and Toru Itakura2
(1)
Department of Neurological Surgery, Wakayama Medical University Hospital, Wakayama, Japan
(2)
Department of Neurological Surgery, Koyo Hospital, Wakayama, Japan
6.1 Introduction
Deep brain stimulation (DBS) is a well-established treatment for movement disorders, such as Parkinson’s disease (PD), essential tremor, and dystonia. The best clinical effect depends on precise placement of the electrode in the motor territory of the target nucleus by stereotactic neurosurgery. Stereotactic neurosurgery was developed by the pioneers Sir Victor Horsley, Clarke, Spiegel and Wycis (Spiegel et al. 1947), and others. To ensure accuracy and safety, surgical techniques have been improved according to the accumulated clinical results. However, surgical techniques are not uniform and differ slightly between countries as well as between institutes in the same country. The results from a recent survey evaluated procedures and apparatus used in institutes performing DBS surgery (Abosch et al. 2013). Frequently used stereotactic frames include Leksell or CRW. The preferred procedures are as follows: imaging by computed tomography (CT) after head-frame fixation, combined AC(anterior commissure)–PC(posterior commissure)-based and direct visual targeting, microelectrode recording setup, and macrostimulation. Combinations of procedures also differ slightly between institutes. In the past, we used to determine tentative targets using ventriculography and CT, however, since 2001, we have been using CT-magnetic resonance imaging (MRI) fusion-guided targeting by Leksell’s frames. Here we describe our procedure with reviewing literatures (Starr 2002; Kramer et al. 2010).
6.2 Patient Selection
Patient selection is an important criterion. At our institute, neurologists and neurosurgeons discuss diagnosis, surgical indication, target, and DBS tuning. A diagnosis of Parkinson’s disease is essential for good surgical results. Medical treatments, the coexistence of visual hallucinations, and higher cortical dysfunction are discussed. To select the optimal target, the patients’ symptoms are also discussed. If unilateral tremor is main symptom, ventral intermediate(Vim) thalamic-DBS is offered, and marked drug-induced dyskinesia for internal segment of globus pallidus (GPi)-DBS, wearing off, fluctuations in Parkinson’s symptoms for subthalamic nucleus (STN)-DBS. The patient’s age and duration of disease are also important factors. If intracerebral microbleedings are apparent on T2*-weighted images (T2*WI), careful surgical interventions and microelectrode recordings are necessary (Terao et al. 2003; Gorgulho et al. 2005; Ben-haim et al. 2009). Bilateral GPi-DBS is performed in primary dystonia (Krause et al. 2004) or tardive dystonia, whereas ventrooralis thalamotomy is performed in unilateral focal dystonia. Aggressiveness of the involuntary movement is discussed because in dystonic cases or in children, general anesthesia is required for frame placement, and while verifying optimal targeting, a staged operation is required (Starr et al. 2006; Air et al. 2011; Cif et al. 2012). Past history, preoperative screening tests, laboratory data, and cardiorespiratory functions are also assessed.
6.3 Presurgical Medication
Medications are interrupted on the night before surgery, if possible. Several dopamine agonists have prolonged antiparkinsonian effects. For suitable targeting, macrostimulation effects for involuntary movements are critical, particularly in Vim thalamotomy or thalamic DBS.
