Abstract
The course of many diseases can be significantly improved by therapeutic modulation of inflammation. Currently available therapy for regulation of inflammation is often suboptimal. There is great promise in the bridging of neuroscience, immunology, engineering, and computer science, i.e., bioelectronic medicine (neuromodulation), to develop new technology that therapeutically targets inflammatory disease: Discoveries in neurophysiology and immunology make it possible to consider using nerve stimulators to regulate inflammation. Experimental animal studies show that electrical activation of the autonomic nervous system, e.g., the vagus nerve, can improve outcomes in acute and chronic inflammation. Results from clinical studies using vagus nerve stimulation to treat rheumatoid arthritis or Crohn’s disease in man are encouraging. Further mechanistic understanding of the neurophysiology and molecular biology that underlies neural reflex control of the immune system may enable development of conceptually novel modalities for treatment of inflammatory disease.
Keywords
Bioelectronic medicine, Cholinergic antiinflammatory pathway, Immune system, Inflammatory disease, Technology targeting molecular mechanisms
Outline
Therapeutic Challenges in Inflammatory Disease 1483
The Nervous System is an Integral Part of the Immune System 1484
The Inflammatory Reflex 1484
The Inflammatory Reflex in Experimental Disease 1488
Vagus Nerve Stimulation in Experimental and Clinical Disease 1488
Bioelectronic Medicine 1488
Conclusions 1489
References 1489
Therapeutic Challenges in Inflammatory Disease
Inflammation is essential for antimicrobial defense and tissue healing but it must be proportional to the injury or threat and very carefully regulated to restore and maintain homeostasis and health. Excessive and nonresolving inflammation disrupts homeostasis and causes inflammatory diseases. Immune system dysregulation underlies septic shock, rheumatoid arthritis, inflammatory bowel disease, psoriasis, multiple sclerosis, atherosclerosis, certain cancers, and a range of other diseases. Consequently, world-wide, nonresolving inflammation causes significant morbidity and mortality ( ).
The course of many diseases can be significantly improved by therapeutic modulation of inflammation. Steroidal and nonsteroidal antiinflammatory drugs, small molecule compounds, and specific anticytokine therapies are widely used in current clinical practice. However, these treatments may cause serious side effects and yet fail to provide satisfactory relief for a significant group of patients. Development of effective therapies with limited side effects to regulate specific inflammatory processes has proven challenging, and paradigm-shifting innovations are needed ( ).
Neural reflex circuits are key regulators of organ function and homeostasis. For example, heart rate, blood pressure, and body temperature are closely monitored by the nervous system and regulated by neural signals. The brain and the nervous system play key roles in maintaining physical balance and homeostasis under various conditions and challenges. Developments in bioelectronic medicine, defined as the convergence of molecular medicine, neuroscience, engineering, and computing, have revealed that neural reflexes also regulate inflammation, cytokine release, and a range of immune system functions. These new discoveries offer a conceptually new approach to use medical devices that modulate nerve activity to regulate inflammation and treat inflammatory disease. It is now conceivable to use electricity instead of drugs to target therapeutic molecular mechanisms through either stimulation or inhibition of peripheral nerves.
The Nervous System is an Integral Part of the Immune System
About 2000 years ago, described the hallmarks of inflammation, which include local redness, swelling, heat, and pain (Original before 47 CE.). A component of pain in peripheral inflammation is detected through increased signals in sensory nerve fibers. The signals travel to the brain and constitute one of the routes that convey information on the intensity and localization of the insult, and alert the organism to the trigger. Inflammation often elicits a “sickness syndrome,” which includes fatigue, loss of appetite, decreased social interactions, and increased sleep. These behavioral changes, in response to immune system activation, are orchestrated by the central nervous system (CNS) through a network of neural signals ( ). Hence, interactions between the immune system and the nervous system influence organism behavior. However, the detailed physiology of these interactions and the underlying molecular mechanisms have long remained unexplored, and much remains to be discovered.
