OCT of the anterior chamber. (A) Image of the pupil demarcated by the iris on either side. (B) Angle of the anterior chamber and canal of Schlemm. (C) Corneal endothelium, epithelium, stroma, and underlying anterior chamber. (D) The cornea and sclera meet at the corneoscleral limbus. Images courtesy of Rick Calderon, O.D.
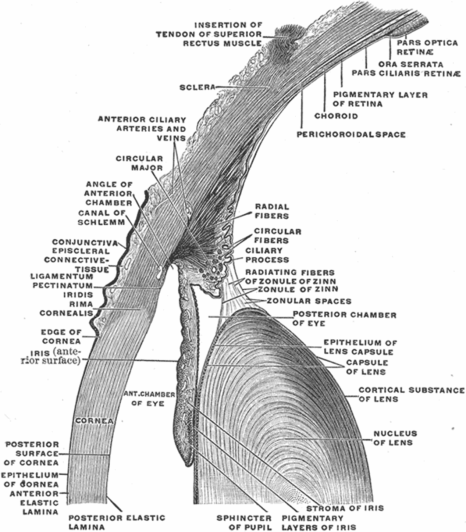
Anterior chamber
Anterior chamber depth and anterior chamber angle (Figure 3.1B) can be assessed with OCT. These measures often complement findings that are observed clinically during gonioscopy. Since the overlying sclera can be highly optically backscattering, detailed features of the anterior chamber are sometimes difficult to resolve.
The anterior angle (Figure 3.1B) can also be evaluated with OCT. The corneal epithelium (Figure 3.1C) continues laterally until it meets with the sclera at the corneoscleral limbus (Figure 3.1D), just anterior to the anterior angle. Schwalbe’s ring is a ring of collagenous fibers found on the posterior corneal surface at the termination of Descemet’s membrane (the basal lamina of the corneal endothelium).
The canal of Schlemm is found lateral to Schwalbe’s ring in the depth of the anterior angle and is the site of aqueous drainage from the anterior chamber (Figure 3.1B). Aqueous outflow proceeds through the trabecular meshwork and into the canal of Schlemm, where aqueous humor enters the aqueous veins. Aqueous veins are distinguished from blood-filled veins by the lack of optical shadows cast on deeper structures. From aqueous veins, the aqueous humor enters the scleral veins, sometimes by way of an intervening vascular plexus. Schlemm’s canal and the aqueous outflow pathways that lead to the scleral veins can be visualized with commercially available SD-OCT. 3-D OCT imaging allows for more detailed visualization of the outflow channel networks [2].
Sclera
The sclera is the dense, white protective covering of the eye, composed almost entirely of collagen (Figures 3.1D and 3.3). Its density and composition make it highly optically backscattering on OCT.
Iris
The iris divides the anterior from the posterior chamber (Figures 3.1A–B, 3.2, and 3.3). It is composed of sphincter and dilator muscles that control the size of the pupil and the amount of light entering the eye [3]. The iris is configured as a shallow cone that points anteriorly and is highly pigmented. Because the iris shadows deeper structures on OCT, images of the posterior parts of the eye must be obtained by focusing the OCT beam through the pupillary aperture.
Cornea
The corneal epithelium, Bowman’s layer, and the corneal lamellae can be imaged using ultrahigh resolution OCT. Corneal adapter modules focus the OCT beam on the cornea, while post-processing algorithms correct for refraction of the OCT beam at the anterior corneal surface. The corneal epithelium is characterized by a low scattering layer on OCT (Figure 3.1C). The striated pattern seen in the corneal stroma is attributed to its collagen structure and organization [4]. Echo reflection artifacts appear in a plane through the center of the cornea, with a strong reflection present where the OCT beam is orthogonal to the air–cornea interface, and is reflected back into the instrument.
Lens
The lens is a normally transparent and avascular structure that focuses light on the retina with the help of its biconvex configuration (Figure 3.2). It is about 4 mm thick and 9 mm in diameter. Zonule fibers suspend the lens from the ciliary body, just behind the iris. Aqueous humor is present anterior to the lens, while vitreous humor is present posteriorly [3].
