injury, hypovolemic shock is a common postinjury sequela. Cervical and cervicothoracic cord injuries will result in loss of motor and sympathetic vasomotor tone frequently contributing to loss of cardiovascular support, systemic vasodilation, and increased venous capacity, with resultant hypotension and shock. Also, in general, when there is loss of the sympathetic input, there can be an associated bradycardia that needs to be differentiated from hemorrhagic shock. For the loss of sympathetic tone, the treatment is volume resuscitation. Volume expansion is usually adequate to treat the child in shock secondary to SCI, but this depends on the other systemic injuries as to whether it will be adequate for hemorrhagic shock. Dopamine, neosynephrine, or other inotropes should be used only when cardiac output is not corrected with volume replacement, and further cardiac support is needed. Central venous catheters are often all that is needed in children to monitor fluid status and venous return, whereas more intensive monitoring to assess pulmonary artery wedge pressure, cardiac output, and vascular resistance is reserved on an individual basis and if extensive inotropic support is necessary.
Adjunctive Management
To better evaluate fluid status in these patients, and because of the lack of normal urinary function (retention or incontinence), urinary catheters are routinely placed to continually assess urine output and prevent distention of the bladder. Although nasogastric tubes are contraindicated in patients with craniofacial injuries because of the danger of intracranial penetration,60 they should be considered to prevent or limit the aspiration of gastric contents due to loss of tone and supine position leading to potential susceptibility for emesis. Gastric pH can be monitored through the nasogastric tube and treated as necessary with adjunctive therapy, including antacids, histamine H2 receptor blockade, and/or sucralfate. Patients with gastrointestinal ulceration or treatment with high-dose steroids should be treated prophylactically with intravenous H2 blockers to diminish gastric acid secretion. Adult patients are often treated with pneumatic compression boots and low-dose heparin because pulmonary embolism (PE) occurs in approximately 5% of adults with SCI and lower-extremity paresis.65 The incidence of PE and deep venous thrombosis in children is uncommon, but it is more likely as a result of a lack of literature and studies in this population rather than a true incidence. It is therefore an option for the treating physician whether prophylactic prevention of thromboembolic treatment should be instituted in children.
Neurological Evaluation
A detailed initial neurological evaluation remains essential in delineating the level of injury, defining the spinal cord syndrome, and obtaining a baseline injury assessment for all patients with a suspected SCI. Subsequently, changes are assessed as to whether there has been improvement or deterioration after initiation of treatment intervention. A complete exam should include motor functions of each of the major muscle groups, as well as a rectal examination for anal sphincter constriction and tone. Additionally, motor function of the diaphragm needs to be assessed to ensure an adequate level of innervation for respiratory sufficiency and can be assessed based on adequate inspiratory effort, or at times is based on chest x-ray. Although the diaphragm is innervated by a high to midcervical level (C3-C5), injury below this level may be complicated by paresis or paralysis of the intercostal musculature, resulting in inadequate respiratory drive and pulmonary dysfunction with aspiration, pneumonia, or atelectasis. Sensory function is tested for all modalities (e.g., temperature, proprioception, etc.) as well as reflex functions. Sacral reflexes may be particularly important because they may indicate residual neurological function and represent a positive prognostic sign. Other associated neurological injuries that can occur with SCI include brachial plexus injuries (2% to 3%)5,15 and head injuries (10% to 25%) that may mask the resultant dysfunction from the SCI. It is important that all head-injured patients be maintained in spine precautions until they are “cleared” radiologically or clinically or both.28
