13 The concept of radiosurgery, which combines principles of stereotactic localization with multiple beams from a high-energy radiation source, was developed in 1951.1 Over the last several decades, this noninvasive technique has proven to be an effective alternative to conventional neurosurgery and irradiation for selected small cranial tumors and arteriovenous malformations. Because previous stereotactic techniques have relied on rigid target fixation, almost all cases treated with radiosurgery have involved intracranial lesions. Stereotactic radiosurgery could be used to treat extracranial lesions if its accuracy could be maintained outside the cranium. Intracranial radiosurgery is practical because lesions are fixed with respect to the skull, which can be rigidly immobilized in a stereotactic frame. Spinal lesions have a fixed relationship to the spine, but fixating the spine in a frame is technically challenging and cumbersome. Skeletal fixation for radiosurgical treatment of spinal tumors has been reported.2 In these cases, a body frame is used to fixate metal clamps to the spinous process of patients, thereby allowing localization. More recently, 19 patients with spine metastases underwent modified linear accelerator (LINAC) radiosurgery after surgical placement of metal clamps for rigid fixation.3 However, the clamps must be placed under general anesthesia, and patients are subjected to the potential for complications (e.g., two wound infections occurred in the above 19 patients). Furthermore, the need for anesthesia, surgery, and radiosurgical treatment results in a lengthy procedure. Fixed spinal frames also limit the degrees of freedom available for treatment, and the metal components of frames produce imaging artifacts on computed tomography (CT) and magnetic resonance imaging (MRI). Finally, the discomfort associated with skeletal spinal fixation makes fractionation impractical and the treatment of children difficult. For spinal tumors, the use of conventional radiation therapy is well established, but the spinal cord’s low tolerance for radiation often limits the treatment dose to a less than optimal level.4 The risks of radiation-induced spinal cord injuries such as myelopathy are also documented. In conventional fractionated radiation therapy to the cervical and thoracic spine, this incidence ranges from 0.2 to 5%.4–7 Radiation therapy can also cause spinal cord edema, which may or may not resolve upon completion of therapy.8 If the radiation dose can be confined more precisely to the treatment volume, the likelihood of tumor control should increase, whereas the risk of spinal cord injury is mitigated; however, conventional external beam radiotherapy typically relies on manual patient positioning, which lacks the precision to allow large doses of radiation to be aimed at targets near radiosensitive structures. Attempts to increase the dose while protecting the spinal cord with conformal radiotherapy have met with only limited success.9 The Cyberknife stereotactic radiosurgery system was developed as a noninvasive method for aligning treatment beams precisely with targets (Fig. 13-1). It incorporates several advances that allow the treatment of spinal lesions without rigid fixation. First, it is a lightweight 6-MV X-band LINAC, designed especially for radiosurgery and mounted to a highly maneuverable robotic manipulator (GMFanuc, Auborn Hills, MI). The Cyberknife operates at a radiofrequency of ~7.5 GHz compared with 2.9 GHz for a typical medical S-band LINAC. Consequently, the dimensions of the 6-MV accelerator cavity are decreased by a factor of 2.5. Less shielding is required, and the collimators for narrow radiosurgical fields are much smaller than those required for large therapeutic fields. These parameters lead to an x-band accelerator head that measures 25 × 45 × 70 cm and weighs only 130 kg. A LINAC of this size can be carried by the robotic arm. The heavier clinical S-band accelerators are far beyond the load limits of robotic arms and require a substantial gantry that limits their positioning capability. The robot can position and point the LINAC with 6 degrees of freedom and has a pointing precision of 0.3 mm. FIGURE 13-1 The Cyberknife at Stanford University School of Medicine. Second, the Cyberknife references the position of the treatment site to internal radiographic features such as skeletal anatomy in the cervical spine or implanted fiducials in the thoracic and lumbar spine. This feature allows radiosurgical treatment without a frame and makes treatment of spinal, pancreatic, and even pulmonary tumors possible. Third, the Cyberknife uses real-time image guidance, thereby eliminating the need to position and immobilize the target via skeletal fixation (e.g., with a frame). This imaging system acquires digital radiographs of skeletal features and fiducials associated with the treatment site and uses image registration techniques to determine the coordinates of the treatment site with respect to the LINAC robot. The Cyberknife imaging system transmits the target coordinates to the robot, which then directs the beam to the treatment site. If the target moves, the process detects the change and corrects the trajectory of the beam. This process is rapid enough that the system reacts in near real time to both translational and rotational changes in the patient’s position. Fourth, the Cyberknife directs each radiosurgical beam independently, without the constraint of a fixed isocenter. The result is non-isocentric-based treatment. Changes in the patient’s position during