Posterior eye
Choroid and choriocapillaris
The choriocapillaris is a network of fenestrated choroidal capillaries located between the sclera and Bruch’s membrane that provides nourishment to the nearby retinal pigment epithelium (RPE) and photoreceptors. In first-generation OCT images, a single layer described as the RPE-choriocapillaris complex was visible, and visualization of the individual layers of the outer retina beyond the photoreceptor inner segments was not possible. With improvements in axial image resolution, discernment of the components of the RPE-choriocapillaris complex became possible.
Ultrahigh resolution OCT used for research purposes provides more detailed imaging of the choriocapillaris and choroid than standard commercially available OCT. While a standard ~800 nm light source can distinguish the major layers of the retina, the penetration of this wavelength to the choroid and choriocapillaris is limited by the optical scattering and absorbing properties of the RPE. The choriocapillaris is best visualized with ~1050 nm light, which undergoes less scattering and absorption by melanin and can provide additional diagnostic information despite a lower axial resolution [5]. The choriocapillaris appears on OCT as a highly backscattering layer with a mean thickness of 24 μm, with optical properties consistent with those of its blood-filled capillaries (Figure 3.4) [6].
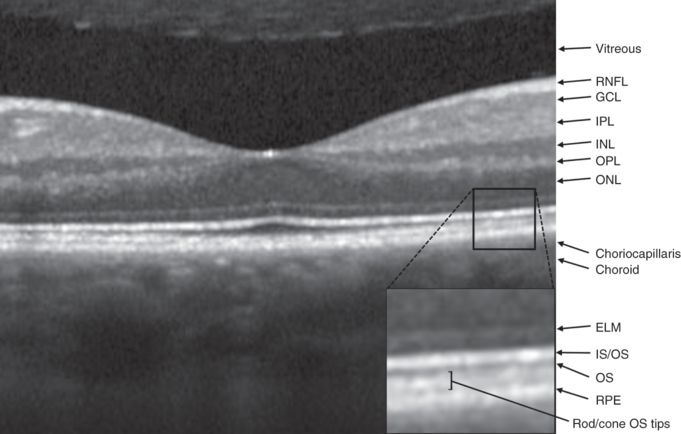
OCT of the fovea and identification of retinal layers. RNFL: Retinal nerve fiber layer; GCL: ganglion cell layer; OPL: outer plexiform layer; INL: inner nuclear layer; IPL: inner plexiform layer; ONL: outer nuclear layer; ELM: external limiting membrane; OS: photoreceptor outer segment; IS/OS: photoreceptor inner segment/outer segment junction; RPE: retinal pigment epithelium.
Bruch’s membrane
Bruch’s membrane (BM) is positioned between the choriocapillaris and the RPE. It regulates the reciprocal exchange of nutrients and waste between the retina and general circulation. It normally increases in thickness with age [7]. According to some investigators, BM measures approximately 5 μm in thickness by OCT [8]. Others maintain that there is uncertainty about the ability of OCT technology to resolve BM because its reported thickness is 1–5 μm and the axial resolution of ultrahigh resolution OCT is currently limited to 2–3 μm [9].
Retinal pigment epithelium (RPE)
The retinal pigment epithelium lies just below the photoreceptor layer in the retina, where its function is to provide metabolic and structural support for the photoreceptors. The RPE-choriocapillaris layer is present throughout the retina and terminates at the optic disc margin, where the choroidal circulation also terminates, at the lamina cribrosa (Figure 3.5). Light that is not absorbed by the photoreceptor layer is absorbed by the RPE, which contains the pigment melanin and prevents backscattered light from interfering with high-acuity vision in humans. The mean RPE layer thickness is 9 μm [6].
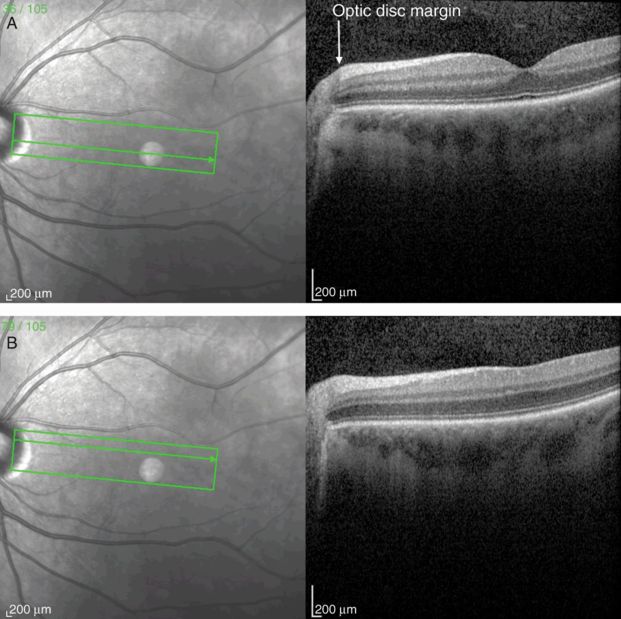
Papillomacular bundle OCT scan from the optic disc through the fovea (A) and perifoveal region (B). Note the increasing thickness of the retinal nerve fiber layer (RNFL) approaching the optic disc.