6.4 Stereotactic Frame Placement
Considering the application accuracy, Leksell’s frame or CRW is widely used for stereotactic surgery (Maciunas et al. 1994). Here we describe frame placement procedures using the Leksell Stereotactic System model G (Elekta Instrument AB, Sweden). Frame fixation should be parallel to the Frankfurt line joining the inferior orbital rim and the upper limit of external auditory canal. Shaving of hair is not necessary (Miyagi et al. 2002), but hair should be tied to perceive head shape easily. Before stereotactic frame fixation, an intravenous line is obtained. Sedative drugs are not preferable because they modify intraoperative involuntary movements, consciousness, and neurological findings. However, they can be used if the patient has anxiety, intolerance during procedures, or hyperkinetic involuntary movements that disturb accurate frame placement. Blood pressure is monitored intermittently at approximately 10-min intervals. An antihypertensive agent is injected intravenously when blood pressure increases above 140/90 mmHg. Pain at the pin site or a sitting position may cause vasovagal reflex. Care should be taken for hypotension, bradykinesia, and autonomic signs such as sleepiness, yawns, or cold sweat. Syncope or convulsive syncope may sometimes occur. A supine position or rapid hydration may improve symptoms and vital signs. A patient is positioned on bed, which is elevated at 60–70°. A cushion is placed under shoulders and upper back. Ear bars are used to prevent head roll and yaw. Inserting a sponge in the external auditory meatus prevents ear pain or discomfort. A stereotactic frame is placed and fixed parallel to the Frankfurt line joining inferior orbital rim and upper limit of external auditory canal (Fig. 6.1). The frame is intended to be parallel to the AC–PC line. However, the AC–PC line varies in length and gradient among individuals; therefore, we adjust individually using preoperative images. Three people check to ensure the frame is parallel with the head from three directions. The pin tightening location is selected 6–7 cm above the Frankfurt line. Care is taken that head pins do not slip because of the forehead curve. The length of the frame pole is adjusted for individuals so that it is not too close to the shoulders. Pin lengths are adjusted according to head shape. Local anesthesia is performed using 4–5 mL of 1 % lidocaine. The skin surface, galea, and periosteum are anesthetized sufficiently. Patients feel no pain but head tightness after frame fixation. Two diagonal pins are tightened simultaneously, followed by the other pair of diagonal pins. Drivers are held by the thumb and index or middle finger, and pins are tightened using maximum power. During pin tightening, care should be taken to avoid frame rotation. Therefore, the correct framing from three directions should be repeatedly verified.
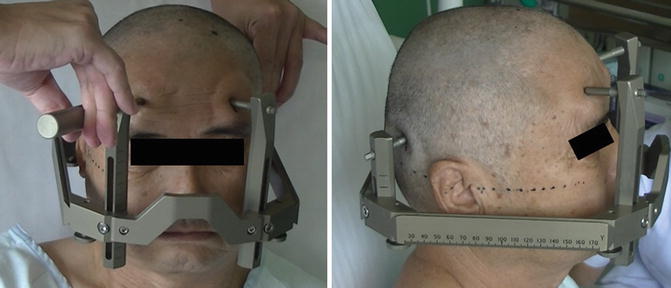
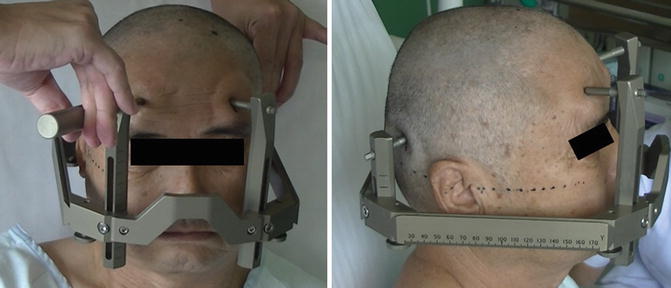
Fig. 6.1
Frame placement procedures using the Leksell Stereotactic System model G (Elekta Instrument AB, Sweden). The stereotactic frame is placed and fixed parallel to the Frankfurt line joining the inferior orbital rim and the upper limit of external auditory canal. The length of the frame pole is adjusted for individuals so that it is not too close to the shoulders. Pin lengths are adjusted according to head shape. The two diagonal pins are tightened simultaneously, followed by the other pair of diagonal pins. Drivers are held by the thumb and index or middle finger, and pins are tightened using maximum power. During pin tightening, care should be taken to avoid frame rotation. Therefore, the correct framing from three directions should be repeatedly verified
6.5 Imaging Acquisition
Historically, tentative targets had been identified using ventriculography or CT-guided targeting, but MRI-guided targeting is being used recently (Kawashima et al. 1992; Alexander et al. 1995; Kondziolka and Flickinger 1996; Starr et al. 1999). A previous report comparing ventriculography and CT-guided stereotactic neurosurgery concluded that ventriculography has no advantage over CT-guided targeting, although no cases of STN ablation were observed (Hariz and Bergenheim 1990). MRI-guided targeting has advantages of high spatial resolution, noninvasiveness, and no exposure to radiation. However, spatial distortion (Sumanaweera et al. 1994) and long acquisition times are disadvantageous. The image fusion method has resolved these problems (Alexander et al. 1995). A CT-MRI fusion method overcomes spatial distortion in MRI-guided targeting. This method is also advantageous because speedy CT scanning after fixation of stereotactic frame relieves the burden on the patient. Among several targeting methods, we describe here a CT-MRI fusion, MRI-guided targeting using Framelink (Medtronics, USA).