Interestingly, neural control of inflammation is an ancient component of animal physiology ( ). Caenorhabditis elegans ( C . elegans ) is a nematode equipped with a feeding system, gut, and a nervous system, but lacks skeletal elements and a circulatory system. It is one of the least complex animals with a nervous system. In this relatively simple organism, the innate immune response to bacterial invasion of nonneural cells is regulated by nerve signals ( ). Neural reflexes constitute an integral part of the immune system in higher vertebrates as well, and our understanding of the underlying molecular mechanisms has expanded significantly ( ).
Importantly, specific pain fibers detect and respond to inflammation and have the capacity to regulate local inflammatory responses. Sensory, or nociceptor, nerve fibers can directly detect bacterial invasion and play a direct role in inhibition of the local inflammation, elicited by microbial invasion ( ). In other experimental models of inflammatory disease, the interaction between immune cells, tissues, and sensory nerve fibers promote inflammation ( ). Certain sensory nerves can also release substances that promote local inflammation and plasma leakage in response to irritants such as capsaicin in a response often referred to as “neurogenic inflammation” ( ). Thus, depending on the specific interactions between neurons, leukocytes, and tissues, nociceptor neurons can promote or inhibit inflammation ( ).
The CNS plays a vital role in orchestrating the systemic inflammatory response. The CNS receives information on inflammation through “danger receptors,” including toll-like receptors and cytokine receptors that activate central and peripheral signaling pathways. For example, bacterial endotoxin activates the hypothalamic–pituitary–adrenal (HPA) axis. This activation increases levels of antiinflammatory steroids, which suppress release of proinflammatory cytokines ( ). Circuits in the sympathetic and parasympathetic nervous systems also regulate local and systemic inflammation and immune responses ( ). Blocking these pathways disrupts normal physiology: Subdiaphragmatic vagotomy abolishes the centrally mediated hyperthermia induced by intraperitoneal injection of interleukin 1β, illustrating that sensory neurons in the autonomic nervous system have the capacity to detect signs of inflammation ( ). Also, injection of interleukin 1β into the rat portal vein elicits afferent, vagus nerve activity and reflex, efferent signal activity in the splenic nerve ( ) and pathogen-associated molecular patterns, recognized by sensory vagus neurons, can elicit reflex neural activity in efferent pathways ( ). In addition, a range of centrally acting drugs, including activators of central muscarinic receptors, reduce the systemic inflammatory response in murine endotoxemia ( ; ) and the vagus nerve plays a key role in attenuation of both systemic and local inflammation in a range of experimental models ( ) and may also influence development of oral tolerance ( ). Thus, accumulating evidence shows that neural reflex circuits detect microbial invasion, tissue damage and signs of inflammation, and respond with local regulation of inflammation and immunity as part of a systemic adaptation to return the organism to homeostasis and health. The nervous system is functionally an integral part of the immune system.
The Inflammatory Reflex
The vagus nerve, the 10th cranial nerve, innervates many inner organs. This bilateral nerve is composed of about 80% afferent fibers and approximately 20% efferent fibers and it has been estimated that each contains about 10 4 axons in mice and 10 5 axons in humans ( ). Signals within the afferent vagus nerve reach the brainstem in the nucleus tractus solitarius (NTS) in the medulla oblongata. Afferent signals also activate neurons that project to other CNS nuclei, including the hypothalamus ( ), and these connections are important for regulation of neurohormonal responses through the HPA axis and for temperature control, i.e., fever. The NTS is interconnected with the dorsal motor nucleus, which is the origin of the majority of efferent vagus nerve fibers. The vagus nerve travels down the neck and meanders to innervate the thymus and many organs of the chest and abdomen.
The vagus nerve is a key component of the “inflammatory reflex” ( ). This neural conduit is a prototypical immune-regulatory reflex circuit. The sensory component of the reflex includes afferent vagus nerve fibers, which express receptors for cytokines and damage- and pathogen-associated, molecular patterns and the motor component comprises efferent vagus nerve fibers and the signal propagation pathway from the efferent vagus to cholinergic receptors on immune cells in spleen ( ). Activation of the efferent arc of the inflammatory reflex significantly reduces inflammation in a number of experimental models of disease ( ) ( Table 125.1 ).