On first-generation OCT, the RPE and choriocapillaris were seen as indistinguishable components of a highly reflective layer (HRL) because these structures have similar optical properties and are in close proximity [10]. Later generations of OCT, however, demonstrated that the outer HRL has several components. The RPE may have a multilayer appearance on OCT due to differences in the length of cone and rod photoreceptor tips (Figure 3.4). Rods are longer and interdigitate with the RPE in the peripheral retina. Variation in the cone and rod distribution in the retina also influences the RPE’s layered appearance [8]. The highly reflective nature of the RPE allows comparatively little light to pass through to the layers below. Below the choriocapillaris, the deep choroid and sclera are visible as weakly reflective layers.
Retina
Once light traverses the eye, it is focused on the neurosensory retina. The retina is traditionally divided into ten layers, including four cellular layers and two layers of neuronal interconnections, many of which can be delineated by OCT (Figure 3.4) [6]. To arrive at the photoreceptors, light must first pass through the retinal nerve fiber layer (RNFL), ganglion cell layer (GCL), inner plexiform layer (IPL), the amacrine, bipolar, and horizontal cells of the inner nuclear layer (INL), and the neuropil of the outer plexiform layer (OPL) (Figure 3.6). The photoreceptors convert the electromagnetic energy of light into an electrochemical signal. Through a series of cellular connections, the signal is modified and relayed to ganglion cells, whose axons compose the retinal nerve fiber layer (RNFL) and serve as the final common output pathway of the retina.
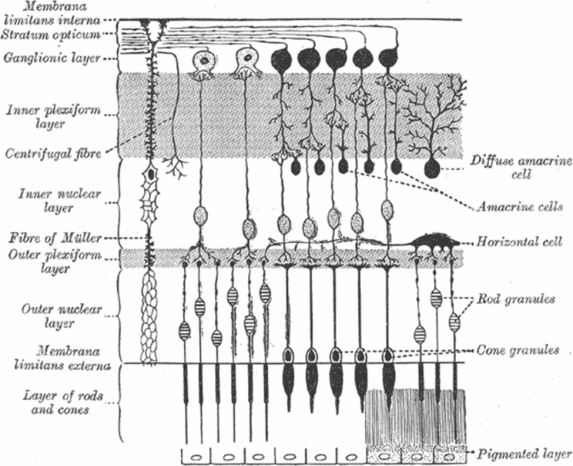
Schematic representation of retinal layers and their cellular components. The anterior retina, where light first reaches the retina, is at the top of the figure. Light passes through each of the layers before reaching the outer segments of the rod and cone photoreceptors, where the phototransduction cascade is initiated.
The axial resolution of many commercially available OCT instruments is 4–7 μm. Generally, layers containing primarily cell bodies are weakly backscattering on OCT (dark), whereas layers containing primarily neuropil are highly backscattering (bright). In animal preparations, studies have demonstrated that many of the layers viewed on ultrahigh resolution OCT can be reliably correlated with histology [11]. The layers of the inner retina seen on OCT, extending from the vitreoretinal interface to the junction of the inner and outer photoreceptor segments (IS/OS junction), correlate well with histologic preparations of monkey retina [11].
Controversy persists, however, regarding the precise correlation between layers of the outer retina visualized on OCT (including the RPE, Bruch’s membrane, and the choriocapillaris) and those demonstrated by histology [9]. For example, the strongest continuous backscattering signal in the outer retina on ultrahigh resolution OCT images has been interpreted as the RPE. While the optical properties of this backscattering layer are consistent with the RPE, some studies have reported that it appears thicker on OCT (up to 20–30 μm) [9] than on histologic sections (~9–12 ± 2 μm) [12]. On the other hand, other investigators report less of a discrepancy, with an average RPE thickness on OCT of 9 μm [6]. In the interpretation of retinal OCT images, it is important to recognize that OCT relies on differences in the optical properties of different retinal layers, whereas histology relies on postmortem tissue staining and light microscopy. While OCT provides extremely high spatial resolution, the correlation between these images and histology is likely to be imperfect.