6.5.1 MRI Acquisition on the Preoperative Day
An MRI is performed on the preoperative day. We use a 1.5 Tesla MRI system and obtain three-dimensional T1-MPRAGE (Siemens, Germany) with gadolinium enhanced images (field of view 240, voxel size 256 × 256, slice thickness 1.5, TE 4 ms, and TR 4.7 ms). Data are imported to recordable media. T2 turbo spin-echo or inversion recovery sequences demarcate the STN or pallido-internal capsule border (Starr et al. 2002; Air et al. 2011) (Fig. 6.2). Images using 3 T MRI may offer reliable targeting for STN-DBS surgery (Toda et al. 2009; Patil et al. 2012). Segmentation of thalamic nucleus and identification of vim is tried by diffusion tensor imaging (Coenen et al. 2011). The best method for correct targeting is under debate (Shin et al. 2010; Thani et al. 2011; Pezeshkian et al. 2011). A combination of these methods may approach the optimal target (Brouenberg et al. 2011) and achieve a better clinical outcome.
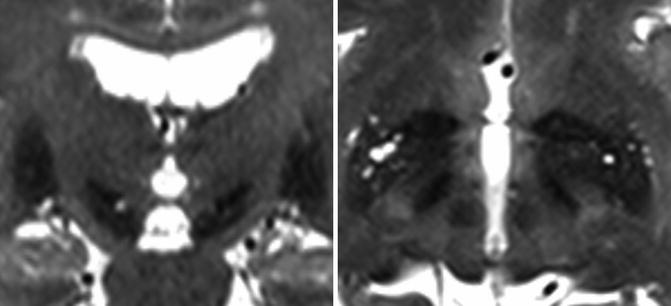
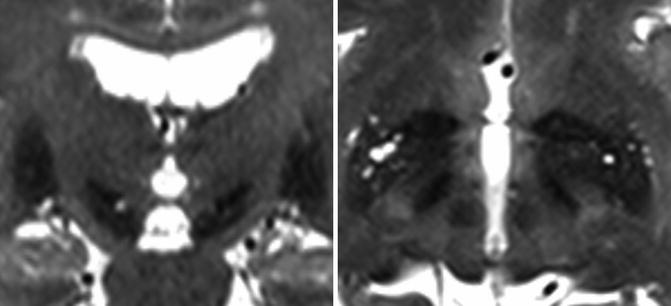
Fig. 6.2
T2 fast spin-echo images by 3T MRI demarcate the subthalamic nucleus or pallido-internal capsule border, which may offer reliable targeting
6.5.2 CT Image Acquisition on the Operative Day
On the operating day, CT images are acquired after stereotactic frame fixation. Care should be taken to avoid respiratory failure due to overflexion or extension of the neck by frame fixation during the CT scan, even during short acquisition times. After proper setting of the CT adapter, the Leksell frame is fixed to it. The frame indicator of the midline and horizontal line should match the laser beam of the CT gantry. If a gap appears, flexion, extension, and lateral tilt are adjusted using a wrench driver. We use the GE Signa CT system. Image sequences are obtained by 1.25 mm slice thickness. After verifying that the CT images show CT indicators, the data are recorded in recordable media. The patient is released from the CT adapter and transported to the operating room.