A complete injury at presentation has been defined as no motor or sensory function below the level of injury. Due to many ambiguities and the mixing of definitions in the literature, an expert panel from the American Spinal Injury Association (ASIA) further refined the definition of a “complete” injury to include no motor and sensory function as well as no anal and perineal sensation or motor representing the lowest sacral cord (S4-S5). Furthermore, ASIA expanded on the Frankel scale, developing a new classification scheme for SCI using ASIA A through E, with an ASIA A defined as a “complete” injury to an ASIA E, where motor and sensory function are preserved (Table 28-2). The ASIA committee also classified incomplete SCI into five types: a central cord syndrome is associated with a greater loss of upper limb function compared with the lower limbs; Brown-Séquard syndrome results from a hemisection lesion of the spinal cord; an anterior cord syndrome occurs when the injury affects the anterior spinal tracts, including the vestibulospinal tract; conus medullaris and cauda equina syndromes occur with damage to the conus or spinal roots of the cord. This classification of SCI has led to greater clarity and uniformity in describing injuries to patients.66
ASIA Grade | Dysfunction |
ASIA A | No motor or sensory preserved below the level of the injury, a “complete” injury |
ASIA B | Sensory but no motor function preserved below the level of injury including the sacral segments |
ASIA C | Motor function in more than half of the key muscle groups below the level of injury, and more than one half of key muscle groups below the neurological level have a muscle grade less than 3 out of 5 (less than antigravity function). |
ASIA D | Motor function is preserved below the level of injury and at least half of the key muscle groups have motor strength greater than 3 (greater than antigravity function) |
ASIA E | Preserved motor and sensory functions |
Imaging
There are several studies that have established five well-defined clinical criteria following blunt trauma that, if met, would suggest a low probability of cervical spine injury and therefore would not warrant radiographic imaging: (1) no tenderness along the posterior midline of their cervical spine, (2) no focal neurological deficit, (3) a normal level of alertness, (4) no evidence of altered mental status due to intoxication or other drugs, and (5) no distracting injury. In children, because plain x-rays may not show a transient dislocation or injury due to lack of bony abnormalities, there is a low threshold for obtaining films to screen for injury. Unfortunately, the overwhelming majority of radiographs are ultimately normal.
In a multicenter prospective, observational study using the algorithm described earlier for imaging was evaluated to determine its reliability and validity. Of the 34,069 patients evaluated radiographically in 21 clinical centers, both community and university, only 818 (2.4%) had radiographically documented cervical spine injury. There were only eight patients that were later found to have a false-negative finding, and only two of these patients had what was deemed a clinically significant injury, leading upon analysis to the conclusion that there was a sensitivity of 99%, a specificity of over 99%, and a negative predictive value of 99.8% for all patients with clinically significant injury. In adult patients without tenderness along the posterior midline of their cervical spine, no neurological deficit, a normal level of consciousness with no evidence of intoxication, and no distracting injuries, clearance of the cervical spine injury can be done without radiographic examination.61 This study did not include children; therefore, although it is likely acceptable to use these criteria for older children and adolescents, young children may require a lower threshold for imaging.
Plain X-Rays
Following cardiopulmonary stabilization, a comprehensive radiological evaluation, when indicated, of the entire spinal neural axis is essential for the overall treatment and care of children following trauma. Plain spinal radiography of the cervical spine is obtained in the emergency department and should include lateral, anteroposterior (AP), and open-mouth views that visualize the C7-T1 juncture, including a “swimmer’s” view for adequacy. The comprehensive valuation includes the thoracolumbar and sacral spines with AP and lateral views to ensure there are no other fractures or subluxations at lower levels (Fig. 28-2).