treatment are compensated for by adaptive beam pointing rather than accepted as a setup error or controlled through rigid immobilization. Consequently, the patient can be positioned in the treatment room without precisely reproducing the position in the treatment planning study. Fifth, the development of amorphous silicon detectors has improved radiographic imaging. Frameless radiosurgery was already used to treat sites within the cervical spine10–13 before amorphous silicon detectors were available. Earlier radiographic cameras were fluoroscopes consisting of a gadolinium-oxysulfide screen viewed by a light-amplified charge-coupled device (CCD) video. Lens optics require the CCD to be 60 cm from the screen. As a result, the signal-to-noise ratio is poor at low exposure levels, contrast is low, and the veiling glare is significant. This design has made it difficult to obtain good-quality images of the skeletal anatomy within and around the thorax and abdomen. To overcome these limitations, the Cyberknife now uses Flashscan 20 flat-panel amorphous silicon x-ray cameras (dpiX, LLC, Palo Alto, CA).14 These devices have a 19.2 × 24 cm active area and a pixel pitch of 0.125 mm. They acquire flat images that avoid distortions inherent to lensed or radiographic image-intensifier techniques. The result is a 10-fold improvement in spatial resolution. This imaging software and hardware have been designed specifically to provide variable fields of view and magnification ranges that can be adapted to multiple anatomic locations. For example, amorphous-silicon x-ray sensors create a high-quality image of the lumbar spine using the typical Cyberknife imaging geometry (10 mAs, 75 kV x-ray exposure14). Such an exposure corresponds to a dose per image of ~25 mrad. The components of the imaging system are fixed at known positions within the treatment room. This feature provides a stationary frame of reference for locating the patient’s spinal lesion, which, in turn, has a known relationship to the reference frame of the robotic arm and LINAC. As with conventional forms of brain stereotaxy, this approach assumes a fixed relationship between the target and the skeletal system (e.g., spine). Once the spine has been located within the coordinate frame of the imaging system, the position of the lesion is known. The Cyberknife determines the location of the spine in the coordinate frame of the radiation delivery system by comparing digitally reconstructed radiographs (DRRs) derived from the treatment-planning CT study with radiographs acquired by the real-time imaging system. The DRRs are produced using a computer model that replicates the actual fluoroscope geometry and optics. So if the patient’s spine is positioned in the coordinate frame of the treatment room in precisely the same way as in the treatment-planning CT study, the radiographs and the DRRs will be identical. If the positioning is not the same, the system calculates the differences using either of two different algorithms. One algorithm uses a large database of precomputed DRRs to simulate the full range of possible positions during treatment. The acquired radiographs are correlated with each of the precomputed DRRs to obtain a measure of their similarity. The degree of correlation with each DRR is used to interpolate to the most probable actual position of the cranium or spine in the imaging reference frame. The DRRs are computed before treatment begins; an array processor computes the correlation in near real-time during treatment. This algorithm accurately measures translational position but cannot determine all rotational effects. Consequently, the orientation of the patient’s spine is maintained using a noninvasive AquaPlast(WFR/AquaPlast Corp., Wyckoff, NJ) mask (Fig. 13-2) for cervical lesions or an AlphaCradle(Med-Tec, Orange City, LA) (Fig. 13-3) for thoracic and lumbar lesions. FIGURE 13-2 Patients with intracranial or cervical spine lesions are immobilized in an AquaPlast mask. FIGURE 13-3 Patients with thoracic or lumbar spine lesions are immobilized in an AlphaCradle. The most recent Cyberknife software uses a more advanced algorithm15 that measures both translation and rotation of the anatomy by iteratively changing the position of the anatomy in the DRR until the two radiographs and two DRRs match exactly. This algorithm does not require the database of precomputed DRRs or the array processor, and it eliminates the need to fix the patient’s orientation during treatment. Once the position of the spine has been determined, the coordinates are relayed to the robotic arm, which adjusts the pointing of the LINAC. The speed of the imaging process and the maneuverability of the lightweight LINAC on the robotic arm allow the system to detect and adjust to changes in the position of the spine in near real time (less than a second). With this capability, a rigid stereotactic frame is unnecessary either as a method to position the treatment site at a fixed beam isocenter or to restrain the patient. Cervical tumors can readily be targeted using bony cervical landmarks. The mass of the soft tissue of the cervical spine is not great enough to prevent optimal imaging of the region; however, when treating thoracic lesions and tumors within the abdominal and thoracic cavity, a soft tissue mass often precludes optimal imaging of bony landmarks. In these cases, tiny metal fiducials can be placed percutaneously adjacent to the lesion. In each case, the fiducials have a fixed relationship with the target. By imaging these fiducials, the location of the lesion is known.