6.6 Operating Room Setting
Neurophysiological examination is important for correct targeting and for suitable local anesthesia. Patients with violent, aggressive involuntary movement such as dystonia or drug-induced dyskinesia, or those with dysphagia or upper respiratory tract problems may be suitable for general anesthesia. Rigid neck fixation may be intolerable in some cases; thus, sedative drugs should be prepared. Propofol is preferable because its effects on microelectrode recordings and evaluation of the effects of macrostimulation are less than other anesthetics. The patient is fixed to the stereotactic frame adapter for the operating table. Overflexion of the neck may cause respiratory failure and dysphagia, as well as aspiration pneumonia; therefore, frame adaptation is very important for future procedures. The upper body is elevated to 30° to prevent continuous cerebrospinal fluid (CSF) leak. Electrocardiography is set up. A sphygmomanometer is put on the upper limb of the one side, and an oximetry is placed on the index finger of the same upper limb. To examine neuronal activity by passive joint movement or macrostimulation effects for involuntary movement, the monitoring setup during surgery is changed to the contralateral upper limb according to the circumstances. These monitoring devices are particularly useful in patients who need mild sedation because of anxiety or restlessness. Blood pressure should be maintained lower than 120/90 mmHg by intravenous bolus or continuous injection of antihypertensive drugs. Transparent draping is preferable because the surgeon can observe the patient’s landmark, consciousness, and neurological findings. The intraoperative fluoroscopy C-arm is set up, and a simulation is performed to determine if the tip of electrode can be observed. Apparatus for neurophysiological examinations and the computer workstation are placed near the surgeon to ensure trajectory and unit recording.
6.7 Preoperative Targeting
Preoperative frameless MRI is fused to CT images with stereotactic frame. Using this method, AC–PC-based targeting (indirect targeting) and MRI-image guided targeting (direct targeting) are developed. Image data are imported to the workstation (Framelink, Medtronics, USA) by recordable media. CT images are used as reference images and MRI images as working images, and then fused on the workstation. We use 3D T1-MPRAGE with gadolinium-enhanced images as our working images because they show the cortical and sulcal vessels that should be avoided during electrode insertion. Reference CT images are selected in which nine indicators are arranged with almost equal distances. Next, all indicators are registered from the right lower to the left lower in a counterclockwise manner. The anterior commissure and the posterior commissure are verified using the MRI working images (Fig. 6.3). The border of each commissure and the third ventricle are selected. Three other midline landmarks such as the pituitary stalk, inter-mammillary body, midbrain aqueduct, or cingulate cortex are also selected. The tentative target is decided by indirect or direct methods, or a combination of both. Our strategy is AC–PC-based indirect targeting, modified according to the patient’s AC–PC length, with confirmed optical points by neurophysiological examinations. We set the X, Y, and Z coordinates of the tentative target (indirect target) by Schaltenbrand Wahren atlas (Schaltenbrand and Wahren 1977) for STN (10 mm lateral, 2.5 mm posterior, and 4 mm ventral from the mid-commissural point), GPi (20 mm lateral, 3 mm anterior, and 4 mm ventral from the mid-commissural point), and Vim (12 mm lateral, 5 mm posterior, and 0 mm from the mid-commissural point). The red nucleus-based targeting in an axial plane or the nigrocapsular angle in a sagittal plane is also used for indirect targeting of STN (Brouenberg et al. 2011). The dorsal border of optic tract is a landmark for indirect GPi targeting (Starr et al. 2002). On the background of extended application of DBS for involuntary movements and psychiatric disorders, neuroimaging-guided electrode placement under general anesthesia without neurophysiological examination or frameless DBS using intraoperative CT and micro-electrode recording has been performed with favorable outcomes (Burchiel et al. 2013; Smith and Bakay 2011).