Macula and fovea
The macula is located temporal to the optic nerve and measures approximately 5.5 mm in diameter. The macula is further divided into the fovea (1.5 mm in diameter) and foveola (0.35 mm). The fovea has up to 200,000 cones/mm2 (nearly 15-fold higher than in peripheral retina) so that it can provide excellent visual acuity [13]. Furthermore, high-acuity vision is subserved by the unique synaptic configurations in the fovea, where each bipolar cell receives input from a single cone photoreceptor and each cone relays information to two ganglion cells [14]. The fovea contains an avascular center (0.4–0.6 mm in diameter) where photoreceptors can be densely packed without making provision for intervening capillaries. The foveal depression reaches its maximum depth at the center of the macula. Here, the inner layers of the retina, including the RNFL, GCL, OPL, INL, and IPL, taper and nearly disappear to allow light to directly stimulate the underlying cone photoreceptors without distortion. The ONL and photoreceptor outer segment layers become thicker in the foveal pit, reflecting this region’s specialization for high spatial acuity. The fovea is readily identified using OCT and characterized by thinning of the anterior retina and dense aggregation of photoreceptors (Figure 3.4).
A map illustrating macular thickness in segments can be generated from a series of scans centered on the fovea. The thickness measurements are typically displayed in a segmented circular graph, with each segment colored to illustrate the relative thickness (Figure 3.10A and C).
Photoreceptors
After photons pass through the inner layers of the retina, including the GCL and INL and ONL layers, they reach the photoreceptors in the outer retina. There, they initiate a photochemical cascade that results in a change in the photoreceptor’s firing rate. Humans possess four photoreceptor types: three cones and a rod. Each type of cone photoreceptor has a unique, optimal response to specific wavelengths of light, either short (blue), middle (green), or long (red). Rods, on the other hand, are saturated at natural light intensities and are incapable of discriminating colors; their higher sensitivity to light renders them effective for night vision (scotopic vision). The retinal photoreceptors are each made up of an outer segment, inner segment, nucleus, and synaptic ending. The membrane of the outer segment has a stacked-disc configuration that houses visual pigments, such as rhodopsin. The inner segment contains mitochondria and ribosomes, where opsin molecules are assembled to be relayed to the outer segment [15].
Ultrahigh resolution OCT has been able to characterize outer retinal morphology with impressive detail, even to the level of visualizing rod and cone outer segment tips [8]. A layer of minimal reflectivity (dark on OCT) just anterior to the RPE and choriocapillaris likely represents the outer segments (OS) of the retinal photoreceptors. This layer is thickest in the fovea, where there is an abundance of cone photoreceptors. Outside the fovea, the OS layer is less defined, because rods and cones with different outer segment lengths are mixed and give rise to a faintly layered appearance between the RPE and the inner segment/outer segment interface (IS/OS). The boundary between the inner and outer segments (IS/OS) is visible on OCT as a highly backscattering layer (bright on OCT) just anterior to the RPE (Figure 3.4). The backscattering nature of this layer suggests there is a difference in the refractive index of the outer and inner photoreceptor segments [8].
The distribution of cones and rods across the retina is highly skewed and directly reflects the specialized functions of the fovea and retinal periphery [16]. OCT demonstrates that the thickness of the photoreceptor layer is increased in the fovea, where there is a high concentration of cones with long outer segments to support high visual acuity. Photoreceptor inner and outer segment length both decrease with increasing distance from the foveola. The retinal periphery has lower concentrations of cones and is associated with lower visual acuity [8]. The tips of cone outer segments extend to the surface of the RPE in the foveola, but separate from the RPE with increasing distance from the foveal center.
