Simplified depiction of acquired factors implicated in the etiopathogenesis of two neurodegenerative diseases: Alzheimer’s Disease and chronic traumatic encephalopathy.
Myelin repair
The idea that myelin in the brain may have some capacity to regenerate after its injury has long intrigued neurologists, and the often-disabling sequelae of demyelination in young adults with multiple sclerosis (MS) has stimulated the most interest (Lassmann et al., 1997; Franklin and Ffrench-Constant, 2008). More recently, thought has also been given to the repair of white matter ischemic and traumatic injuries (Salat, 2014; Shi et al., 2015). Interest in myelin repair has surged in other contexts as well, and the idea may have broad implications for the treatment of neurologic disease (Maki et al., 2013).
As a first point in this discussion, it is important to recognize that myelin repair occurs as a normal phenomenon in adulthood and aging. Although white matter volume declines in the aging process, as reviewed in Chapter 3, remyelination is evident in normal adulthood, and has been observed well into late life (Peters, 2009). Without this phenomenon, thought to be an ongoing response to oligodendrocyte stress and subsequent apoptotic death (Peters, 2009), the loss of white matter volume in aging would be even greater. The mechanism of remyelination in aging is thought to involve the differentiation of new oligodendrocytes from oligodendrocyte precursor cells (OPCs), also known as NG2 cells, which are scattered throughout the central nervous system (CNS) and constitute 5% of all glial cells (Levine, Reynolds, and Fawcett, 2001). In aging, the number of oligodendrocytes produced by this process of NG2 differentiation may increase by as much as 50% (Peters and Sethares, 2002). Given that oligodendrogenesis occurs under normal conditions, a natural assumption would be that the potential exists to facilitate this process for therapeutic benefit.
Vascular white matter disease is relevant in this context because myelin repair after vascular injury may be mediated by the formation of new oligodendrocytes. Recent laboratory investigations have demonstrated that oligodendrogenesis is stimulated by cerebral ischemia (Zhang, Chopp, and Zhang, 2013). Experimental studies have also suggested the possibility that several exogenous agents may be able to further enhance oligodendrogenesis after ischemia; one such drug is the familiar nonprescription drug aspirin (Chen et al., 2014), and others include erythropoietin, thymosin β4, cerberolysin, and sildenafil (Zhang, Chopp, and Zhang, 2013). In addition, myelin repair can conceivably be enhanced by angiogenesis within damaged white matter. This possibility is most relevant to stroke and related vascular white matter damage (Salat, 2014) but may also apply to TBI, in which microvascular injury regularly accompanies DAI (Xiong, Mahmood, and Chopp, 2010). Stimulation of angiogenesis has been observed with a diverse array of agents, including statins, sildenafil, erythropoietin, and various growth factors (Xiong, Mahmood, and Chopp, 2010), and the benefits of increasing perfusion to injured white matter by such treatments could be profound.
Turning to MS, remyelination would clearly be a direct means of addressing the fundamental pathology of this common disease. Remyelination is known to occur in MS, but the extent of effective repair of demyelinated axons is generally regarded as disappointingly modest (Franklin and Ffrench-Constant, 2008). Despite the repeated demonstration of the efficacy of remyelination in animal models of the disease, patients with MS are generally thought to gain clinical benefit only infrequently from this process (Franklin and Ffrench-Constant, 2008). Of interest, an exception to this rule may exist in the case of cortical remyelination. Recent evidence has indicated that remyelination in the cerebral cortex of MS patients is considerably more extensive than in lesions within large white matter tracts (Albert, Antel, and Brick, 2007; Chang et al., 2012). The result of this phenomenon may be the attenuation or abolition of cognitive deficits related to cortical lesions in MS, and robust cortical remyelination may help explain why the cognitive profile of MS more resembles other white matter disorders than cortical dementia such as AD (Chapter 7). However vigorous the remyelination of cortical versus subcortical lesions may be, the idea remains highly attractive, and investigation continues.
One line of inquiry considers the capacity of available immunotherapeutic agents for MS to enhance remyelination (Keough and Yong, 2013). Two of the existing drugs for this disease – glatiramer acetate and fingolimod, the first oral medication for MS – have been shown experimentally to have some potential for remyelination, but clinical data to support this capacity are at present lacking (Keough and Yong, 2013). The consensus view (Keough and Yong, 2013) is therefore that drugs specifically designed to enhance remyelination will be needed to complement the immunomodulation available through the existing MS armamentarium.