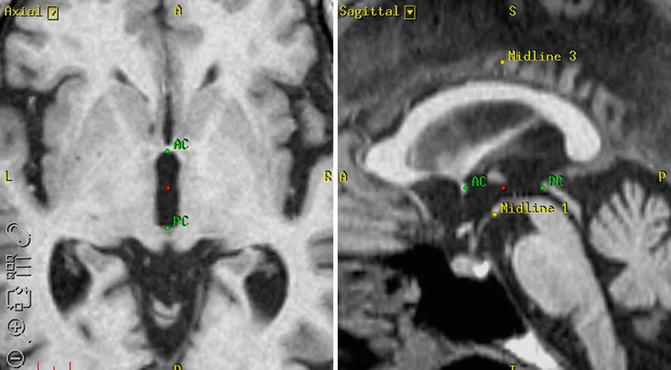
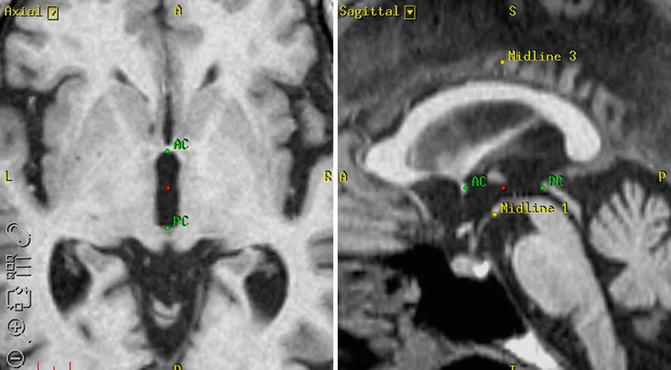
Fig. 6.3
The anterior commissure and the posterior commissure are verified using the MRI working images. The border of each commissure and the third ventricle are selected. Three other midline landmarks such as the pituitary stalk, inter-mammillary body, midbrain aqueduct, or cingulate cortex are also selected. The tentative target can be decided by AC–PC based indirect method
6.8 Preoperative Trajectory
The standard trajectory is 60° to the AC–PC line. In practice, ventricle shape and size can be asymmetrical, and inter-individual differences cannot be disregarded. We must modify the trajectory to the lateral side to avoid ventricular