Disease Model | Mode of Efferent Arc Inflammatory Reflex Activation | Outcome |
---|---|---|
Endotoxemia | Galantamine b | Improved survival. Reduced serum tumor necrosis factor (TNF) ( ) |
Physostigmine b | Attenuated capillary leakage. Decrease interaction time between leukocytes and endothelium ( ) | |
Vagal nerve stimulation (VNS) | Attenuated shock. Reduced TNF in liver and serum ( ) | |
Decreased serum and myocardial TNF ( ) | ||
GTS-21 a | Reduced liver and spleen TNF ( ) | |
Improved survival. Reduced serum TNF ( ) | ||
Cecal ligation and puncture | Neostigmine b and physostigmine b | Improved survival. Reduced serum TNF, IL-1β, and IL-6. Reduced neutrophil lung infiltration ( ) |
GTS-21 a | Improved survival. Reduced serum HMGB1 ( ) | |
VNS | Improved survival. Reduced serum HMGB1 ( ) | |
Prevented hypotension. Reduced hepatic damage. Reduced serum TNF ( ) | ||
Increased survival ( ) | ||
Colitis | VNS | Decreased body weight loss. Improved histological score ( ) |
Pancreatitis | GTS-21 a | Improved histological score. Decreased serum IL-6 ( ) |
Hemorrhagic shock | VNS | Reverted hypotension and prolonged survival. Attenuated serum TNF ( ) |
Intracerebral hemorrhage | Muscarine | Increased HRV. Reduced brain edema. Reduced TNF and IL-1β in brain and spleen ( ) |
Ischemia-reperfusion | VNS | Prevented hypotension. Reduced TNF in heart, liver and serum ( ) |
Suprarrenal aortic | PNU-282987 a | Reduced infarct size. Decreased serum TNF, HMGB1, and troponin I ( ) |
Myocardial | VNS | Reduced lethality. Decreased histopathological changes in heart ( ) |
Renal | GTS-21 a | Improved renal function. Attenuated TNF in kidney. Reduced tubular necrosis ( ) |
VNS | Attenuated kidney injury, reduced plasma TNF. The effect required spleen and α7nAChR ( ). | |
Artery occlusion shock | VNS | Reverted hypotension. Increased survival. Reduced leukocyte infiltration to lung and ileum. Decreased serum TNF ( ) |
Carrageenan-induced inflammation | VNS and GTS-21 a | Inhibited expression of endothelial cell adhesion molecules ( ) |
Arthritis | AR-R17779 a | Improved clinical arthritis score. Reduced synovial inflammation. Reduced plasma and synovial TNF ( ) |
VNS | Reduced joint swelling and reduced histological arthritis score ( ) | |
Burn-induced injury | VNS | Decreased gut permeability ( ) |
Improved pulmonary histopathology ( ). | ||
Hypertension-induced end organ damage | PNU-282987 a | Decreased end organ damage. Decreased TNF, IL-1β, and IL-6, and inhibited nuclear factor kappa B (NFκB) activation in heart, aorta, and kidney ( ) |
Hemorrhage | VNS | Reduced bleeding time independent of changes in heart rate or blood pressure. Increased thrombin/antithrombin III complex generation ( ) |
Postoperative ileus | VNS | Improved gastric emptying. Reduced TNF and IL-6 in peritoneal cavity ( ) |
AR-R17779 a | Improved gastric emptying. Reduced inflammatory cell recruitment ( ) | |
Ventilator-induced lung injury | VNS and PNU-282987 a | Attenuated lung injury. Decreased lung IL-6 and substance P. Decreased plasma IL-6 ( ) |
GTS-21 a | Improved lung function. Decreased TNF lung and plasma levels ( ) |
Neurophysiological and molecular mapping of the efferent arc of the inflammatory reflex has shown that signals travel through the efferent vagus nerve to the celiac ganglion where the adrenergic splenic nerve arises. An intact splenic nerve is required for the integrity of the inflammatory reflex ( ). In the spleen, the splenic nerve signal is relayed by T lymphocytes capable of acetylcholine (ACh) biosynthesis, known as T ChAT cells ( ), which release ACh in response to norepinephrine (NE) ( ). ACh activates α7 nicotinic ACh receptor subunit-containing receptors (α7nAChR) in innate immune cells in the spleen, including monocytes and macrophages ( ), which inhibit release of tumor necrosis factor (TNF) from these cells (see Fig. 125.1 ). The intracellular signaling mechanism that blocks release of proinflammatory cytokines from macrophages in response to cholinergic receptor activation is not yet fully clear, but involvement of Janus Kinase (JAK) 2/Signal Transducers and Activators of Transcription (STAT) 3 and nuclear factor kappa B (NFκB) signaling has been reported ( ).