Looking ahead to new treatments, it is becoming clear that foundational to this work is the recognition that myelination is under the control of a wide range of molecular signals that could potentially be manipulated with beneficial result (Mitew et al., 2014). These signals are of two types: those that enhance myelination, and those that act to inhibit the process. Treatments for MS, and perhaps other white matter disorders in which remyelination is a goal, would therefore logically be aimed at either stimulating the factors that enhance myelination or inhibiting those that have the opposite effect. An agent that might stimulate remyelination, to cite but one example, is retinoic acid (Huang et al., 2011), and one intended to block the inhibition of remyelination is the anti-LINGO-1 antibody (Mi, Pepinsky, and Cadavid, 2013).
More recent information has introduced the idea that endogenous systems exist for the repair of white matter by potentiating the activity of normal oligodendrocytes. These systems may be restorative to white matter after damage of many varieties. One such system involves neuregulin, a protein growth factor that has been well recognized in the peripheral nervous system (PNS) and more recently in the central nervous system (CNS) as well (Hu et al., 2006). In the brain, neuregulin has been proposed to protect myelinated neurons in perinatal white matter injury (Dammann et al., 2008), and its contribution to myelination has been implicated in a variety of adult neuropsychiatric disorders (Mei and Nave, 2014). One key role of neuregulin appears to be its capacity to induce oligodendrocytes to remyelinate axons after white matter damage has been sustained (Lundgaard et al., 2013). Because neuregulin is activated by the enzyme β-secretase, also known as β-site APP cleaving enzyme (BACE), the generation of new myelin in response to injury may be accompanied by the formation of β-amyloid from its parent molecule amyloid precursor protein (APP) (Evin, Barakat, and Masters, 2010). Thus, because BACE has a dual mechanism, the restorative process of myelin repair may involve a simultaneously damaging accumulation of β-amyloid (Evin, Barakat, and Masters, 2010). As postulated by Bartzokis (2011), cortical β-amyloid may be a by-product of the continuous activation of neuregulin as it attempts to repair damaged myelin. The role of neuregulin with respect to both white matter repair and AD pathogenesis is thus of potentially great interest, and will be discussed further in the next chapter.
A related idea invokes the role of the apolipoprotein E (APOE) genotype in myelin repair, as it has been found that the APOE ε4 allele, with its associated protein ApoE ε4, is less effective in this process than the other common alleles ε2 or ε3. ApoE has many functions in the brain, among them the potentiation of β-amyloid formation, but its primary role is the transport of cholesterol, principally from astrocytes to neurons (Bu, 2009). The APOE ε2 and ε3 alleles may assist in myelin repair because they enhance the delivery of cholesterol and other lipids to damaged axons, whereas the ε4 allele may have the opposite effect (Bu, 2009). Recent DTI studies have in fact shown that the presence of the APOE ε4 allele exacerbates white matter injury related to vascular risk factors in older people, and accelerates cognitive decline (Wang et al., 2015) as assessed by the Mini-Mental State Examination (Folstein, Folstein, and McHugh, 1975). This genetic influence on myelin repair may also be relevant to AD. As again proposed by Bartzokis (2011), the APOE ε4 allele may predispose to AD by hindering cholesterol transport to myelin that has been damaged by vascular and other age-related insults. In contrast, the ε2 allele may be protective against AD because of more effective delivery of cholesterol to axons that enhances their repair (Bartzokis, 2011). This notion is supported by neuroimaging studies showing that cognitively normal APOE ε4 carriers have (1) lower magnetic resonance imaging (MRI) white matter volume that correlates with slower processing speed (Ready et al., 2011), (2) decreased frontal white matter FA on DTI that correlates with lower executive function (Ryan et al., 2011), and (3) disrupted structural connectivity and diminished memory performance (Chen et al., 2015), whereas APOE ε2 carriers have higher FA in many white matter tracts (Chiang et al., 2012). The following chapter will consider in more detail the possible role of ineffective white matter repair in the pathogenesis of AD.