penetration, which may increase hemorrhage risk and electrode malposition (Zrinzo et al. 2009). Additionally, cortical vessels or sulci should be avoided. MRI images showing visible vessels, 3D T1-weighted images may assist in avoiding these complications (Fig. 6.4). The Leksell Multi Arc is set to the frame. The Y and Z scales are confirmed by two nonsterile assistants and fixed. The X scale is confirmed by two sterile surgeons. The scale of arc (lateral rotation) and ring (anterior–posterior rotation) is input to the workstation. The probe is set at the presumed burr hole, and the trajectory is verified virtually to avoid penetration into the sulci, cortical vessels, or lateral ventricle. The scale of arc and ring is modified if necessary. The design of the presumed burr holes and skin incisions is marked.
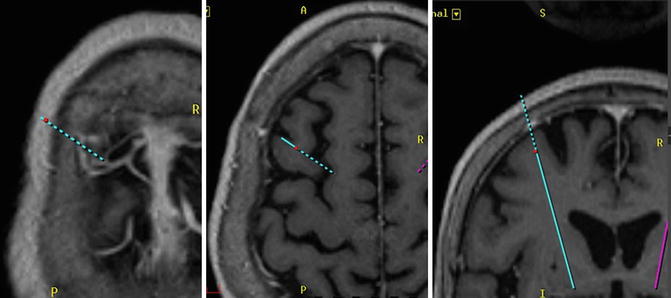
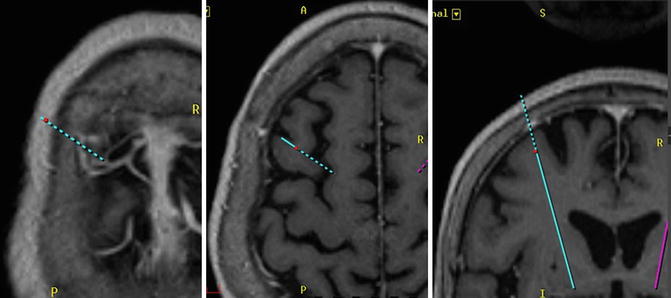
Fig. 6.4
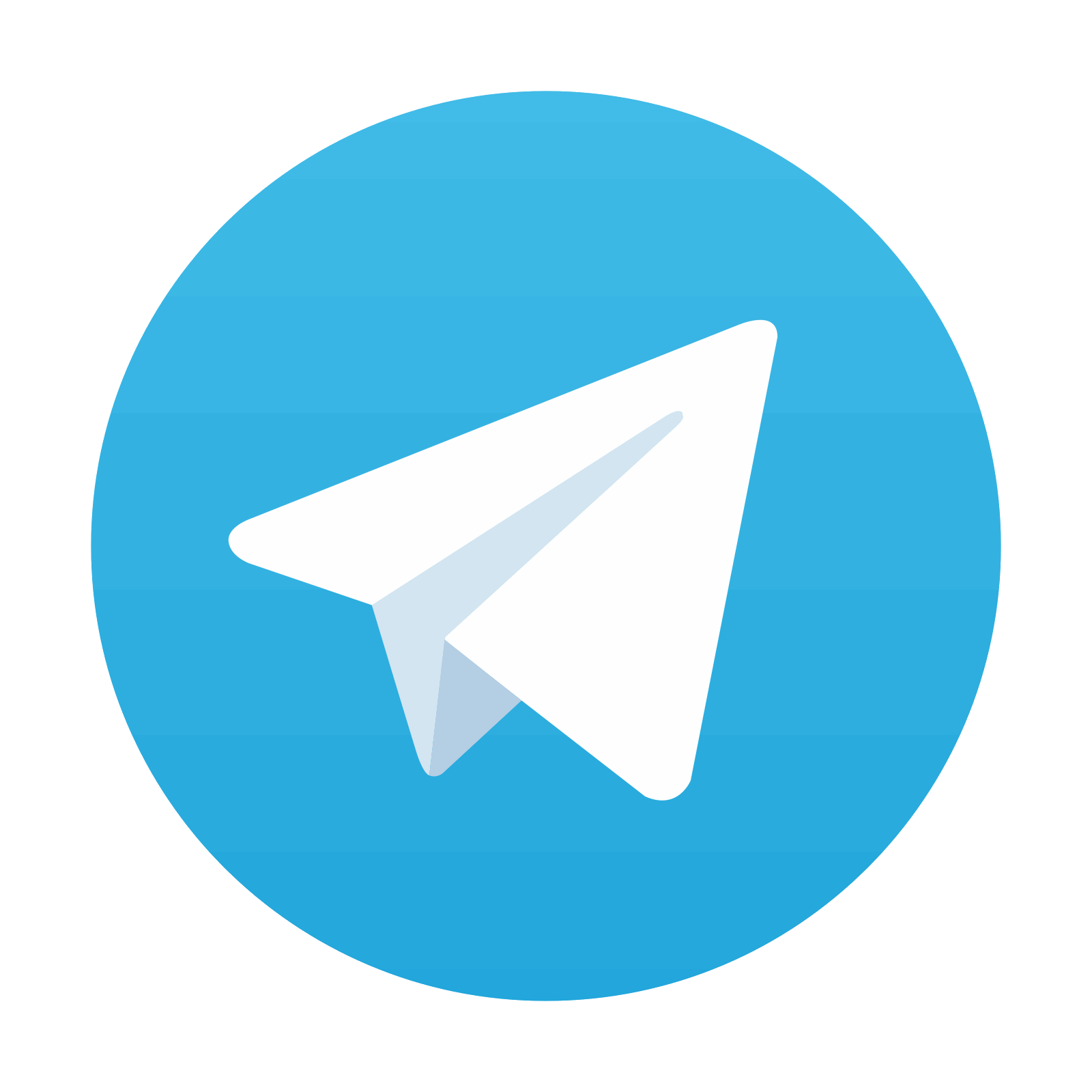
Three-dimensional T1-MPRAGE images with gadolinium-enhanced images are used for planning trajectory. They show the cortical and sulcal vessels that should be avoided during electrode insertion. The trajectory is verified virtually to avoid penetration into the sulci, cortical vessels, or lateral ventricle
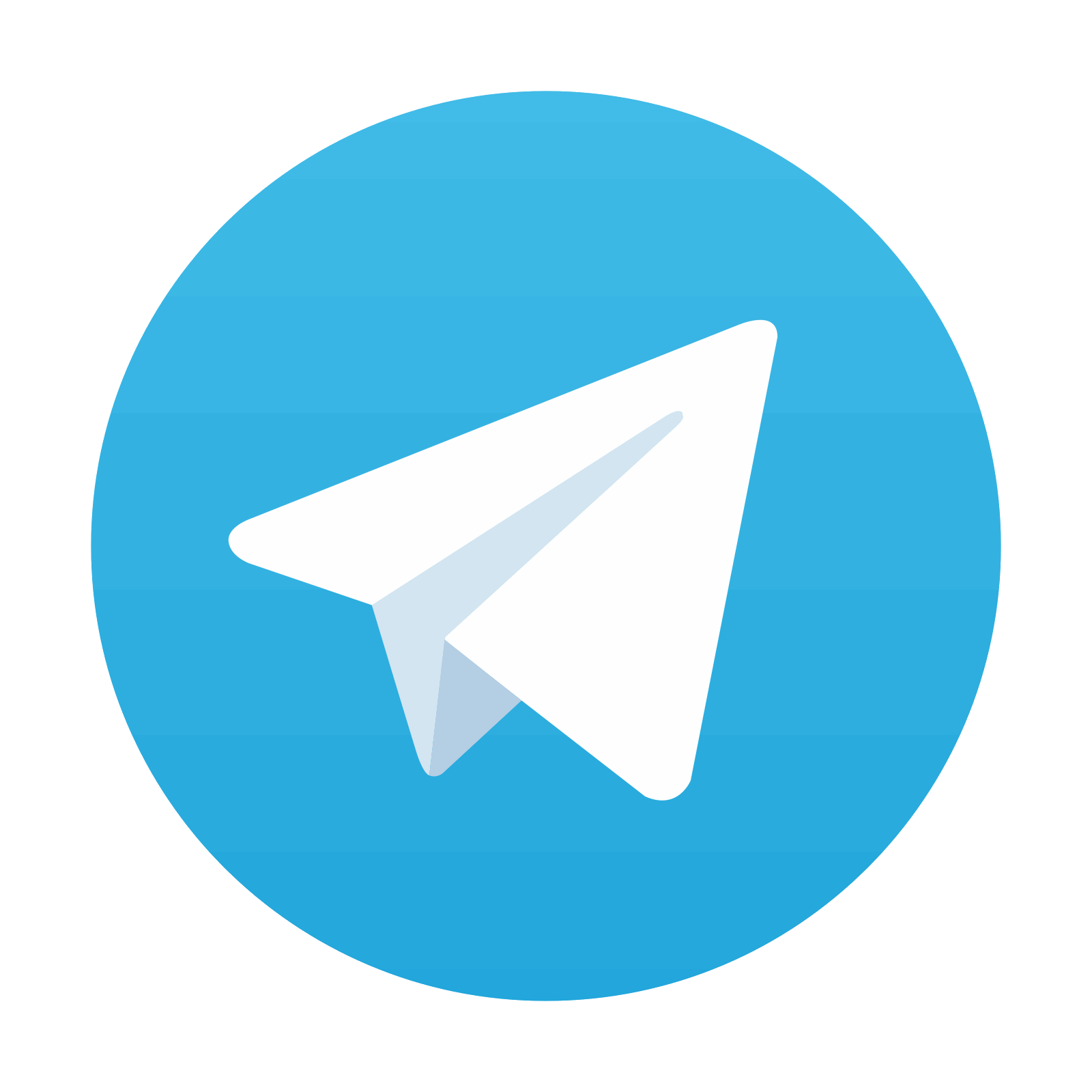
Stay updated, free articles. Join our Telegram channel

Full access? Get Clinical Tree
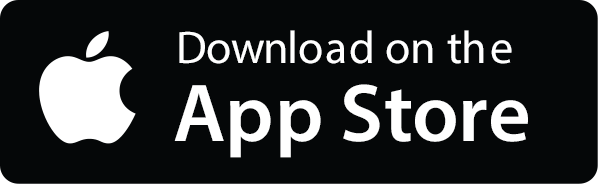
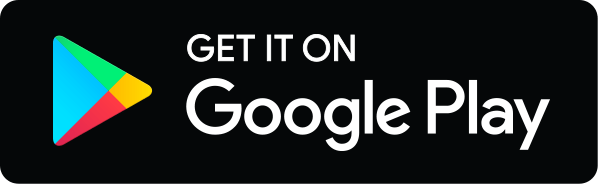