Rods are virtually absent in the fovea; they are the dominant photoreceptor in the periphery, where they specialize in high sensitivity motion and light detection. Rod outer segment tips are longer than those of cones, extend to the RPE surface, and interdigitate with the RPE in the peripheral retina. The difference in outer segment length between rods and cones is demonstrated by bands visible on OCT just posterior to the IS/OS junction band, which correspond to the cone and rod outer segment tips (Figure 3.4). The distance between the IS/OS junction band and outer segment tips bands is believed to approximate the outer segment length [8].
Nuclear and plexiform layers
The neurosensory retina consists of three major layers: photoreceptors synapse with bipolar cells, which then relay their signal to ganglion cells. In addition, horizontal and amacrine cells form lateral connections between elements of these layers via direct excitatory or indirect inhibitory connections (Figure 3.6) [17]. While each bipolar cell receives input from a single photoreceptor in the fovea, bipolar cells in the retinal periphery summate the inputs from multiple photoreceptor cells to support more sensitive detection of light.
The nuclear layers of the retina exhibit an intermediate degree of backscattering of light and appear as varying shades of gray in grayscale OCT and as blue-black in false-color images. The three nuclear layers that can be discerned include the outer nuclear layer (ONL), inner nuclear layer (INL), and ganglion cell layer (GCL). The inner and outer plexiform layers, which contain mostly neuropil and dense synaptic connections, demonstrate greater optical backscattering.
The ONL is composed of cell bodies of rods and cones (Figure 3.5) and shares the optical properties of the other nuclear layers. The ONL increases in thickness at the fovea, despite reduced total retinal thickness. The external limiting membrane (ELM) is visualized with OCT as a thin, backscattering layer posterior to the ONL. It is not a physical membrane but probably represents a collection of junctions between photoreceptor cells and the supportive Müller cell processes. The membrane is situated between the photoreceptor cell body and the inner/outer segments of the photoreceptor.
The outer plexiform layer (OPL), also known as Henle’s fiber layer, is somewhat thinner than the inner plexiform layer (IPL) and contains horizontal cell dendrites, bipolar cell dendrites, and photoreceptor axons (Figure 3.6). The synapses here represent the first integration of visual information in the retina. The OPL backscatters light to a moderate degree, resulting in a lighter gray layer on grayscale OCT and a mostly green layer in false-color images.
The inner nuclear layer (INL) is composed of the cell bodies of bipolar cells, amacrine cells, and horizontal cells (Figure 3.6). This layer is weakly backscattering, giving rise to a dark gray layer on grayscale OCT and a blue-black layer on false-color tomograms.
The inner plexiform layer (IPL) is found between the GCL and INL and contains neuropil made of ganglion cell dendrites, bipolar cell axons, and amacrine cell axons (Figure 3.6). The IPL is moderately backscattering on OCT images, producing a light gray layer on grayscale OCT and a blue-green layer on false-color images. The abundance of synapses in the IPL provides one of the last exchanges of visual information before being transmitted by the retinal ganglion cell axons to the lateral geniculate nuclei of the thalamus.
Ganglion cells serve as the final output pathway of visual information from the retina. There is a large convergence of information from the retinal photoreceptor cells, which include about 100 million rods and 4 million cones, to the approximately 1.2 million ganglion cells [14]. The majority of ganglion cell axons project to the lateral geniculate nucleus. There are three main types of ganglion cells, each with specialized functions in the detection of visual inputs. Anatomical differences underlie important physiological and functional differences in these types of cells. Eighty percent of ganglion cells are midget cells, 10% are parasol cells, and 10% are other types [15]. The fovea has a high concentration of midget ganglion cells. Via a narrow dendritic tree, these cells receive signals conveyed by a bipolar cell that has received inputs from a single cone. The GCL is made of ganglion cell bodies that lie just posterior to the retinal nerve fiber layer (RNFL). Like other nuclear layers in the retina, the GCL is weakly backscattering on OCT, giving rise to a dark gray layer on grayscale OCT and blue-black layer on false-color images. The GCL is thickest in the parafoveal region because of the high density of photoreceptors in the fovea, but decreases in thickness toward the optic disc (Figure 3.5A).