Stem cells
The idea of stem cell therapeutics has been attracting much attention in recent decades as a means of restoring normal function in the brain and spinal cord. The most obvious approach using stem cells is the targeting of damaged neurons, and the degenerative diseases offer many opportunities to test the efficacy of this strategy. Hence diseases such as Parkinson’s Disease, HD, AD, and amyotrophic lateral sclerosis have been examined with regard to the possibility of replacing expiring or absent neurons with stem cells that can assume the function of the dysfunctional or lost cells (Lunn et al., 2011). The replacement cells could be implanted via grafting procedures or possibly delivered intravenously or intrathecally to the desired site in the brain. Whereas these exogenous stem cells have much promise in the treatment of diseases characterized by primary neuronal loss, the possibility also exists that endogenous stem cells can be exploited for the treatment of white matter disorders such as MS in which myelin damage is prominent (Franklin and Goldman, 2015). In this scenario, medical intervention would be intended to augment endogenous myelin repair mechanisms. Whereas the idea of using stem cells for treating neurologic disease has garnered most attention with respect to gray matter disease, stem cells may prove equally or even more efficacious for the treatment of white matter disorders (Maki et al., 2013; Braun and Jessberger, 2014).
The concept that neural stem cells could contribute to the prognosis of any neurologic disorder – originating in either gray or white matter – was not considered until recent years. For most of the history of neuroscience, it was firmly believed that neurologic and neurobehavioral functions are subserved by post-mitotic neurons, and recovery is solely a function of the activity of those neurons that remain intact after brain insult. Beginning in the 1960s, a remarkable series of observations has produced the steady accumulation of evidence indicating that neurogenesis – the generation of new neurons – actually does occur in the adult human brain (Ming and Song, 2011). Under normal conditions, these neurons are generated from precursor cells in two primary sites: the dentate gyrus of the hippocampus, and the subventricular zone adjacent to the lateral ventricle (Ming and Song, 2011). More recently, it was recognized that new glial cells are also generated in the adult human brain, and, in particular, OPCs that are widely distributed in the brain can differentiate into functional oligodendrocytes (Levine, Reynolds, and Fawcett, 2001). With the knowledge now accumulating on various stem cells, a new vista of opportunities has been revealed for improving the outcome of devastating disorders of cognition, many of which implicate white matter neuropathology.
Of the white matter disorders, MS, cerebral ischemia, the leukodystrophies, and periventricular leukomalacia have attracted the most attention as diseases that could be treated by stimulation of endogenous OPCs, the use of exogenous stem cells, or both (Goldman, Schanz, and Windrem, 2008; Ben-Hur, 2011; Zhang, Chopp, and Zhang, 2013; Vanderver et al., 2014; Chen et al., 2014). In the case of MS, in which remyelination is notoriously incomplete (Franklin and Ffrench-Constant, 2008), researchers have pondered the use of endogenous or exogenous stem cells as an approach to the long-elusive challenge of enhancing remyelination (Ben-Hur, 2011). From a clinical perspective, there are many obvious advantages of using the brain’s existing precursor cells to repair myelin, and the idea that even a drug as familiar as aspirin (discussed previously) could be useful in enhancing oligodendrogenesis is most appealing. The relative simplicity and safety of stimulating endogenous brain cells to repair the white matter render this option preferable to the use of invasive methods – most notably intracerebral implantation of exogenous stem cells – that would entail more risk and expense. However, it has been pointed out that the typically feeble remyelination in MS is in part due to the axonal loss that occurs in many areas of demyelination, and because this process can occur early in MS, all options should be kept open (Ben-Hur, 2011). Indeed, an open-label study of intravenous and intrathecal mesenchymal stem cells in MS patients suggested clinical improvement without major side effects (Karussis et al., 2010).
Stem cell neurobiology, however, while surely exciting in ways that could not be imagined even a few decades ago, is still a field that is clearly in its formative stages, and much remains to be learned. In addition to ethical issues centering on the use of fetal tissue that dominated the field in its early years and at times still prove contentious, the technical challenges of this approach are far from trivial. Research will continue, and clinicians will await with interest the outcomes of many parallel investigations. The ultimate utility of stem cell therapeutics will likely depend not only on the details of how both endogeous and exogenous cells may be exploited, but also on the specific neuropathology and pathogenesis of the disorder being considered for treatment.