The role of the spleen in the vagus nerve control of inflammation is of particular interest. The spleen appears to play the role of a “router” for efferent neural information that regulates inflammation and is required for vagal nerve stimulation (VNS) to inhibit inflammation in a range of experimental inflammatory diseases. Splenic immune cells contribute to systemic TNF production and release in systemic inflammation ( ), and nerve cells and lymphocytes in spleen appear in close apposition ( ). This anatomical arrangement likely facilitates interactions between nerves and leukocytes. Notably, the spleen can contribute a substantial proportion of the monocytes that are found in inflammation ( ). Interestingly, VNS and cholinergic agonists reduce leukocyte recruitment to sites of inflammation, limit the proinflammatory phenotype of macrophages and shift the phenotype from “inflammatory” toward “reparative,” and improve outcome in experimental inflammatory disease ( ). The vital role of the spleen is further supported by the observation that an intact spleen and expression of the α7 nicotinic ACh receptor subunit (the key receptor for cholinergic inhibition of macrophage cytokine release ( )) are required for VNS-induced protection from kidney ischemia-reperfusion injury ( ). Even with the abundant direct innervation of the myenteric plexus by vagus nerve neurons, the spleen is also required for vagus nerve-mediated attenuation of experimental colitis ( ). Surprisingly, in the absence of T ChAT cells, which release ACh to relay neural signals in spleen and perhaps elsewhere, the composition of the mouse intestinal microflora shifts significantly ( ). Concurrently, the spleen is dispensable for VNS-induced attenuation of postoperative ileus and inflammation in experimental animals ( ), supporting the notion that, in addition to the inflammatory reflex, many neural conduits that regulate inflammation remain to be mapped in detail and dissected for molecular mechanisms. Since the vagus nerve meanders and extends branches to a multitude of tissues and organs, it is likely that some of these neural connections take part in neuroimmune reflex circuits other than the prototypical inflammatory reflex.
An important part of homeostasis and healing is the resolution of inflammation, which is an active process regulated by specific mediators, called specialized proresolving mediators (SPMs) ( ). Hence, homeostasis in inflammation does not only involve balancing the proinflammatory response to the threat and preventing exaggerated release of cytokines and similar mediators, but it also includes promoting reparative processes and healing, where SPMs play an active role. A discovery by Mirakaj et al. suggests that levels of SPMs are regulated by signals from the vagus nerve. In their study of sterile peritonitis, vagotomized animals showed reduced levels of SPMs and took significantly longer time to resolve inflammation, as measured by neutrophil infiltration, in peritonitis ( ). Ongoing efforts are investigating to what extent SPM release is under neural control in health and inflammatory disease. Taken together, the observations suggest that the inflammatory reflex tonically balances the production and release of inflammatory mediators and also plays an important role in resolution of inflammation ( ).
The neurophysiological and molecular mapping of the inflammatory reflex has produced a number of scientific discoveries (e.g., nerve stimulation for cytokine control and hemostasis, T lymphocytes that relay neural signals, immune cells that reduce blood pressure), identified a number of potential drug targets (e.g., specific central muscarinic, peripheral nicotinic ACh receptors, cholinergic signals that control bleeding) and significantly contributed to developing the field of bioelectronic medicine (e.g., spawning clinical trials using VNS to treat inflammatory diseases, covered in detail later in this section) ( ). These developments underscore the importance and translational promise of mechanistically focused basic research in bioelectronic medicine.