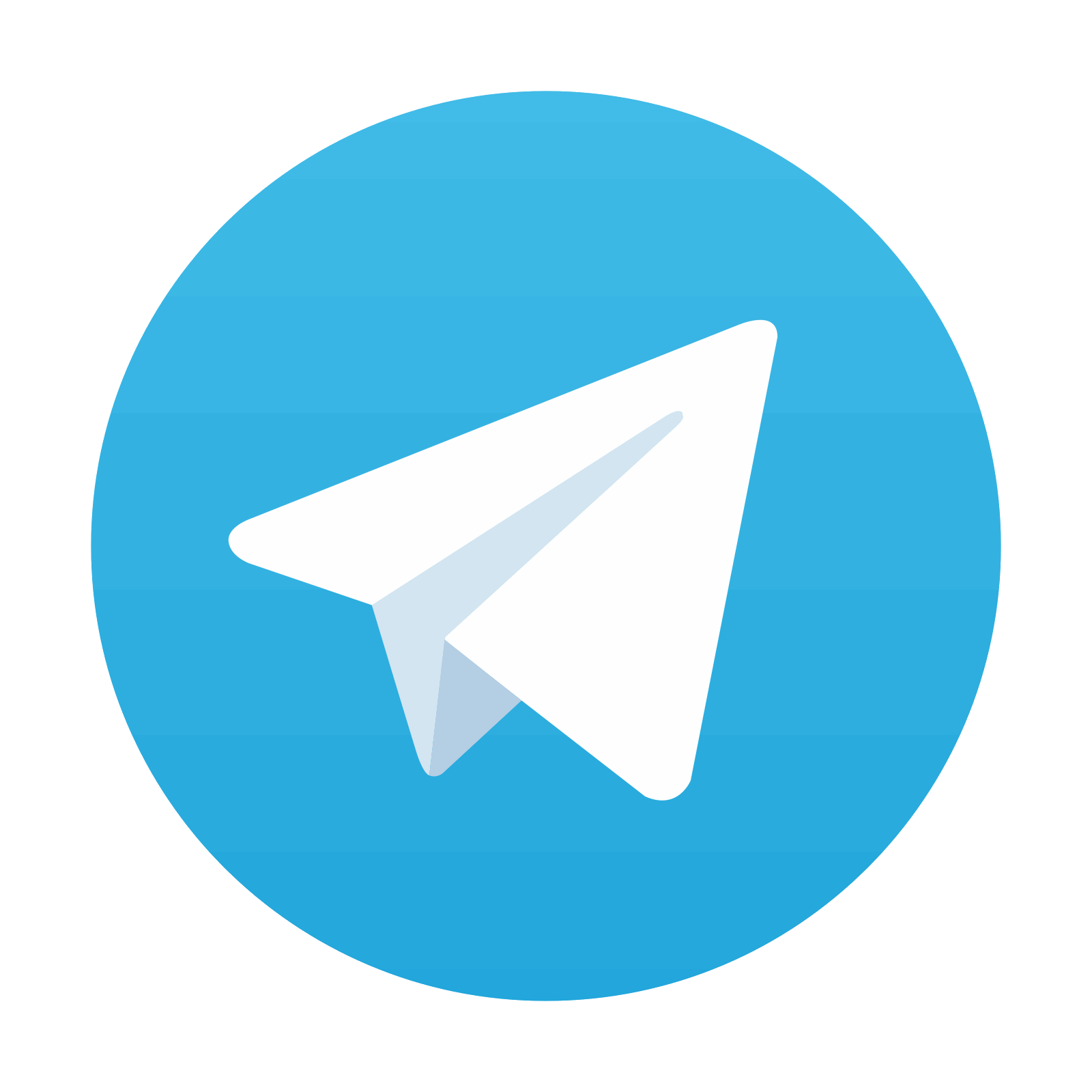
Stay updated, free articles. Join our Telegram channel

Full access? Get Clinical Tree
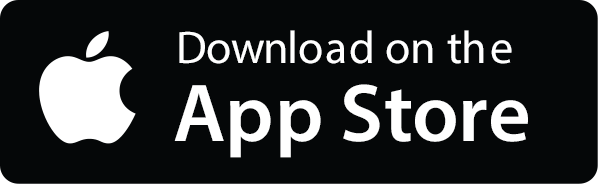
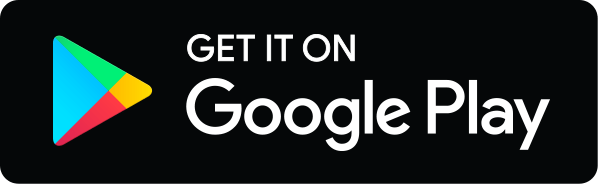