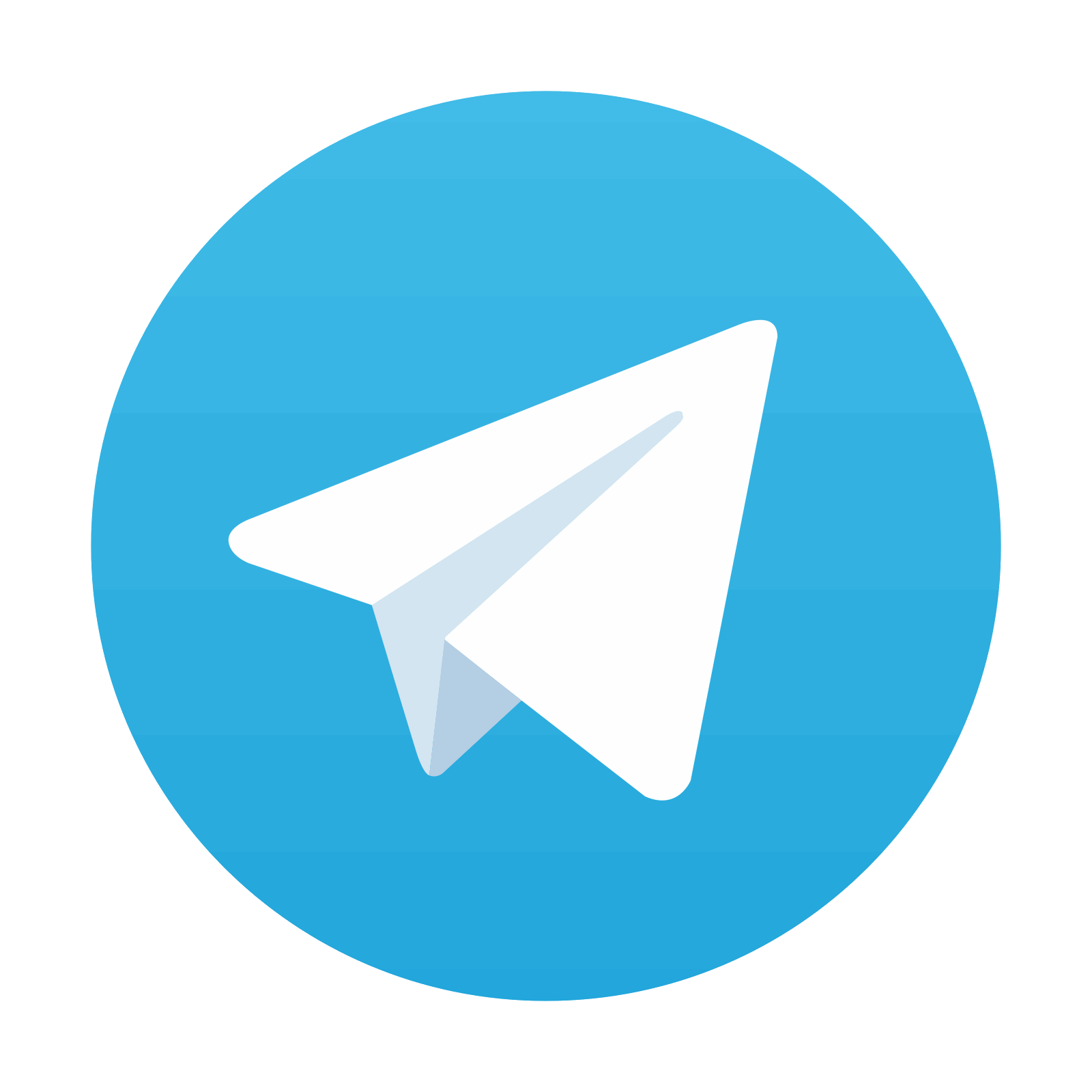
Stay updated, free articles. Join our Telegram channel

Full access? Get Clinical Tree
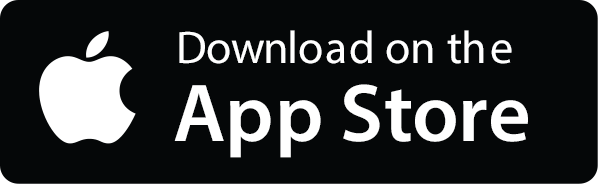
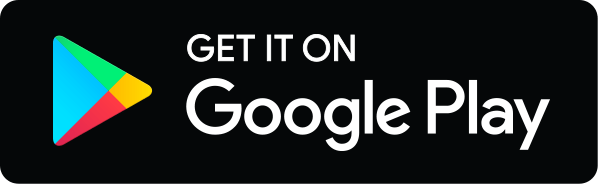