The Cyberknife
Advantages of the Cyberknife
Localization of Spinal Targets with the Cyberknife
Targeting Spine Lesions with Fiducials
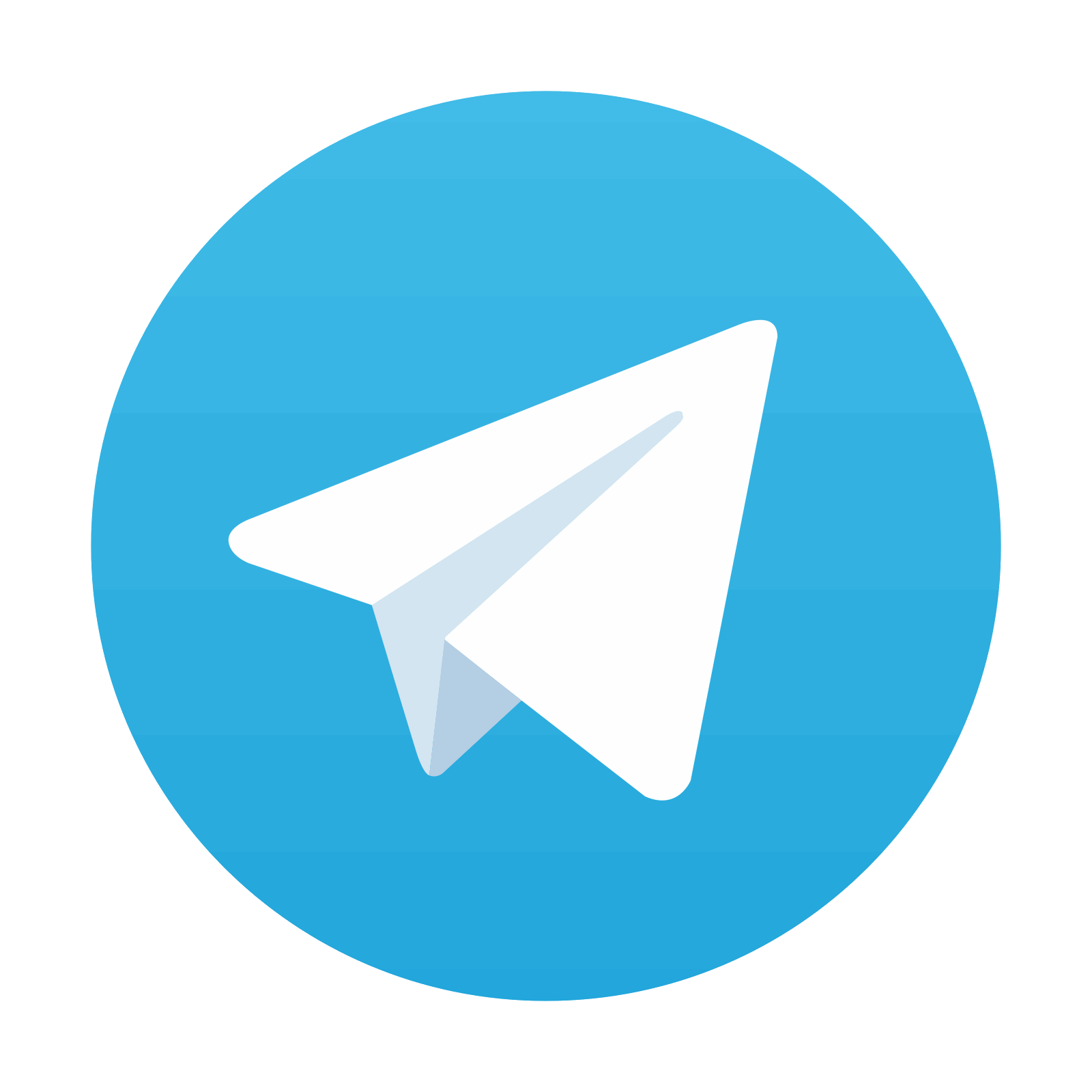
Stay updated, free articles. Join our Telegram channel

Full access? Get Clinical Tree
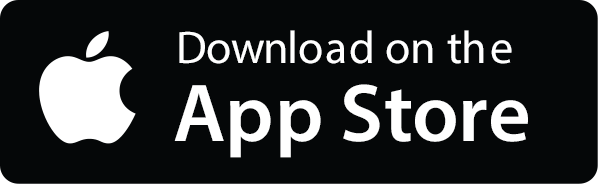
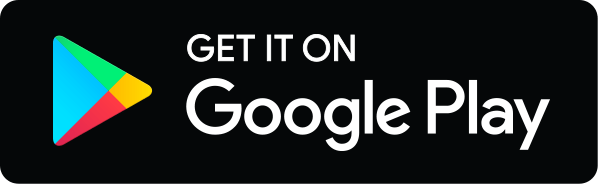