Flexion/extension views of the spine are often used to determine the stability of a particular region whereas oblique views are useful if there is concern of a traumatic spondylolisthesis. Flexion/extension views are contraindicated if there is instability noted on the plain views; this is particularly true in those patients who have neurological deficits or obvious compression fractures or soft-tissue injury. Flexion/extension views can only be obtained in cooperative patients without distracting injury who are able to relate to the physician whether there is pain or change in neurological function in their extremities or body (e.g., paresthesias or motor problems). When adequate flexion or extension views cannot be obtained due to limited range of motion from spasm or “concussion” of the spinal cord leading to transient neurological deficits, immobilization for 1 to 2 weeks in a hard cervical collar followed by a repeated examination may facilitate the determination of stability. Although neurological deficits may indicate a level of injury to focus attention, plain x-rays of the thoracolumbar and sacral as well as cervical injuries often require further evaluation and assessment including MRI or CT myelography to exclude a surgical lesion.67
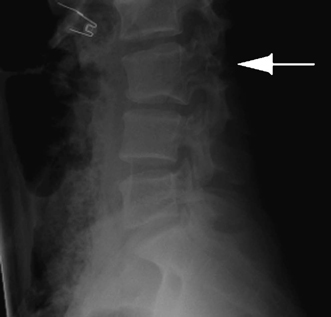
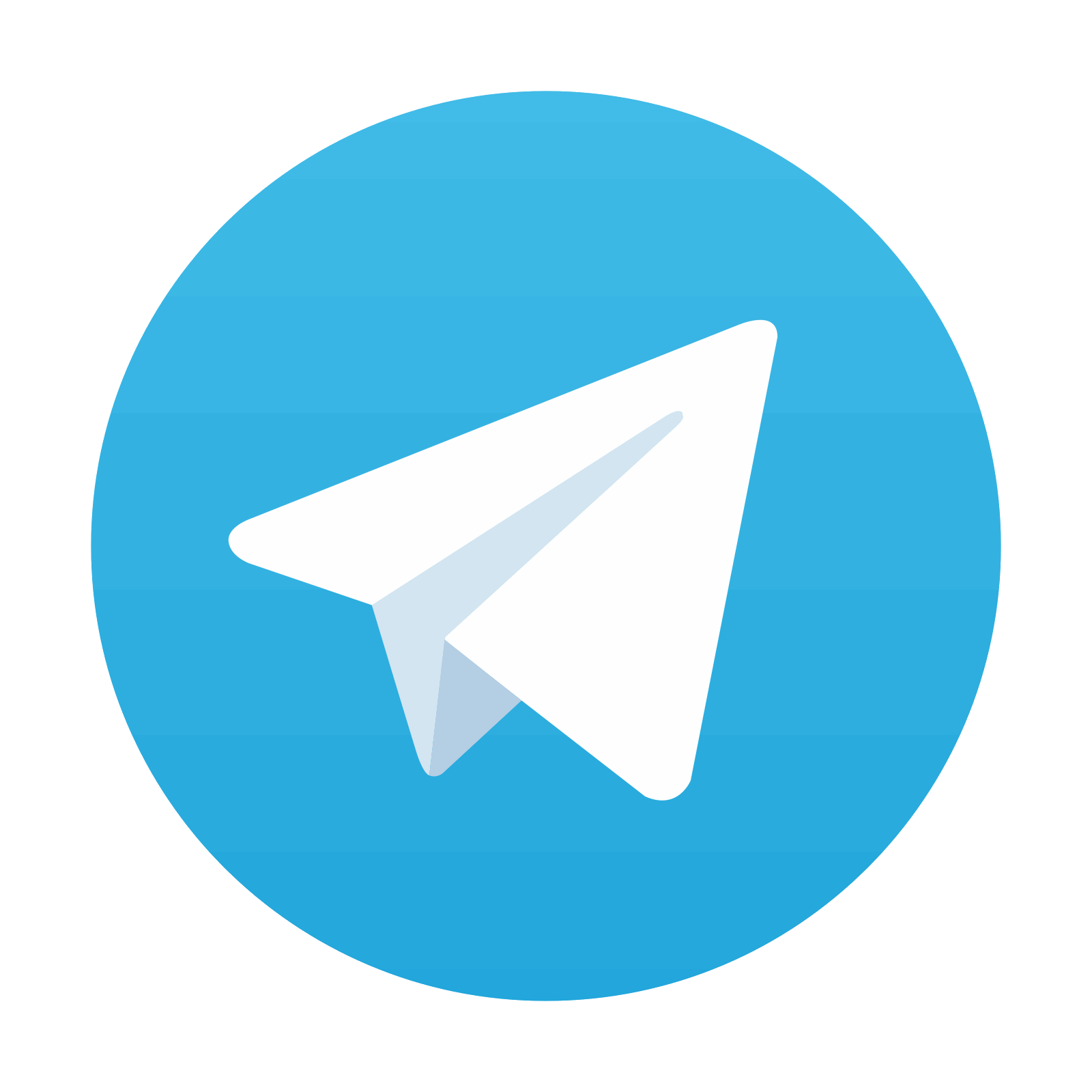