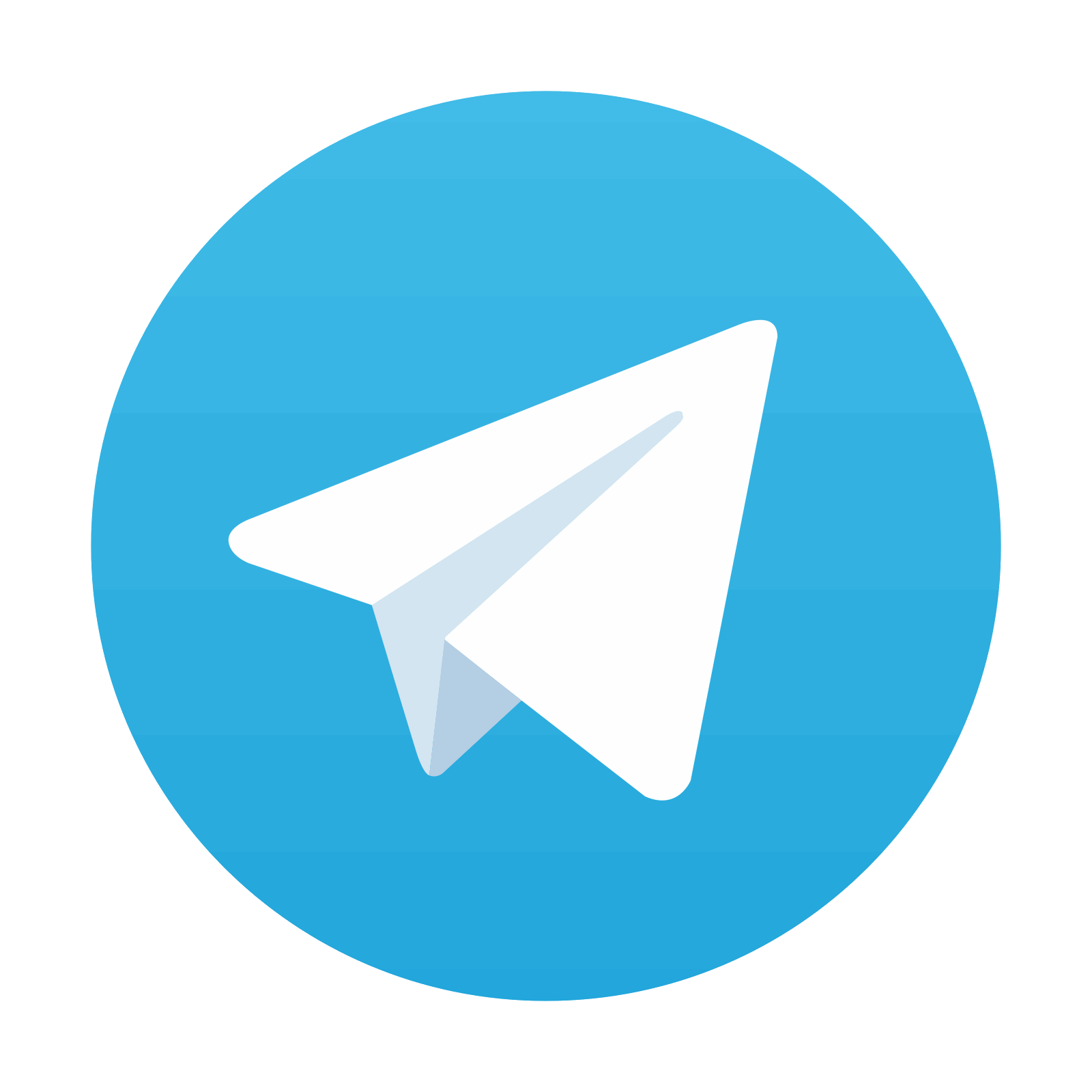
Stay updated, free articles. Join our Telegram channel

Full access? Get Clinical Tree
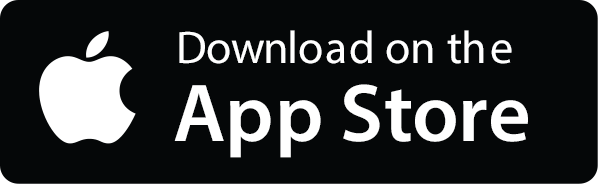
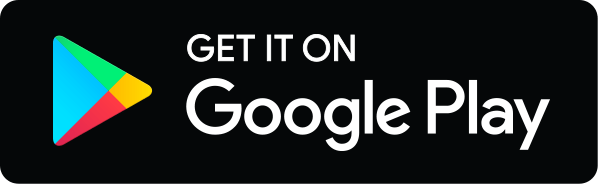